- What Is The Economic Importance Of Algae?
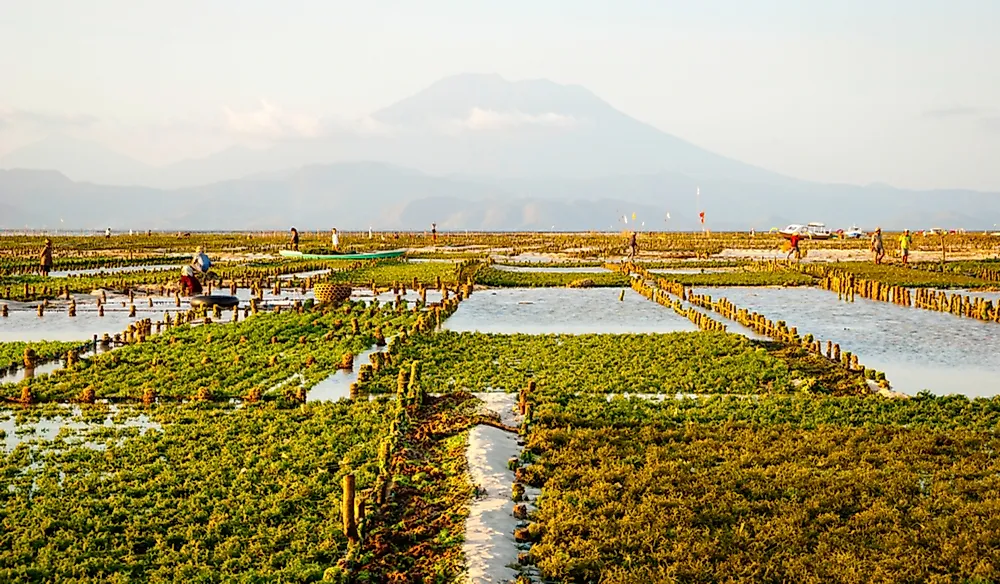
Algae refers to a wide range of eukaryotic marine organisms, all of which engage in the process of photosynthesis. Ranging in size from unicellular microalgae to giant kelp, these aquatic plants are characterized by their lack of flowers, formal roots, leaves, or even stems. Algae are economically important in a variety of ways. The natural substance can be used as a food source, a fodder, in fish farming, and as a fertilizer. It also plays a key role in alkaline reclaiming, can be used as a soil binding agent, and is used in a variety of commercial products.

Uses for Algae
Numerous cultures around the world consider algae an important source of nourishment. Citizens living in European countries such as Ireland , Scotland , France , Germany , Norway , and Sweden , as well as populations living in North and South America, and Asian nations such as China and Japan use algae as a key ingredient in a number of local dishes. These meals can include algae as part of a salad, accompanying meat in a fried dish, as a topping on oatmeal, or even in liquid form as an extract in a nutritious smoothie.
Algae contains several healthy elements including carbohydrates, fats, proteins, and vitamins A, B, C, and E. Not only is algae considered by many consumers worldwide to be a low cost source of protein, but it also contains a number of important minerals such as iron, potassium, magnesium, calcium, manganese, and zinc. Foods which commonly contain algae include a variety of dairy products such as milk, ice cream, cheese, whipped topping, as well as syrup, icing, fruit juice, and even salad dressings. Brown algae, in particular, is used in order to stabilize, thicken, and emulsify numerous food products, while red algae is used in the preparation of various semi-solid products as wide-ranging as medicines, cosmetics, and in the production of a wide array of foods.
Algae, especially seaweed, is used as a feed for a variety of farm animals. For example, Rhodymenia palmate, or so-called "Sheep’s weed," is used in order to feed livestock such as cattle and chickens. Algae is used as fodder in various countries, such as the northern European nations of Sweden, Denmark , and Norway, as well as in Scotland, China, New Zealand , and throughout North and South America.
Pisciculture
The industry involved in the breeding and farming of fish, also known as fish farming or pisciculture, also utilizes algae as part of its production process. According to scientists, numerous species of fish like to consume a variety of types of algae, the most common sources being blue-green and green algae, as well as with microalgae. Fish usually feed on floating plankton and zooplankton, which provides a source of healthy vitamins. Algae is also used in pisciculture as a way in which to naturally absorb carbon dioxide from the environment, while at the same time providing oxygen to the water, thus making the marine environment more habitable for fish.
The two most common varieties of algae used in the manufacture of fertilizer are large red and brown. In particular, these two types of algae are utilized in areas located near the ocean. Liquid fertilizer can also be produced using a concentrated seaweed extract. The reasons why this type of fertilizer is so popular involves the organism’s ability to repair levels of nitrogen already present in the soil. For example, rice producers in India typically employ blue-green algae in order to fertilize their agricultural fields.
Reclaiming Alkaline
In many countries, such as India, fields that once produced large agricultural yields can no longer be used due to high concentrations of alkalinity in the soil. In order for crops to eventually be grown in these lands, often referred to as "Usar" lands, the ph level must be lowered and the ability of the soil to hold onto water must be increased. This process can be achieved using blue-green algae.
Binding Agent
Algae can also be used to help bind soil together. The use of algae to aid in the healthy formation of soil is important in the protection against natural processes such as erosion.
Algae Products
Algae is used as a key ingredient in various food products. The substance can either be used as an additive or can act as a thickener in many types of food products. Algae can also be found in household products, such as toothpaste and various pharmaceuticals. A type of algae, known as carrageenan, is often added to dairy products such as cheese and sour cream in order to give them a denser texture. Alginic acid, which can be found in algae, is used to stabilize foods such as milkshakes, malts, and mayonnaise. Consumers might also be familiar with the green paper-like sheets used to wrap sushi. This product, called nori, is actually a leafy form of algae which was traditionally grown in Japan and contains plenty of healthy nutrients and assorted vitamins.
Gelatin is used in the production of numerous edible products. In fact, this substance is made up of a type of algae known as agar. Purportedly first used in 17 th century China, gelatin acts to solidify liquids found in such popular products such as pie crusts and fillings.
Purple-colored algae, also known as Porphyra, is commonly used in Japan and is known as nori, which is used to wrap up sushi rolls. In Korea, this same substance is known as gim. In Wales , this type of algae is referred to as laverbread, which is traditionally served for breakfast along with bacon and cockles (a type of mollusk). In Ireland, residents stew or boil the algae, thus making it into a pink colored jelly.
In the manufacturing of toothpaste, algae is used in order to thicken what would otherwise be a runny substance and transform it into a partially solid form. This particular form of algae is safe to consume and dissolves during the tooth brushing process.
- Environment
More in Environment
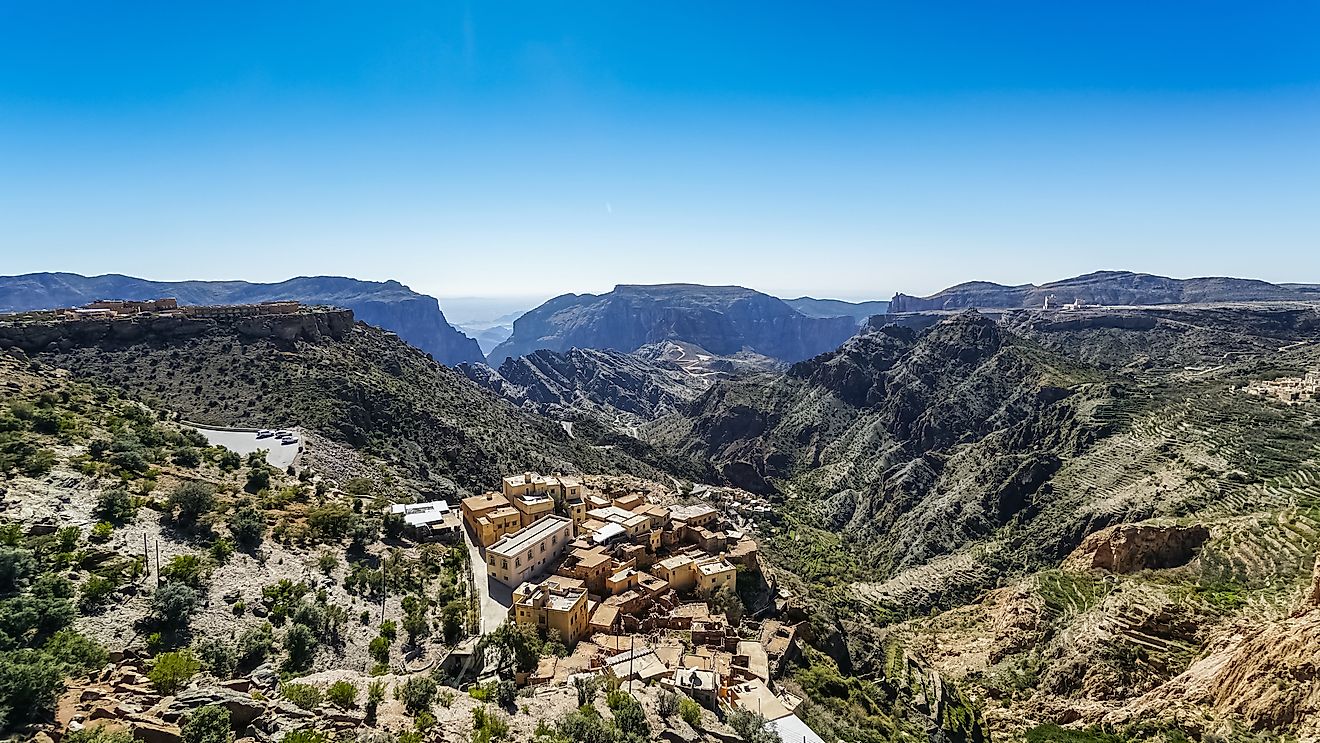
Importance Of Wetlands
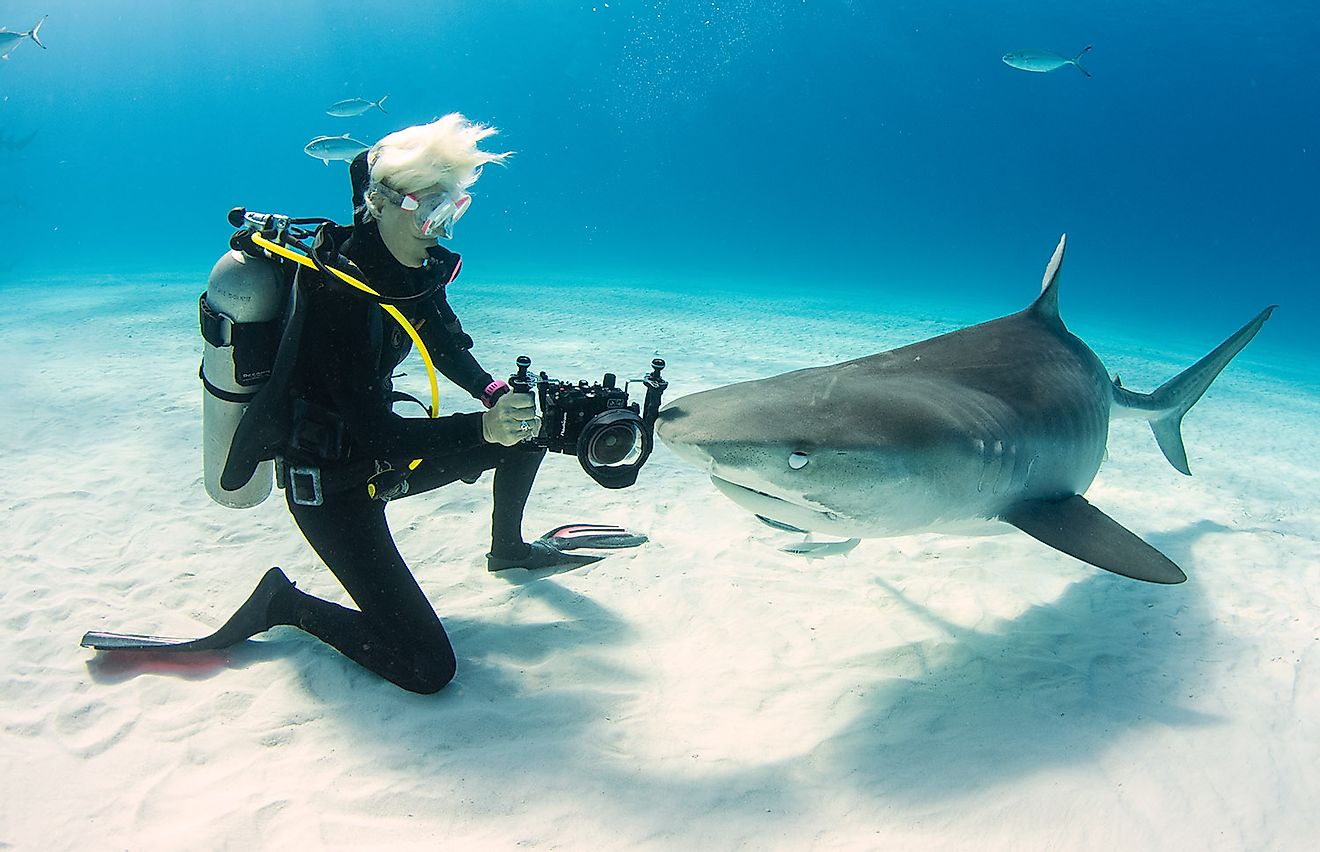
Meet 12 Incredible Conservation Heroes Saving Our Wildlife From Extinction
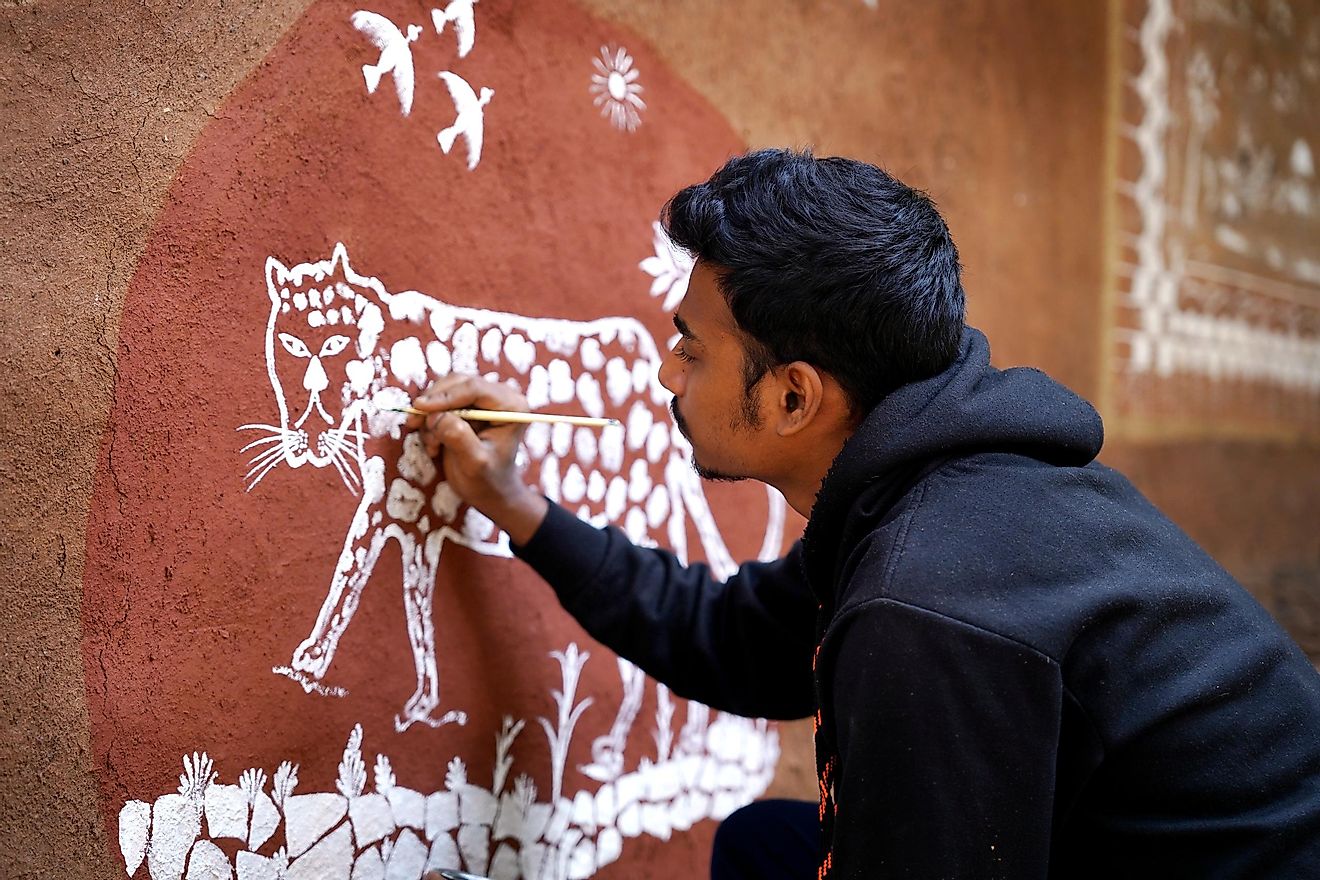
India's Leopard God, Waghoba, Aids Wildlife Conservation In The Country
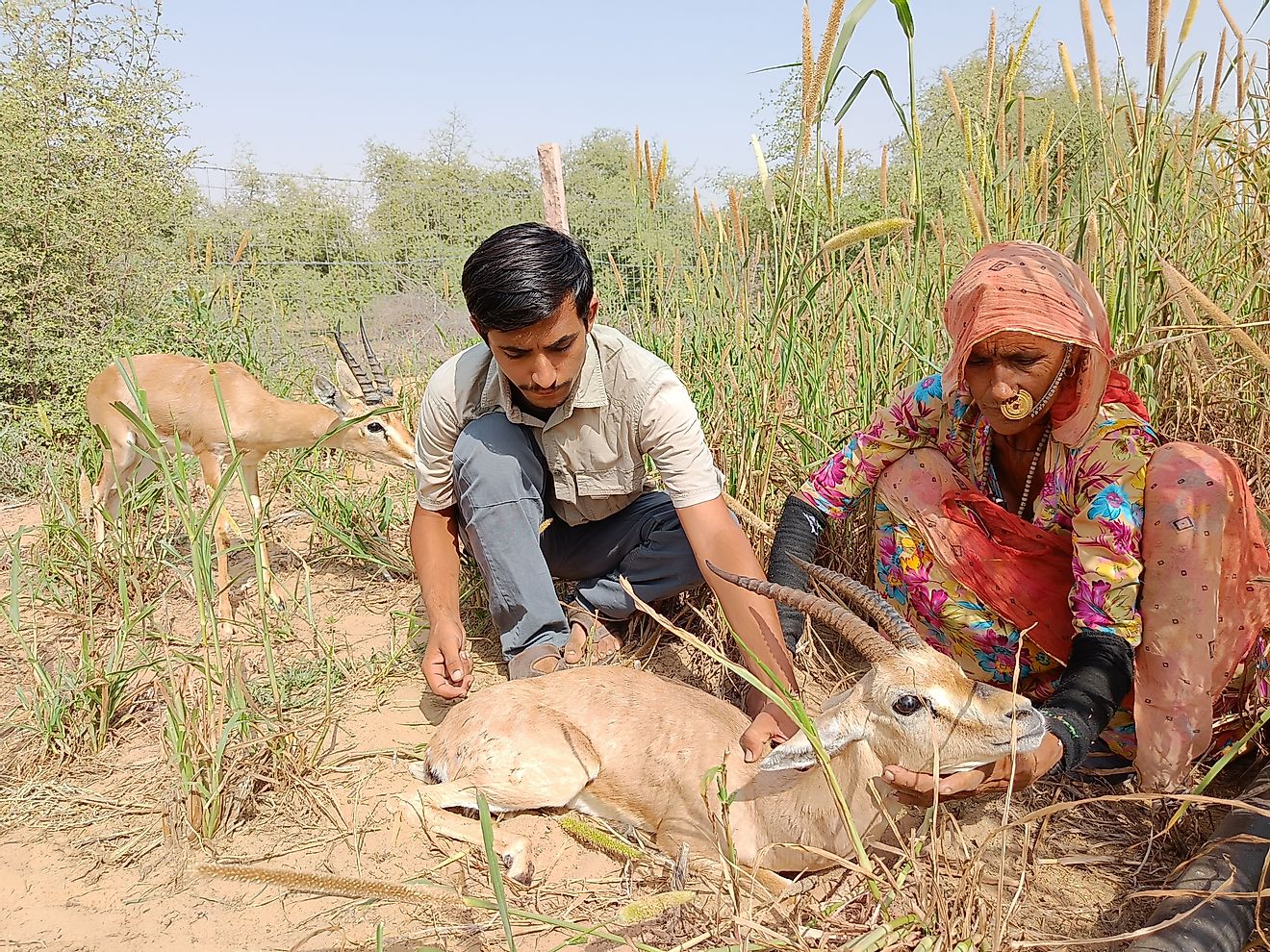
India's Bishnoi Community Has Fearlessly Protected Nature For Over 500 Years
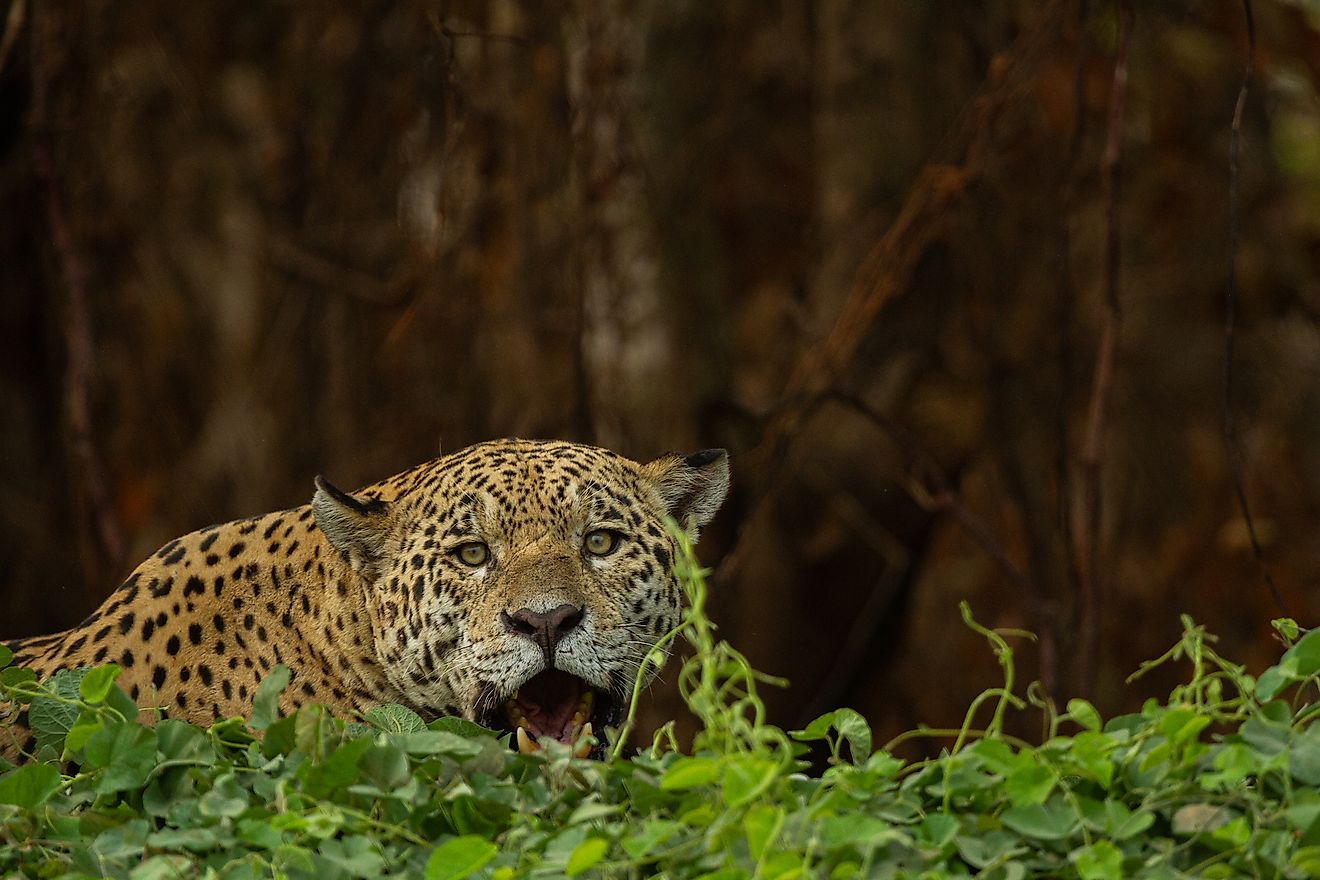
Wildfires And Habitat Loss Are Killing Jaguars In The Amazon Rainforest
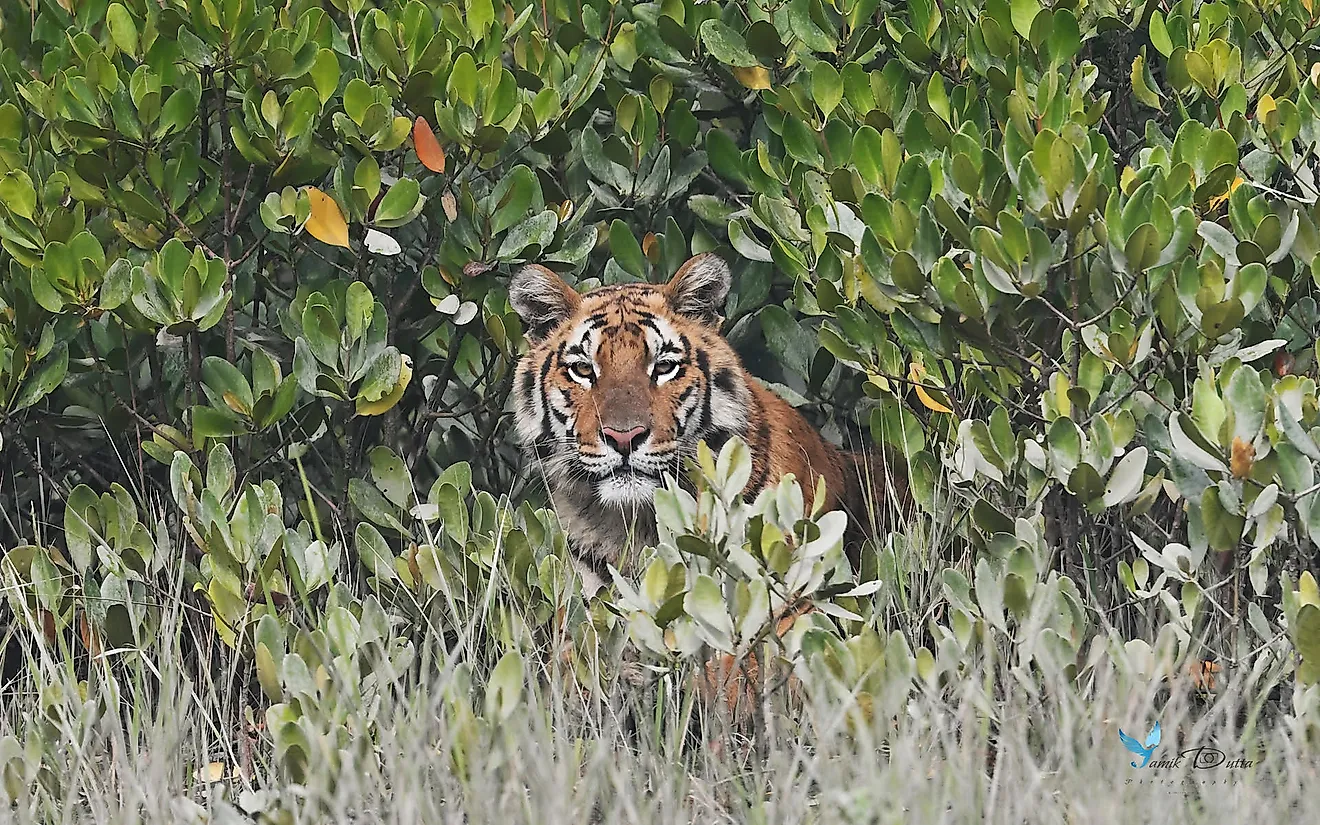
In India's Sundarbans: Where People Live Face-To-Face With Wild Tigers
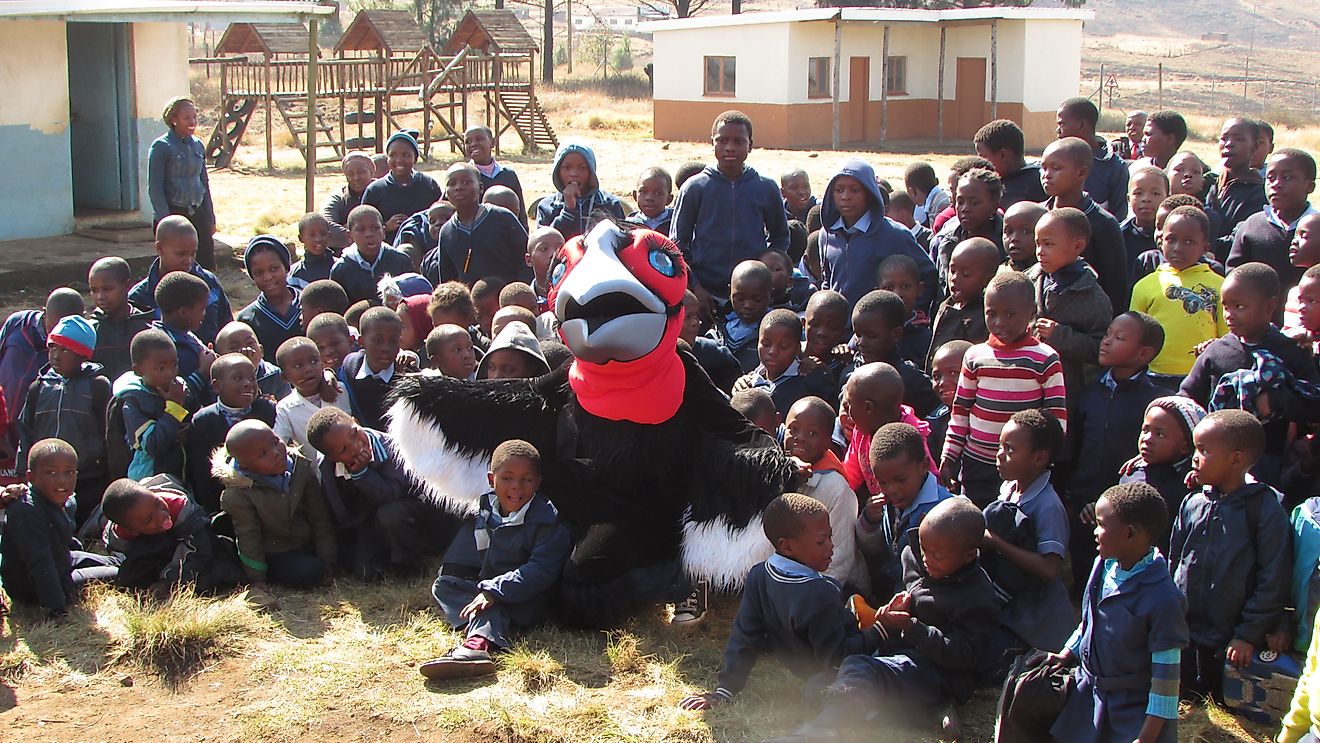
Africa's "Thunderbird" Is At Risk Of Extinction
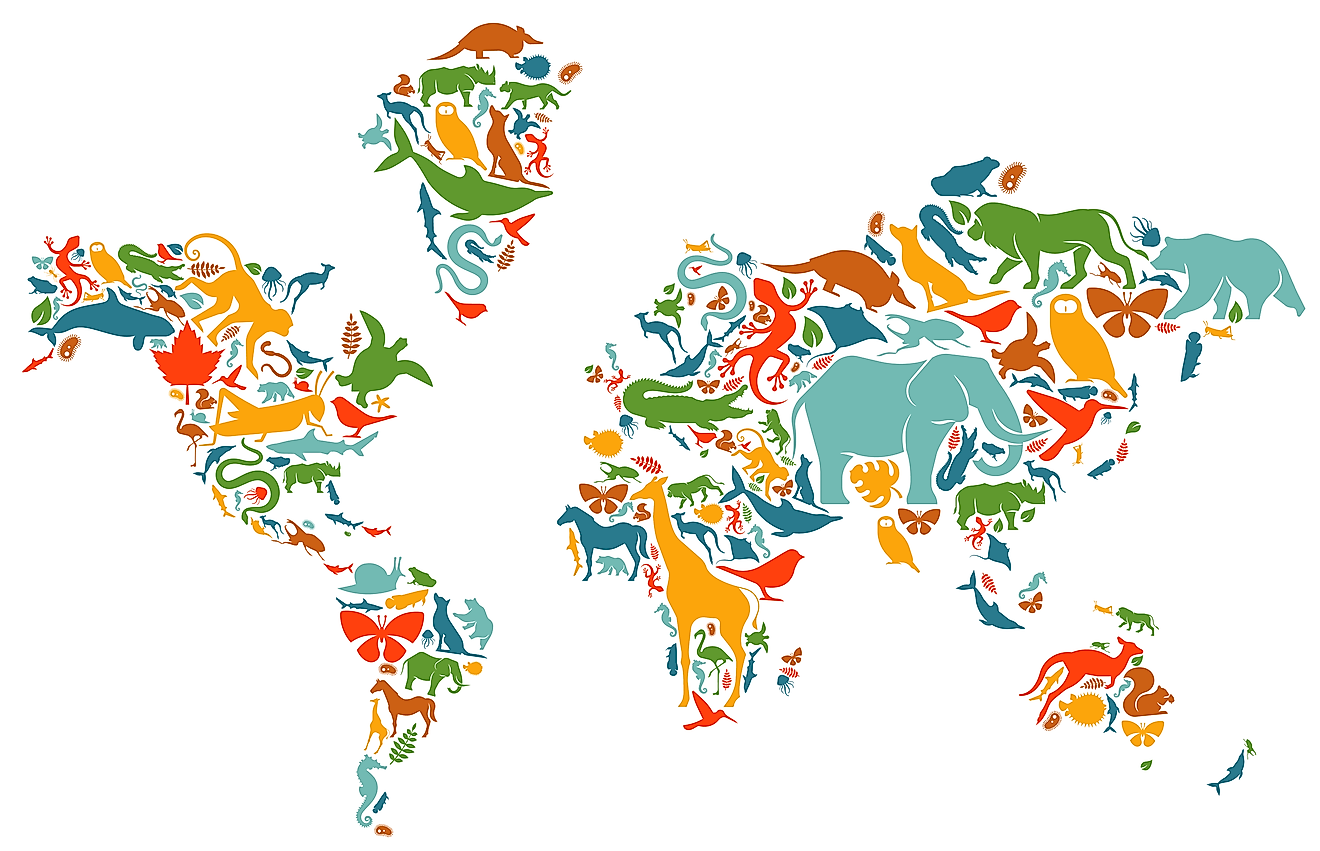
Why Is Biodiversity Critical To Life On Earth?

Economic Uses
- Kombu, nori and wakame (Japan) Kombu = Laminaria Nori = Porphyra Wakame = Undaria stipes and blades
- Hai dai - (China) Laminaria
- Limu (Hawaii) - [Miscellaneous algal species] Limu kohu = Asparagopsis taxiformis Limu wawaeiole = Codium Limu huluhuluwaena = Grateloupia filicina Limu palahalaha = Ulva
- Dulse (Scotland); Dillisk - (Ireland); Sol - (Iceland) Rhodymenia palmata
- Irish moss or Carraghean (Europe) Chondrus crispus
- Nori or Amanori (Japan); Zicai (China) Porphyra
- Abbott, Isabella A. 1984. An Ethnobotanical Study of Some Hawaiian Seaweeds . Pacific Tropical Botanical Garden. 35 pgs.
- Fortner, Heather J. 1978. The Limu Eater - A cookbook of Hawaiian seaweed . Sea Grant Miscellaneous Report UNIHI- Seagrant-MR-79-01 107 pgs.
- Madlener, Judith Cooper 1977. The Seavegetable Book -- Foraging and Cooking Seaweed . Clarkson N. Potter, Inc. 288 pgs.
- Major, Alan. 1977. The Book of Seaweed . Gordon & Cremonesi Publ. 234 pgs.
Brown algae (Phaeophyta) includes Kelps ( Macrocystis , Laminaria ) and Ascophyllum , Fucus , and Sargassum . Alginic Acid (Alginate) is a colloidal product used for thickening, suspending, stabilizing, emulsifying, gel-forming, or film-forming, as required.
- About half of the alginate produced is used for making ice cream and other dairy products, the rest is used in other products, including shaving cream, rubber, or paint.
- In textiles, alginates are used to thicken fiber-reactive dye pastes, which facilitates sharpeness in printed lines and conserves dyes.
- Dentists use alginates to make dental impressions of teeth.
Applications using Alginates
FOOD (Nondairy): Frozen foods, Pastry fillings, Syrups, Bakery icings, Relishes, Cooked/ instant puddings, Meringues, Chiffons, Dessert gels, Candies, Fruit juices, Jams & Jellies, Sauces and gravies, Pimiento strips, Salad dressings FOOD (Dairy) :Whipped toppings, Milk shakes, Cheeses, Flans and custards, Instant breakfasts, Ice Cream INDUSTRIAL :Paper sizing / coatings, Adhesives, Textile printing / dyeing Air freshener gels, Explosives, Boiler compounds, Polishes Antifoaming agents, Ceramics, Welding rods, Cleaners, Castings and impressions, Enzyme immobilization MEDICAL & PHARMACEUTICAL :Baulking agents, Capsules and tablets, Lotions and creams, Ulcer products
Red algae (Rhodophyta) Carrageenan is made from Gigartina stellata , Chondrus crispus and Eucheuma . Carrageenan (carrageenin, carragheen) is similar to agar, but requires higher concentrations to form gels.
- Carrageenan is used for stabilizing chocolate, milk, egg nog, ice cream, sherbets, instant puddings, frostings, creamed soups, etc.
Red algae (Rhodophyta) Agar is made from Gelidium , Gracilaria , Pterocladia and Ahnfeltia . Agar is another colloidal agent used for thickening, suspending, and stabilizing. However, it is best noted for its unique ability to form thermally reversible gels at low temperatures.
- The greatest use of agar is in association with food preparation and in the pharmaceutical industry (as a laxative, or as an inert carrier for drug products where slow release of the drug is required).
- Agar is used in bacteriology and mycology as a stiffening agent in growth media.
- Agar is used as a stabilizer for emulsions, and as a constituent of cosmetic skin preparations, ointments, and lotions. It is used in photographic film, shoe polish, dental impression molds, shaving soaps, hand lotions, and in the tanning industry.
- In food, agar is used as a substitute for gelatin, as an antidrying agent in breads and pastry, and also for gelling and thickening purposes. It is used in the manufacture of processed cheese, mayonnaise, puddings, creams, and jellies and in the manufacture of frozen dairy products.
Applications using Red Algae Carrageenan
FOOD (Nondairy) :Frozen foods, Dessert gels, Pastry fillings Fruit juices, Syrups, Jams & Jellies, Bakery icings, Sauces and gravies, Relishes, Pimiento strips, Cooked/ instant puddings, Salad dressings, Chiffons FOOD (Dairy) :Whipped toppings, Milk shakes, Skim milk, Evaporated milk, Chocolate milk, Cheeses, Cottage cheese, Infant formulas, Flans and custards, Yogurt, Instant breakfasts, Ice cream INDUSTRIAL :Air freshener gels, Tertiary oil treatment, Cleaners, Enzyme immobilization, Electrophoretic media, Chromatographic media MEDICAL & PHARMACEUTICAL :Laxatives, Baulking agents, Capsules and tablets, Lotions and creams, Shampoos, Ulcer products, Toothpastes
FOOD (Nondairy) : Frozen foods, Dessert gels, Bakery icings, Candies, Meringues, Fruit juices FOOD (Dairy) :Cheeses, Yogurt INDUSTRIAL :Paper sizing / coatings, Microtomy media, Adhesives, Electrophoretic media, Textile printing / dyeing, Chromatographic media, Castings and impressions, Conductivity bridges MEDICAL & PHARMACEUTICAL :Laxatives, Capsules and tablets, Baulking agents, Suppositories, Radiology suspending agents, Anticoagulants
- Sewage treatment to remove inorganic nutrients and toxins Unicellular freshwater Chlorophyta and other micro- and macroalgae.
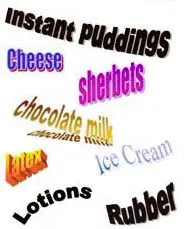
- Smithsonian Institution
- Terms of Use
- Privacy Policy
- Host an Event
Advertisement
Algae and their potential for a future bioeconomy, landless food production, and the socio-economic impact of an algae industry
- SI: LandLessFood Workshop 2019
- Open access
- Published: 21 January 2021
- Volume 11 , pages 261–267, ( 2021 )
Cite this article
You have full access to this open access article
- Jörg Ullmann 1 &
- Daniel Grimm ORCID: orcid.org/0000-0003-4873-150X 2
18k Accesses
48 Citations
11 Altmetric
Explore all metrics
Despite being a comparatively new branch of agriculture, algae production is often considered to be a solution to many food security-related problems, such as land scarcity, climate change, inefficient and unsustainable fertilizer usage, as well as associated nutrient leakage and water pollution. Algae can be cultivated independent of arable land and, especially in the case of many microalgae, produce oil- and/or protein-rich biomass with spatial efficiency which far exceeds that of terrestrial plants. Nevertheless, algae and algae-derived products are almost exclusively produced for high-value, low-volume markets and are far from being able to compete with cheap commodities such as plant-based proteins or fossil fuel. High investment and production costs are considered the main reason for this, but a lack of economic incentives for sustainable production and CO 2 mitigation should not be overlooked. The development of new production technologies; the monetization of ecosystem services, such as water treatment, CO 2 sequestration, and nutrient recycling; as well as the simultaneous production and marketing of “high-value, low-volume” and “low-value, high-volume” products from the same algal biomass are the most promising ways forward. A sustainable “algae industry” could be an integral part of the future bioeconomy, enabling more resource-efficient food and fuel production and creating new products, companies, and jobs.
Similar content being viewed by others
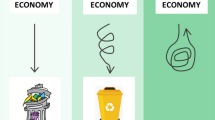
Circular economy strategies for combating climate change and other environmental issues
Mingyu Yang, Lin Chen, … Pow-Seng Yap
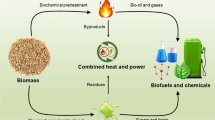
Conversion of biomass to biofuels and life cycle assessment: a review
Ahmed I. Osman, Neha Mehta, … David W. Rooney
Rice Straw Overview: Availability, Properties, and Management Practices
Avoid common mistakes on your manuscript.
Algae production: history, present, and future
Algae are a polyphyletic group of organisms from four different biological kingdoms: Bacteria, Plantae, Chromista, and Protozoa. Around 44,000 species have been scientifically described, but the real number, though dependent on which definition of “algae” is used, will be much higher, with some estimates being as high as a million different species (Guiry 2012 ). As photoautotroph organisms, algae are the starting point of most food webs in aquatic ecosystems. The biomass productivity of many algal species is much higher compared to that of terrestrial plants, and they can be efficiently cultivated without antibiotics and pesticides in fresh or sea water. These factors, as well as their high content of vitamins, polyunsaturated fatty acids, and other healthy compounds, have led to increasing consumer demand and commercial interest in algae production during the last decades.
Seaweeds have been used as food and medicine for at least 14,000 years and possibly even played an important role in the peopling of the Americas (Erlandson et al. 2007 ). First farming activities of seaweeds in East Asia date back at least to 1640 (Pulz and Gross 2004 ), though possibly much longer (Ferdouse et al. 2018 ). Consumption of microalgae on the other hand has historically been rare. The only commonly cited examples are the harvesting of naturally occurring Spirulina , a genus of cyanobacteria, by the Aztec people in the valley of Mexico and the Kanembu people around Lake Chad (Ahsan et al. 2008 ). The large-scale cultivation of microalgae and seaweeds is a very new branch of aquaculture, dating back only to the middle of the twentieth century, when the global industrial production of algae was near to “zero” tons. The first commercial cultivation of a microalgae was that of Chlorella vulgaris , which started in Japan, in the 1960s (Mobin and Alam 2017 ). In 2004, one estimate put the global production of microalgae at around 5000 tons (dry weight) and US$ 1.25 billion per year (Pulz and Gross 2004). The production is likely to have increased a lot since then; however, it is surprisingly hard to find reliable data on this. Seaweed production has also increased rapidly: the FAO estimates that the global production more than doubled, from 14.7 million tons (wet weight) in 2005 to 30.4 million tons in 2015, which accounts for a market of roughly US$ 6 billion (Ferdouse et al. 2018 ; Camia et al. 2018 ). Compared to the global agricultural production of roughly 1.6 trillion tons, this is still an extremely small amount (Buschmann et al. 2017 ).
Of the 220 species of seaweed that are commercially used, most are farmed offshore, in marine environments, while only about 1.1 million tons are harvested in the wild—a number that has been stagnant in recent years (Ferdouse et al. 2018 ). Most of the produced seaweed is either used as human food (roughly 47%) or for the production of hydrocolloids (over 50%), such as agar, carrageenan, and alginate (Buschmann et al. 2017 ). The productivity of different seaweeds in offshore farms in Japan lies between 1.3 kg/m2 for Laminaria angustata and 8.3 kg/m 2 for Sargassum macrocarpum and is thus comparable to the productivity of land crops (Notoya 2010 ). According to estimates by the World Bank (Bjerregaard et al. 2016 ), 5 million km 2 of seaweed production area—roughly 0.3% of the ocean surface—would be enough to produce as much biomass as is produced in all of global agriculture annually.
Even though microalgae are spatially more productive than terrestrial plants and macroalgae— Spirulina , for example, produces about 10 times more biomass per hectare than high-yielding corn hybrids (Dismukes et al. 2008 )—their production is still more expensive, especially due to high initial investment and production costs. For this reason, microalgae production is mainly focused on the provision of “low-volume, high-value” products, such as β-carotene, astaxanthin, docosahexaenoic acid, docosahexaenoic acid, phycobilin pigments, and algal extracts for use in cosmetics, instead of “high-volume, low-value” products, such as biofuel, food, or feed (Borowitzka 2013 ). The same goes for seaweeds, which some also view as ideal crops for biofuel production, due to high carbohydrate contents of roughly 50%. With fossil oil prices being as low as they are, there is at the moment little chance of competitiveness. One way of overcoming this problem in the case of microalgae production is either the development of a scalable phototrophic production technology or the development of a convincing biorefinery concept for the multiple use of the algal biomass. Light limitation and technological limits are the most challenging tasks. The other approach is focused on the monetarization of ecosystem services that algae can provide: the cleaning of water, the mitigation of climate change, etc. This approach, though promising, requires significant changes in the legislative set-up of the economic system and is thus highly controversial. In the following passage, we want to delve deeper into these problems and outline a way forward.
The ideal microalgae production system?
An ideal production system for algae cultivation is not focused only on the provision of one product. Instead, it takes advantage of the ecosystem services that algae can provide and extracts several products from the same biomass. This has economic as well as environmental advantages. Today three different production methods are established: open pond systems, photobioreactors, and fermenters. Each technology has its advantages and disadvantages. A decision on which one to choose should be highly dependent on the final product and/or application, the microalgae species, and the production costs. Next to the availability of water (especially for the cultivation of freshwater species) and high solar radiation, labor and energy costs are important factors to bear in mind, since these are major drivers of production costs. It could be argued that an “ideal” production system would favor marine species over freshwater species, since sea water is an unlimited resource, and phototrophic over heterotroph production, since this would bind rather than emit CO 2 . However, this would be an oversimplification. Freshwater algae can, for example, be used for wastewater processing or in areas where freshwater is abundant. Fermentation on the other hand is easily scalable, relatively cheap, and interesting for “refining” organic carbon compounds such as dextrose or acidic acid into biomass which is rich in compounds such as Omega 3 or antioxidants. Also, the CO 2 from fermentation could be used to boost productivity in photobioreactors.
In an ideal production system, the provision of CO 2 as fertilization for photobioreactors should not be a cost factor. Richardson et al. ( 2010 ) estimate a price for CO 2 of $0.0035–0.313/kg of biomass produced. If money was made rather than spent by using CO 2 as fertilizer in algae production, for example, if a CO 2 tax was introduced, this would have a significant impact. However, political and social support for improving the economic conditions for algae production through such means is still lacking (Nhat et al. 2018 ).
When it comes to photobioreactors, open pond systems are generally less expensive to build and operate. Therefore, for large-scale biomass production at low product cost, closed photobioreactors are usually considered unsuitable (Lundquist et al. 2010 ). However, closed bioreactors or fermenters are ideal for cultivation of high-quality starter cultures, which are used to inoculate open pond bioreactors. Also, when it comes to high-value products, closed photobioreactors are oftentimes ideal, because they produce more reliably and are less affected by pests than open pond systems. Closed photobioreactors also require more energy for gas exchange, to avoid oxidative stress (Kuenz et al. 2020 ). However, they can also be more efficiently used for the sequestration of CO 2 from flue gases than open systems.
To help reduce the costs of high-volume, low-value products, derived from algae, a simultaneous production of high-value products from the same biomass seems to be the most promising way forward.
Pigments, such as chlorophyll, carotenoids, or phycobiliproteins, as well as biologically active compounds used for pharmacological applications, can be extracted from algae as byproducts of oil or protein extraction. This could help to offset the high costs of cultivation and harvesting and thus make microalgae-derived biofuel (Bai et al. 2011 ) or proteins more competitive. However, this extraction method uses chemicals such as methanol and hexane, which could increase the environmental impact.
It is also possible to extract oil from microalgae via hydrothermal liquefaction (HDL). This process, in which algal biomass is heated at around 200–350 °C and under pressure of 15–20 MPa, has the advantage that it can be used on wet biomass, which safes energy (Fernandez et al. 2018 ). The byproducts of HDL are organic compounds, some of which are poisonous if released as wastewater. However, it has been shown that anaerobic digestion of these compounds enables efficient methane and thus energy production, which could further reduce the costs of this process—which is especially cost and energy efficient compared to alternatives (Nhat et al. 2018 ).
Algae and their potential in a future/circular bioeconomy
Since algae production needs not only water and carbon dioxide, but also a range of macronutrients, like nitrogen and phosphorous, it could be the ideal production system to produce biomass from certain waste streams. Considerable amounts of these nutrients are used in agriculture and end up in the sea and lakes, which often results in harmful algal blooms (Michalak et al. 2013 ). Seaweed farming in deltas, river mouths, or bay areas could not only profit from this resource but also help to reduce the environmental impact of nutrient run-off derived from agricultural activities.
Another question of increasing importance is how to recycle urban waste streams. It is estimated that 68% of the human world population will be living in cities by the year 2050 (United Nations 2018 ). A potential solution for nutrient recycling under such circumstances could be the urban/vertical farming of algae, potentially indoors, e.g., under greenhouses on rooftops, using closed or semi-closed reactor systems. There are already a few realized concepts, such as the “Algenhaus” in Hamburg, Germany (Aßmus et al. 2018 ), and a lot of theoretical concepts on how to integrate algae farms into modern architecture.
Especially in urban settings, the bioremediation of waste waters is of increasing importance, and it is thus one of the applications of microalgae which have sparked most interest. By using phototrophic algae in the bioremediation process, the energy costs and greenhouse gas emissions of wastewater treatment could be reduced, since biomass would be created, which could be processed to biogas or fuel, and because the oxygen produced by algae could potentially be used to make other processing steps, such as aerobic fermentation, more effective. Vice versa, the CO 2 , which is produced in the burning of biogas, could be fed into bioreactors, to increase algal growth. In addition to producing drinking water and binding nonrenewable nutrients, such as phosphate, and renewable nutrients, such as nitrogen, algae as part of wastewater treatments would thus reduce the environmental impact of this process. A likely downside compared to “conventional” wastewater treatment would be the need for more space. Lundquist et al. ( 2010 ) calculate that around 100 ha of open pond bioreactors would suffice to treat the wastewater of 165,000 to 235,000 people. The area for such a wastewater treatment plant/algae farm should not infringe on agricultural land or the living space of urban populations. Figure 1 shows different scenarios (on-shore, off-shore, urban settings, and aquaponics) in which algae cultivation can be practiced, which we discuss in the paragraphs below, and highlights products, as well as ecosystem services, which can be provided in these ways.
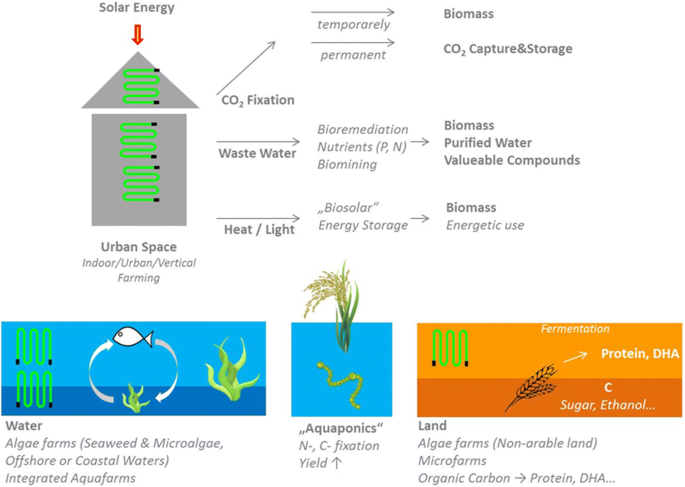
Potential of algae for a circular bioeconomy, landless food production, and urban farming
Land-based algae farms
Land-based microalgae cultivation should ideally take place on non-arable land. A lot of examples of open pond systems or photobioreactors in arid regions are available, like the Arava desert (Israel), the Atacama Desert, or the coastal desert of Morocco (SuSeWi 2020 ). Coastal deserts are excellent locations for the cultivation of marine microalgae, since no freshwater is used, while the sea water that is being used is virtually unlimited. Cultivation of freshwater algae should take place either in urban areas, ideally as part of wastewater treatment, or in humid climate zones, where freshwater is not a limited resource. In such areas, open pond “microfarms” could help to fight malnutrition locally and make farmers independent of increasing prices for arable land and fertilizers (Rahmann et al. 2020 ).
The options that take up the least space are fermenter systems, which could be used to refine lower value products, like organic C-compounds into high-value products (PUFA, pigments).
Sea-based algae farms
Classical seaweed farms are installed on the coastlines, as sole seaweed farms or as integrated multitrophic farms realizing fish/invertebrate farms in combination with seaweed production at the same place and time. This could also help capturing fertilizer run-off from intensive agricultural areas and thus prevent damage to coastal aquatic ecosystems. Another option, offshore farms of seaweed, seems to be too expensive to realize and run, though this might change in the future.
Offshore microalgae farms are part of research projects but still in a very preliminary phase. The idea is to have floating, closed bioreactors, on water surfaces. The OMEGA research program by the NASA, for example, looks into floating, more or less tubular membrane enclosures (Wiley 2013 ). Advantages of floating microalgae farms, apart from the lack of need for land, are that naturally occurring could provide mixing and the seawater could provide cooling of the photobioreactors.
Special Aquaponic systems based on blue-green algae
Nitrogen-fixing Cyanobacteria (blue-green algae) have been used for a long time as inoculum for rice fields to increase yields (Mishra and Pabbi 2004 ). Similar Cyanobacteria are the natural symbionts of some legumes and known for their N-fixing potential. These cyanobacteria could fixate nitrogen as macronutrient from the air and recover phosphorous from wastewater simultaneously, which would be an interesting application and an enhancement of the traditional use of these species.
Establishment of an algae industry and its socio-economic impact
The algae industry is no older than 70 years and currently mainly produces extracts for processed foods and other industries, such as cosmetics and medicine. High growth rates in these sectors, as well as new innovations, such as sustainable packaging from seaweed, to replace oil-based plastics, are reason for optimism about the future of algae cultivation. But apart from pure biomass production, there is a huge potential for developing a sustainable algae industry along the whole value chain, supported by a strong applied research and the valorization of strain collections and genetic resources, as well as patents, new applications, technologies, and product developments. This could be an important step towards developing a bioeconomy, while creating new education possibilities, innovations, services, and jobs. The following five points should be the strategic focus points for developing such an algal bioeconomy and promoting growth in the sector:
Basic and applied research center(s)
Focused mainly on applicative research, product development, technological development, process development, publications, and commercialization of patents
Commercial (local) strain collections
Knowledge collection and commercialization of local strains and biological/genetical resources. Also, a means of biological monitoring of environmental changes or natural conservation
Education (schools and universities)
New study subjects, e.g., “Algae Biotechnology”
Support of startup companies
Creation of a strong network to develop promising go-to the market strategies
Development of tools to finance projects (“algae fund”) and research
Asian producers of microalgae and seaweed are currently the main drivers of growth. The number of algae-producing companies in Europe has grown, but their impact is still low. Algae biomass production in the EU (0.23 Mt, fresh weight) contributed less than 1 % to the global production of 30.4 Mt in 2015 (Camia et al. 2018 ). However, current EU political priorities favor a transition towards a sustainable economy and therefore also the development of an algae sector, e.g., the EU Bioeconomy Strategy (European Commission 2018 ), the EU Blue Growth Strategy (EC, 2012), and the European Green Deal. The EU also funds development aid projects related to algae cultivation, for example, a €8 million project to help Kanembu communities in Chad adapt to the impacts of climate change and develop renewable energies, providing technology for more effective cultivation and drying of Spirulina (European Union 2020 ). The work is done mainly by women. Increase of production volumes and quality could generate more jobs and income for them.
There are many examples of the socio-economic benefit of algae industries in developing countries. The harvesting of Eucheuma seaweed in Zanzibar accounts for 7.6% of the islands GDP, and in total, Tanzania’s seaweed industry employs 30,000 people (The Fish Site 2020 ).
Recently in Bali, seaweed farming has received a renewed boom, after having been dialed down, roughly a decade ago. Due to the Corona crisis, the tourist industry has collapsed, and seaweed farming was rediscovered, generating an income of up to 400 USD a month for workers. This is just over half of what people had before pandemic but shows that diversification could help to overcome such crisis (Channel News Asia 2020 ) .
The cultivation of algae can make an important contribution to the food security of future generations, especially in regions of the world where, due to population growth, the available cropland is likely to be insufficient. This is especially true for Africa, where only 458 to 629 m 2 of cropland will be available per person in 2100 (Rahmann and Grimm 2020 ). If integrated into the agricultural system in a circular manner, as depicted in Fig. 2 , algae cultivation could decrease nutrient losses and greenhouse gas emissions, as well as provide green energy. For this to become a reality, algae production has to become cheaper, in order to compete with cheap, high-quantity products, such as oil. New technology and upscaling of algae production, as is already happening today, can make an important difference. For algae producers, the valorization of strain collections and genetic resources, as well as patents, new applications, technologies, and product developments, is an important way forward. On a grander scale, however, it is important that governments support research programs on cultivation and processing of algae and introduce legislation which incentivizes sustainable production, while discouraging pollution, for example, through the introduction of a carbon tax or similar measures related to pollution by over-fertilization. Only if externalities which are caused, for example, by the burning of fossil fuels, excessive fertilizer use, or deforestation for food production are reflected in the price of products can sustainable technologies such as algae production become competitive in a relatively short time period.
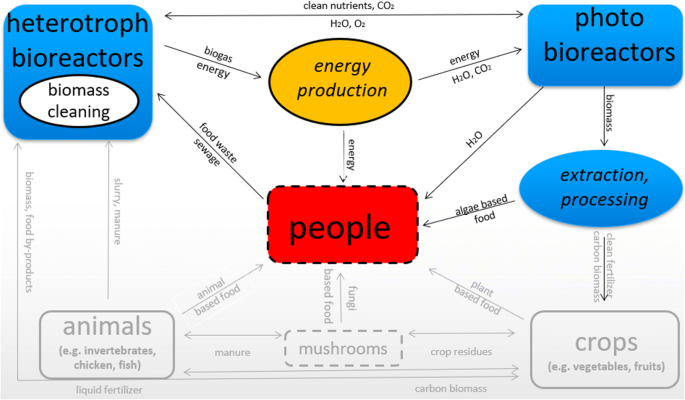
Flow model of a circular food system, as proposed by the LandLessFood project (Rahmann et al. 2020 ), with algae production and related elements highlighted
Ahsan M, Habib B, Parvin M, Huntington TC, Hasan MR (2008) A review on culture, production and use of spirulina as food for humans and feeds for domestic animals. FAO Fisheries and Aquaculture Circular (FAO)
Aßmus E, Weller B, Walter F, Kerner M (2018) Fassadenelemente einer Bioenergiefassade–Entwicklung eines Prototyps. ce/papers, 2(1), 211-220
Bai MD, Cheng CH, Wan HM, Lin YH (2011) Microalgal pigments potential as byproducts in lipid production. J Taiwan Inst Chem Eng 42(5):783–786
Article CAS Google Scholar
Bjerregaard R, Valderrama D, Radulovich R, Diana J, Capron M, Mckinnie CA, ..., Forster J (2016) Seaweed aquaculture for food security, income generation and environmental health in tropical developing countries (No. 107147, pp. 1–16). The World Bank
Borowitzka MA (2013) High-value products from microalgae—their development and commercialisation. J Appl Phycol 25(3):743–756
Buschmann AH, Camus C, Infante J, Neori A, Israel Á, Hernández-González MC, Pereda SV, Gomez-Pinchetti JL, Golberg A, Tadmor-Shalev N, Critchley AT (2017) Seaweed production: overview of the global state of exploitation, farming and emerging research activity. Eur J Phycol 52(4):391–406
Article Google Scholar
Camia A, Robert N, Jonsson R, Pilli R, García-Condado S, López-Lozano R, van der Velde M, Ronzon T, Gurría P, M’Barek R, Tamosiunas S, Fiore G, Araujo R, Hoepffner N, Marelli L, Giuntoli J (2018) Biomass production, supply, uses and flows in the European Union. First results from an integrated assessment, EUR 28993 EN, Publications Office of the European Union, Luxembourg, ISBN978-92-79-77237-5, doi: https://doi.org/10.2760/539520 , JRC109869
Channel News Asia (2020) With foreign tourists gone, Balinese rediscover seaweed farming. https://www.channelnewsasia.com/news/asia/bali-seaweed-farming-tourism-gone-covid-19-coronavirus-13166778 . Accessed 6 Oct 2020
Dismukes GC, Carrieri D, Bennette N, Ananyev GM, Posewitz MC (2008) Aquatic phototrophs: efficient alternatives to land-based crops for biofuels. Curr Opin Biotechnol 19(3):235–240. https://doi.org/10.1016/j.copbio.2008.05.007
Article CAS PubMed Google Scholar
Erlandson JM, Graham MH, Bourque BJ, Corbett D, Estes JA, Steneck RS (2007) The kelp highway hypothesis: marine ecology, the coastal migration theory, and the peopling of the Americas. The Journal of Island and Coastal Archaeology 2(2):161–174
European Commission (2018) A sustainable bioeconomy for Europe: strengthening the connection between economy, society and the environment. https://ec.europa.eu/research/bioeconomy/pdf/ec_bioeconomy_strategy_2018.pdf#view=fit&pagemode=none
European Union (2020) Harvest of hope: spirulina from Lake Chad. https://gcca.eu/stories/harvest-hope-sprirulina-lake-chad . Accessed 6 Oct 2020
Ferdouse F, Holdt SL, Smith R, Murúa P, Yang Z (2018) The global status of seaweed production, trade and utilization. Globefish Research Programme, 124, I
Fernandez S, Srinivas K, Schmidt AJ, Swita MS, Ahring BK (2018) Anaerobic digestion of organic fraction from hydrothermal liquefied algae wastewater byproduct. Bioresour Technol 247:250–258
Guiry MD (2012) How many species of algae are there? J Phycol 48(5):1057–1063
Kuenz A, Grimm D, Rahmann G (2020) Versatility of algae—exploring the potential of algae for nutrient circulation. Org Agric. https://doi.org/10.1007/s13165-020-00308-0
Lundquist TJ, Woertz IC, Quinn NWT, Benemann JR (2010) A realistic technology and engineering assessment of algae biofuel production. Energy Biosciences Institute, 1
Michalak AM, Anderson EJ, Beletsky D, Boland S, Bosch NS, Bridgeman TB et al (2013) Record-setting algal bloom in Lake Erie caused by agricultural and meteorological trends consistent with expected future conditions. Proc Natl Acad Sci 110(16):6448–6452
Mobin S, Alam F (2017) Some promising microalgal species for commercial applications: a review. Energy Procedia 110:510–517
Mishra U, Pabbi S (2004) Cyanobacteria: a potential biofertilizer for rice. Resonance 9(6):6–10
Nhat PVH, Ngo HH, Guo WS, Chang SW, Nguyen DD, Nguyen PD et al (2018) Can algae-based technologies be an affordable green process for biofuel production and wastewater remediation? Bioresour Technol 256:491–501
Notoya M (2010) Production of biofuel by macroalgae with preservation of marine resources and environment. In: Seckbach J., Einav R., Israel A. (eds) Seaweeds and their Role in Globally Changing Environments. Cellular Origin, Life in Extreme Habitats and Astrobiology, vol 15. Springer, Dordrecht
Pulz O, Gross W (2004) Valuable products from biotechnology of microalgae. Applied microbiology and biotechnology 65(6):635–648
Rahmann G, Grimm D (2020) Food from 458 m 2—calculation for a sustainable, circular, and local land-based and landless food production system. Org Agric, 1-12
Rahmann G, Grimm D, Kuenz A, Hessel E (2020) Combining land-based organic and landless food production: a concept for a circular and sustainable food chain for Africa in 2100. Org Agric 10(1):9–21
Richardson JW, Outlaw JL, Allison M (2010) The economics of microalgae oil. AgBioForum 13(2) 2010:119–130
SuSeWi (accessed October 6, 2020), https://www.susewi.life/
The Fish Site (2020) How seaweed farming can help tackle global poverty. https://thefishsite.com/articles/how-seaweed-farming-can-help-tackle-global-poverty . Accessed 6 Oct 2020
United Nations (2018) World urbanization prospects: the 2018 revision. Key facts. With assistance of United Nations. Edited by United Nations, Department of Economic and Social Affairs
Wiley PE (2013) Microalgae cultivation using offshore membrane enclosures for growing algae (OMEGA) (Doctoral dissertation, UC Merced)
Download references
Acknowledgements
Open Access funding enabled and organized by Projekt DEAL.
Author information
Authors and affiliations.
Roquette Klötze GmbH & Co. KG, Klötze, Germany
Jörg Ullmann
Thuenen-Institute of Organic Farming, Westerau, Germany
Daniel Grimm
You can also search for this author in PubMed Google Scholar
Corresponding author
Correspondence to Daniel Grimm .
Additional information
Publisher’s note.
Springer Nature remains neutral with regard to jurisdictional claims in published maps and institutional affiliations.
Rights and permissions
Open Access This article is licensed under a Creative Commons Attribution 4.0 International License, which permits use, sharing, adaptation, distribution and reproduction in any medium or format, as long as you give appropriate credit to the original author(s) and the source, provide a link to the Creative Commons licence, and indicate if changes were made. The images or other third party material in this article are included in the article's Creative Commons licence, unless indicated otherwise in a credit line to the material. If material is not included in the article's Creative Commons licence and your intended use is not permitted by statutory regulation or exceeds the permitted use, you will need to obtain permission directly from the copyright holder. To view a copy of this licence, visit http://creativecommons.org/licenses/by/4.0/ .
Reprints and permissions
About this article
Ullmann, J., Grimm, D. Algae and their potential for a future bioeconomy, landless food production, and the socio-economic impact of an algae industry. Org. Agr. 11 , 261–267 (2021). https://doi.org/10.1007/s13165-020-00337-9
Download citation
Received : 11 November 2020
Accepted : 20 November 2020
Published : 21 January 2021
Issue Date : June 2021
DOI : https://doi.org/10.1007/s13165-020-00337-9
Share this article
Anyone you share the following link with will be able to read this content:
Sorry, a shareable link is not currently available for this article.
Provided by the Springer Nature SharedIt content-sharing initiative
- Biomass processing
- Circular economy
- Landless food
- Climate change
- Ecosystem services
- Find a journal
- Publish with us
- Track your research
Victoria University of Wellington Library

▼ About this page
.jpg)
Title: The Biological and Economic Importance of Algae, Part 2
Author: H. W. Johnston
In: Tuatara: Volume 14, Issue 1, April 1966
Publication details: Wellington
Part of: Tuatara : Journal of the Biological Society
Keywords: Science and Natural History
Conditions of use
Other formats

▼ Browse collection
- Autobiography; Biography; Journals; Correspondence
- Contemporary Māori and Pacific Islands
- Historical Māori and Pacific Islands
- Literary Criticism and History
- New Zealand History
- Science and Natural History
Tuatara: Volume 14, Issue 1, April 1966
The biological and economic importance of algae, part 2.
Previous Section | Table of Contents | Up
by H. W. Johnston Botany Department, Victoria University of Wellington.
In the First Article of this series (Johnston 1965), we dealt with the importance of algae as producers of organic matter—the fuel of the biological world. While this is of fundamental importance to Man, it is not of such direct and personal concern as some of the other algal roles with which we intend to deal. As stated earlier, the range of their influence is rather astonishing and because of this it is difficult to know where to begin — where to make the incision into this corpus of fact and phenomenon.
Algae are photosynthetic, and together with their more highly evolved counterparts on dry land, are the only organisms which can lay aside the vast quantities of food reserves on which the animal world is entirely and irrevocably dependent. It is to be expected therefore that algae will initiate all animal food-chains in the seas and freshwaters, in much the same way as land plants initiate terrestrial animal food-chains. So our next section will deal with algae as articles of food.
Algae as a Source of Food
Since food is the most important requirement of the human body and eating an ever enjoyable pastime, it is perhaps natural enough if we broach this huge topic by considering the algae that Man has used directly as food for himself. Many things that Nature does, Science tries to emulate or do better. Having seen how easily planktonic algae yield to laboratory culture, Science has tried to cultivate algae industrially for food — hoping through advanced technology to be able to improve on Nature's productivity. We must therefore see to what extent Science has been successful in improving on Nature in this sphere. Most important of all we must see how the algae fit into the scheme of things by acting as the biological protyle for aquatic animal foodchains, since these ultimately support the fishing industry — still the second largest industry in the world. It must not be forgotten that fish and not land-animal flesh is the major source of food protein for many millions of people.
In the present article we will deal with naturally-occurring algae as food for man, and the macroscopic marine algae called seaweeds will be our main concern.
For the production of food, people living on continents and large island masses have mostly been accustomed to what we regard as “conventional agriculture” with its cereal crops, pastures and grazing animals. Consequently, the use of seaweeds as food would usually be foreign to such societies except for those folk living on a seacoast — and these would use algae only to allay hunger in times of dire necessity such as crop-failure, to follow a fad, or to perpetuate the practice of an older indigenous population. It so happens that most if not all of the textbooks on algae have been written by people from societies living on land masses pedologically and climatically suited to conventional agriculture. Thus in these books the use of seaweeds as food finds little mention, and one might form the erroneous opinion that they are rather insignificant as articles of diet.
But outside the European, Eurasian, African, Australian and American continents and the larger islands capable of supporting agriculture as we know it, we find many millions of people living on islands where conventional agriculture can never be adopted. For this reason, those people inhabiting myriads of islands in the Pacific and Indian Oceans, the South China Sea and the seas around Borneo, New Guinea and Indonesia have traditionally and of necessity relied on the sea as their major source of food. For this reason, many of these island people have come to rely on seaweeds as an important element in their diet. In certain areas such as Japan this reliance have been so great and the demand so constant that methods of seaweed cultivation have been evolved and put into practice (see later). Acquired knowledge and skill have finally produced specialists in this technique, and given rise throughout time to a thriving industry employing considerable labour. Heavy outside demands have led over the years to the development of an export market.
In Zaneveld's article, Japan does not feature as a great consumer of seaweeds; but the algae listed are mainly warm-water ones. Okamura lists more than fifty which are eaten by the Japanese (see Table III). Among these we recognise the cool-water ones familiar to us in temperate waters. The Koreans are just as keen on eating seaweeds as the Japanese and use almost all those quoted in Table III as well as ‘young fronds of Costaria turneri and Pelvetia wrightii’ (Okamura). The earliest reference to the culinary use of seaweed is found in Chinese writings. The Chinese have always regarded seaweeds as a delicacy; yet according to Table I China uses hardly any at all, despite the fact that much of her coastline lies below 32° latitude. This apparent anomaly calls for comment; but this is delayed till near the end of the article.
Before considering the value of seaweed as food, we must answer the question —‘What do we derive from food?’ Lamentably, the human body is neither photosynthetic nor nitrogen-fixing; so among the first things we need are sugars to provide us with energy for our body functions, and proteins whose constituents we can dismantle and remodel into the types of protein the human body requires. As well as the carbon, hydrogen, oxygen and nitrogen contained in sugars and proteins, we also need about a dozen inorganic elements — sodium, potassium, magnesium, calcium, phosphorus, sulphur, iron, copper, zinc, manganese, cobalt, chlorine, and iodine. Since we are unable to manufacture our own vitamins, we must also acquire a ready-made set of these too — which include vitamins A (or its precursor), B 1-6 , B 12 , C and D. So, when assessing seaweeds as a source of food, we must evaluate them in terms of what they can provide in the way of sugars, vitamins, fats, proteins and the elements mentioned, apart from any piquancy or flavour which might endear them to our palate.
References: Brook, Chapman, Kirby(1950), Newton (1951, 1963), Tiffany.
The monosaccharides can be directly assimilated by the human body and hence are of immediate food value without further modification; the oligosaccharides must be dismantled to their constituent monosaccharide building-units before they are of use; the polysaccharides, likewise, have to be broken down into their monosaccharide units. Being insoluble, the latter group in any case could not be digested before being broken down. If we examine higher plant carbohydrates we find that most occur as polysaccharides, and in not very many plants do we find mono- or oligosaccharides in plenty. The two major exceptions are sugarcane and sugar-beet. We must therefore focus our attention on polysaccharides in greater detail. In assessing polysaccharides in terms of food value, we must consider whether they can be hydrolysed to their monosaccharide units and thus be useful as a source of food, and therefore energy. The human body can hydrolyse the polysaccharide starch to its structural monomer very easily; but the body cannot attack cellulose. To see why this is so, we must look at the structures of cellulose and starch — remembering that both substances are built entirely of glucose units. Why, then, the difference in digestibility?
.jpg)
Alpha-glucose Beta-glucose
.jpg)
Reference : Okamura
Table III: Seaweeds eaten by the Japanese.
cellulose— by cellulolytic enzymes called cellulases which are quite rare and not possessed by many organisms be they plant or animal.
Despite the hundreds of different kinds of herbivorous animals, we at present know of very few possessing cellulase in their kit-bag of enzymes. These biochemical elite include the silverfish, the snail (Helix pomatia) , the limpet (Patella patella) and the chiton. There may be others; but these seem to be the only ones whose oddity has up to the present been recorded in the literature. And yet most of the world's agriculture relies (as does almost the whole of New Zealand's export income) on the digestion of cellulose in the gut of cows, beef cattle and sheep, and other hoofed animals. However, all these are ruminant animals and maintain in their rumen intestinal bacteria and protozoa which excrete a cellulase capable page 38 of hydrolysing the cellulose in pasture plants to glucose. When viewed in this light, almost our entire economy is based on one biochemical capacity of rumen micro-organisms. An exceedingly slender thread!
It appears that the stability of cellulose may be due to its being composed of beta-glucose units, which seem to have a much more stable structure electronically than those of the alpha-glucose form. Maybe this is why cellulose was the carbohydrate selected during evolution as the structural material of plant-life after the latter had appeared on dry land. The difference in stability between the alphatype linkage and beta-type linkage is even reflected in their ease of hydrolysis in acid conditions: alpha-linkage is broken by dilute hydrochloric, but the fracture of beta-linkage requires the use of concentrated sulphuric acid and prolonged boiling. The structural stability of cellulose is not a feature of starch — with its polymerised array of alpha-glucose units whose unions are so easily severed.
Funnily enough, if we cross the border into the zoological world, we see a similar demonstration of the choice on biochemical grounds of the right material for the right job. Glycogen, the main metabolic polysaccharide of animals, is a polymer of glucose units joined by alpha-1:4 and 1:6 linkages. Chitin, the structural material of the insects and crustacea, is a polymer of a nitrogen-containing glucose derivative called N-acetylglucosamine. This polymer consists of N-acetylglucosamine units joined together in beta-1:4 linkage — just as we see in cellulose. This similarity is perhaps more easily appreciated after comparing the formulae of these two structural substances.
.jpg)
Cellulose Chitin
In both plant and animal worlds, the metabolic polysaccharides which must be broken down very quickly for immediate release of energy are built on the alpha-linkage pattern — i.e. starch and glycogen. But the structural polysaccharides which must ‘stand four-square against all the winds that blow,’ even to the extent page 39 of resisting the subtle biochemical weapons of parasite attack, are built on the beta-linkage system — i.e. cellulose and chitin. What is the explanation of this coincidence — trial and error, teleological or theopneustic?
In our digestive interiors we produce the following polysaccharide-splitting enzymes: salivary amylase (ptyalin) which acts to a limited extent on starch and glycogen and breaks them down to dextrin and maltose by hydrolysing alpha-1:4 linkages; pancreatic amylase (diastase or amylopsin) which attacks the alpha-1:4 links of starch and glycogen: and finally the intestinal enzymes for splitting carbohydrates. The latter include maltase for hydrolysing maltose (alpha-1:4 linkage); a specific sucrase or invertase for splitting sucrose; a specific enzyme for hydrolysing lactose: and an oligo-1:6 glucosidase for hydrolysing the alpha-1:6 linkages of the dextrins formed as a result of salivary amylase working on starch and glycogen (Cantarow and Schepartz). Not being ruminants, we cannot break down cellulose; but we can handle starch quite readily and reduce it to its constituent glucose molecules: i.e., we cannot hydrolyse the 1:4 links between beta-glucose units, but we can rupture the 1:4 and 1:6 links between alpha-glucose units. Armed with this information, let us now look at seaweeds as a source of food in terms of hydrolysable polysaccharides, since this class of substance is the main supplier of energy for our metabolic reticulation system.
When assessing the major carbohydrates of seaweeds as energy sources, we should use the same classification for these carbohydrates as we used above.
(a) Monosaccharides
There appear to be no free monosaccharides in seaweeds, but we do find some polyhydric alcohols related to monosaccharides e.g. dulcitol, sorbitol, mannitol and one or two derivatives. Also found are mannoglyceric acid and floridoside, the former being a compound between mannose and glyceric acid, and the latter a compound between galactose and glycerol (Meeuse). These two chemicals have alpha-glycoside links and are not difficult for humans to hydrolyse.
(b) Oligosaccharides
Sucrose has been found in Chlorophyta and Rhodophyta but not in Phaeophyta. This sugar we can handle very easily. Trehalose, also a disaccharide, is found in a few algae but has not yet been reported from the usual edible seaweeds.
(c) Polysaccharides
As in land plants, we find that glucose is a major building unit; but we also find polymers of galactose and a few of xylose and page 40 mannose. These polysaccharides can be classified according to the type of linkage between units — keeping in mind the fact that humans can handle the alpha-1:4 and alpha-1:6 but not the beta-1:4 type. This classification will immediately give a clue to what humans can handle metabolically and what defeats their digestion.
The first point to emerge from this classification is the exotic nature of some of the linkage types which the seaweeds seem to specialise in. These are quite unlike any types found in land plants and therefore quite foreign to the human digestive system's enzyme complement. Let's take each of these linkages and see what the chances are of digesting such polysaccharides.
The first group contains alpha-1:4 and alpha-1:6 linkages. This is well known to our gastronomy because starch and glycogen molecules are based on this pattern. True starches identical with those of higher plants are found in the green seaweeds. This group includes floridean starch, the usual product of photosynthesis in a number of red seaweeds such as Rhodymenia pertusa. It appears to be very closely related to the higher-plant amylopectins. Apparently there are a few 1:3 linkages as well. Myxophycean starch also belongs within this group; but very few blue-greens are used as food and they therefore hardly warrant further consideration.
In the second group we find those polysaccharides with a beta-1:3 linkage. It is now thought that these are probably the most abundant polysaccharides on earth — even usurping the exalted position of cellulose. ‘the greatest’ of the organic carbon compounds. They are also found in highe ‘plants — as callose in grapevine phloem, as a deposit in the cell walls of other plant tissues, and as yeast glucan from yeast cell walls. In algae they are found in the cell walls of some greens such as Caulerpa —but more particularly page 41 in laminarin in brown algae, as paramylon in Euglena , as chrysolaminarin in certain diatoms and possibly all Chrysophyta (Meeuse). The compound of interest to us is laminarin — not that it is widespread in the browns, since it has so far been found in quantity only in Laminaria and Fucus , though it has also been isolated from Eisenia bicyclis (Nisizawa 1938).
The enzyme laminarase which degrades laminarin is rather infrequently encountered. It has been identified in wheat, barley, oats, potato, malt, and in hyacinth bulbs — to match, no doubt, the distribution in these plants of a glucan of this nature. It has also been found in snail juice (Helix potmatia or H. aspersa) (Barry 1941) and in the sea-hare, Tethys punctata (Nisizawa 1939); and laminarin can also be degraded by enzymes in the fore-and midgut of the herbivorous marine snail, Tegula funebralis (Galli and Giese). The question arises — can laminarin be broken down easily in the human body or is it like cellulose in being difficult to degrade because of these beta-linkages? Remembering that the polysaccharide-attacking enzymes produced by humans can operate only on alpha-1:4 and alpha-1:6 linkages, we are forced to admit that a feast of laminarin seems synonymous with a famine of monosaccharide — particularly since it is impregnable to diastase (i.e. amylase) and ptyalin (Barry 1938).
The next group for review contains carbohydrates with beta-1:4 linkage. Here we are amongst old friends — cellulose, xylan and mannan: the newcomer is alginic acid which is a mixture of a polymer of mannuronic acid units and a polymer of guluronic acid units. Cellulose is not common in seaweeds. It has been found in Chondrus crispus (2.2% D.W.), Rhodymenia palmata (2.1% D.W.), Laminaria saccharina (5.7% D.W.), and Laminaria digitata (3.7% D.W.), Mannan occurs in Porphyra umbilicalis — the only record so far in the reds. It has also been found in some of the greens e.g. Codium fragile. This compound consists of beta-1:4 linked mannose units. Xylan (of beta-1:4 xylose units) as we know it in higher plants occurs in red seaweeds too. We are already aware of our inability to do anything with cellulose; and it appears that xylans and mannans along with galactans are also immune to our digestive attack (Tiffany).
As far as alginic acid is concerned, it seems we are unable to handle this any better than we can cellulose because although alginic acid has a -COOH group where cellulose has a -CH 2 OH, it is the nature of the connecting bond between the monomer units which seems to determine ultimate digestibility. This link is the same for cellulose and alginic acid.
We come now to groups which are hybrid as regards type of linkage; in which beta-1:3 alternates with beta-1:4 (as in agar and its allies) or where there is a different ratio of beta-1:3 to beta-1:4 (as in the particular type of xylan called ‘dulsin ’ found page 42 in dulse, Rhodymenia palmata ). Since human enzymes will not attack either of these linkages, we are unable to claim any of the photosynthetic prizes locked away in these polysaccharides. And the same would apply to fucoidin — found in some of the laminarians, Fucus, Chordaria and others. Bacteria from a sheep's rumen have been found capable of hydrolysing dulsin — but one would expect this since some of these bacterial types can split the beta-1:4 link in cellulose.
Thus it seems that seaweeds cannot be relied upon to provide a large proportion of usable carbohydrates. Tiffany has this to say — ‘About 65% of the dry weight of most edible algae is composed of complex carbohydrates with rather low digestibility. In fact Oshima places the digestibility of edible seaweeds of Japan at 67.7% which is lower than that of any carbohydrate of common foodstuffs.’ In 1906 Saiki wrote —‘Experiments with a variety of alga preparations (Irish moss, kombu, wakame, asakusanori, kanten (agar-agar)—H.W.J.) containing a large proportion of polysaccharide carbohydrates indicated that the latter were not readily transformed to sugar by carbohydrate-digesting enzymes of animal origin and scarcely more readily by vegetable enzymes or bacteria. Corresponding with this, the digestibility and availability of such products in the alimentary tract were found to be very imperfect in both man and animals.’ Anybody who has grown fungi and bacteria will know that not many organisms of these two classes will attack agar, although most would excrete carbohydrate-splitting enzymes of some kind into the growing medium. It looks then as if we can dismiss seaweeds as a high-class source of carbohydrates.
Kirby (1950) made a pertinent observation about the digestibility of seaweeds — ‘It is interesting to note that in Japan the weeds are often left until they have been attacked by fungi. These may help to break down the weeds so that they are more easily digested when eaten.’ Fungi are well-known for their ability to break down most things, and few of the algal polysaccharides are likely to withstand the onset of fungal attack. Some are even equipped with cellulases to break this intractible beta-1:4 linkage. One encounters more bacteria capable of breaking down agar than fungi; and because of this fact, maybe bacteria are just as important as fungi in this softening-up process. In many cases this predigestion would be necessary because some seaweeds appear to be rather indigestible. Ulva , for instance, has been described by one writer as something which would tax the digestive system of the mighty. There is a certain amount of conflicting evidence about the digestibility of seaweeds. If, in the experiments, the seaweeds were eaten fresh, the digestibility could quite easily be low; but if the seaweeds had been kept for sometime and thus were partially predigested by fungi and bacteria, the results would understandably page 43 be different. This may explain the discrepancy. Several writers have implied that people used to eating seaweed may have brought about the selection of intestinal micro-organisms capable of processing this type of food. Because of this, they may be able to digest seaweed to a greater extent than their less fortunate friends who have not eaten this type of food frequently enough to bring about about an ecological change in the types of the intestinal bacteria.
If we compare the protein content of algae with that of the garden pea (Pisum sativum) , we find that certain seaweeds contain more protein than do green peas — whose content is about 27% D.W. (Spector). While the seaweeds are not rich in protein when compared with animal sources, what is present in some would make a useful supplement to the diet. (Additional protein figures are quoted below).
Green peas are quoted as having 1.58% D.W. (Spector). Other edible seaweeds which have fat figures similar to these can be dismissed as unimportant sources of this high-energy form of food.
The fatty acids are of the same type as those found in higher plants. Of the saturated-acid series, palmitic (C 16 ) is found in highest concentration — followed by myristic (C 14 ) and stearic (C 18 ) in that order. Among the unsaturated acids, oleic (C 18 ) is present to the greatest extent followed by palmitoleic (C 16 ) and then gadoleic (C 20 ) (Fogg). Humans are used to handling these molecules and what little fat is present could be easily assimilated.
These figures reveal the high potassium content of most of these seaweeds as well as high amounts of sulphur, sodium and chloride. In those cases given, calcium is quite high; but the phosphorus figure seems low when compared with that for garden peas (0.44% D.W.).
There is not a great deal of information available on the minor-element content of seaweeds. Black and Mitchell report some analyses for several brown algae gathered off the Scottish coast in early summer 1949. Except for ash, the other figures are expressed as parts per million (p.p.m.) on a dry-weight basis.
They also give a figure called the ‘concentration factor’: this is the ratio of minor-element content in the fresh seaweed to the content of the same minor-element in the sea-water.
(No Fe figure was given by them for the sea-water analyses).
Seaweeds vary in their contents of these elements. For instance, it seems that minor-elements, which may have some role in reproduction, are lower in quantity in sterile fronds than in sporulating ones. Then there is seasonal variation, as well as variation due to location, proximity to land drainage, type of shoreline rock. There is also the variation within the plant itself, for we see that the minor-element content is higher in Laminaria digitata stipe than in the frond.
The presence of iodine has been known for a long time — seaweed was the first commercial source of this element. Arsenic has also been known to be present. Many other elements are found — such as nickel, lead, tin, vanadium, titanium, chromium, silver, strontium. The presence of the latter calls for some comment. The strontium figures given below are also taken from Black and Mitchell and represent analyses done on seaweeds collected in winter time.
This differential concentration of strontium between groups may occur because brown algae contain alginic acid which can act like a cation exchange resin whereas the greens and reds do not contain this chemical.
The absorption of strontium by certain algae is highlighted in the following analysis of Macrocystis pyrifera — expressed in p.p.m. on a D.W. basis (Wilson and Fieldes).
In relation to metabolically required minor-elements it is interesting to see that iron is again high, with zinc next in order, followed by copper, manganese, molybdenum and cobalt.
We will now examine seaweeds as a source of vitamins — the last group of essential requirements for human nutrition to be considered. Beta-carotene is present in all seaweeds, and since it is the precursor of vitamin A, there should be no shortage of this vitamin in people who include seaweed in their diet. The beta-carotene of several algae investigated is not much affected after harvest: but in one, Rhodymenia palmata , there is a very quick enzymatic breakdown unless the enzymes are killed (Haug and Larsen).
Apparently green seaweeds contain more than red, and both are higher than brown. It has been shown that some algae can accumulate cobalt to the remarkable extent of producing a concentration factor of 10,000 (Ericson). The origin of the B 12 is bacterial — due either to the presence on the algae of epiphytic bacteria which synthesize this vitamin or to the occurrence of these bacteria in the surrounding sea-water. In either case the vitamin is taken up by the seaweed and accumulation occurs. ‘Bacteria from algae which were poor in vitamin B 12 , generally produce small amounts of B 12 , while bacteria from vitamin B 12 -rich algae formed larger quantities of the vitamin’ (Lundin and Ericson). These authors also suggested that red and green seaweeds may have higher B 12 contents than brown because the former ‘often have greater surface areas per gram dry weight than the brown algae.’
Vitamin C is well represented. Ascorbic acid content of Fucus vesiculosus may reach 77mgs./100gms. wet weight — which is considerably higher than lemon juice at 31-57mgs./100gms. Vitamin C content also varies with season and depth (Mautner). Several browns compare favourably with many fruits and vegetables as sources of B 1 and C (Mautner). Lundin and Ericson stated ‘it can be concluded that many marine algae are good sources of vitamins;’ and this would seem to be the case.
From a dietetic point of view, we lack a great deal of analytical information on the constituents of seaweeds. The little page 49 that is available and quoted here does not permit us to make any well-founded generalizations. But what there is points to the fact that the seaweeds, like many of our home-grown vegetables, seem to emerge mainly as suppliers of minerals and vitamins with a possible contribution of proteins in some cases. Thus, many islands edaphically unsuited for the culture of land vegetables may be encircled instead by a ring of seaweeds — the marine equivalent of terrestrial vegetables. The fringing sea, therefore, can be regarded as a marine vegetable garden — one which requires no cultivation and harbours neither weeds nor pests apart from a few fungi. It is really a colossal hydroponics system which is maintained in uniform condition without the supervision of Man. What a fabulous garden! On land the soil serves as a medium for anchoring the plant as well as a source of nutrients; but water has always to be supplied. Similarly, a rocky or coral shore-line provides a medium for anchoring the plant, with the added advantages that the plant is always bathed in a never-ending supply of its nutrients and a shortage of water is inconceivable. In many ways, the sea makes a better vegetable garden than the land-based one on which we usually spend so much time and effort, and is ideal for those situated along a coast-line.
The absence of insect pests to ravage this marine vegetable garden is rather interesting to ponder. Many land plants are poisonous, and their poisonous nature is thought by some to have been evolved through selection as a survival mechanism against predation by insects and other forms of animal life. But one never sees reference to poisonous seaweeds. Some algae are toxic — but these are planktonic and microscopic. Maybe it is too soon to state categorically ‘There are no poisonous seaweeds!,’ because future research may reveal examples. There are certain seaweeds, for example Desmarestia , which would not be toxic in the normally-accepted meaning of the word but might have to be considered hazardous because of the relatively high sulphuric acid content. At the moment no truly poisonous seaweeds appear to have been reported. Alkaloids are the toxic chemicals present in many terrestrial plants: for example nicotine, colchicine, coniine (from hemlock), ricinine (from castor oil seeds), atropine and hyoscyamine (from the Solanaceae), strychnine and brucine, curine and curarine (from Strychnos ); and all the opium poppy alkaloids such as narcotine, morphine and codeine. It seems that alkaloidal compounds have not been found in seaweeds so far. Another often-found plant poison, oxalic acid, has not yet been reported although two of its non-poisonous cohorts — citric and malic — are known to occur.
This apparent lack of poisonous seaweeds is of some consequence since any seaweed — apart from those with a violently acid taste — could therefore be used as food by starving castaways. Looking page 50 through one or two books on survival, one finds no mention of the use or value of seaweeds under such circumstances; yet they would provide vitamins and trace elements not otherwise readily obtainable, as well as small amounts of proteins. Knowing how people of the Pacific and other areas have used seaweeds as food for centuries, one finds it difficult to understand why the recommendation of this practice should have been omitted from manuals on survival when precedent is centuries old and hoary with age.
In what form are the seaweeds eaten? How are they prepared for the table? In Western European countries such as Scotland and Wales, Porphyra laciniata (popularly called ‘laver bread’) has been collected and eaten for centuries. It was harvested and washed free of extraneous materials and slime, shredded very finely and kneaded like dough into balls or rolls. These were eaten raw or fried with oatmeal and butter (Newton 1957). Or it was pickled and stored in stone jars. ‘It was then served cold with oil, vinegar, pepper and a dash of sugar —’ (Newton). One of the earlier delicacies was moor mutton with laver sauce. The sauce was made by boiling the cleaned weed ‘to a stiff green mush; two cupfuls of this were added to a knob of butter and the juice of half a lemon or Seville orange (not a sweet orange)’. This mixture was beaten and served hot (Hartley).
Some are eaten as dessert: Caulerpa racemosa var. clavifera is quite often eaten as a dessert after a rice meal. Padina australis is made into a gelatine-like sweetmeat in Indonesia; and in the Phillipines, Agardhiella is made into a sweetmeat by boiling with sugar and spices. Ulva lactuca is made into a soup or is used in a garnish for other dishes. Others are prepared in various ways, such as — page 51 dried, boiled and eaten with bacon — Chaetomorpha javanica with coconut milk or vinegar — Sargassum granuliferum —Moluccas as a vegetable — Sargassum siliquosum — Phillipines with coconut milk — Turbinaria ornata — Moluccas as a vegetable — Gracilaria eucheumoides — Phillipines.
And if some of these accounts have not activated your salivary glands, you might like to tantalise them with either of these two recipes. In Burma Catenilla nipae is eaten raw (or boiled) mixed with oil of Sesamum indicum , salt, powdered fruit of Capsicum annuum , fried rhizome of ginger ( Zingiber officinale ), onion and garlic. On the island of Bali, Hypnea cervicornis is collected, dried and bleached on the beach for 3 or 4 days. It is then boiled, filtered and cooled; the resulting jelly is eaten with palm sugar and grated coconut.
Because of their agar content, a number are used for making jellies. Notable amongst these are Corallopsis salicornia; Eucheuma edule, E. gelatinae, E. horridum, E. muricatum; Gelidium amansii, G. rigidum, G. latifolium; Gigartina spp; Gracilaria confervoides, G. lichenoides, G. taeniodes; Hypnea divaricata, H. museiformis.
Japan ranks very high as a user of seaweeds; and for this reason we will now consider in some detail how the Japanese prepare and use some of their seaweeds. Most are eaten raw after having been thoroughly washed. These include Porphyra, Nemalion vermiculare, Undaria pinnatifida, Cladosiphon decipiens, Mesogloea crassa, Grateloupia flabella — and many more. Others are blanched by boiling just enough to change their colour: this treatment is accorded to Gracelaria confervoides, G. compressa , young shoots of Codium , and Meristotheca papulosa. All of these may be eaten as side dishes to the regular meal or may be taken with saké. Others such as Laminaria, Undaria, Cladosiphon, Porphyra, Gloiopeltis , are used in soups. Many are eaten with boiled rice — for instance Laminaria, Eisenia, Ecklonia spp., Undaria spp., Porphyra (mainly tenera ), Enteromorpha ; but they require some form of pre-treatment. Laminaria, Undaria, Porphyra and Enteromorpha are sun-dried before use, while Eisenia and Ecklonia are boiled first to remove an astringency before being sun-dried.
Okamura states that of all the seaweeds eaten in Japan the most important are Porphyra, Laminaria and Gelidium. Some Porphyra is eaten fresh but most is sun-dried and preserved in thin sheets. The following passages referring to Porphyra and Laminaria are taken from his article.
‘These dried Porphyra sheets are extensively used in Japanese cooking, and are the special delight of children. The sheets, almost black, are gently heated over the fire until the colour changes to green, when they also become quite crisp. Used with soy the taste is much improved. Porphyra sheets are an essential article in the page 52 preparation of ‘sushi,’ which may best be described as rice sandwiches. To the boiled, hot rice which has been mixed with a little vinegar, certain foods and condiments are added, and the whole is then spread over the Porphyra sheet. It is then rolled up and cut transversely into smaller cylinders. The Porphyra being fairly tough, holds the rice and the ingredients together, acting the part of a sausage skin. Porphyra is also cooked with soy and made into a paste called ‘norino - tsukudani.’ This condiment, which is greatly relished, is an expensive luxury, with the result that cheaper substitutes are made from Monostroma, Enteromorpha and Ulva. '
‘‘Kombu’ is a general term for several species of Laminaria , chiefly L. japonica and L. ochotensis , both widely consumed for food in Japan. ‘Kombu’ is prepared in a dozen or more ways. The most important form in which it is manufactured is shredded, greendyed ‘kombu.’ This is prepared by dyeing the dried Laminaria green in a large kettle containing a boiling solution of malachite green. The dyed kelp is drained and partially dried. After partially drying, the fronds are flattened out and arranged in wooden frames in piles. Each pile is then tightly compressed and held by four transverse cords. When the frame is completely filled with the evenly-arranged pieces, the whole mass is compressed by means of ropes, wedges, and levers. One of the sides of the frame is then removed and the ‘kombu’ is shredded by means of a hand plane The shredded ‘kombu’ is spread out on boards or on mats and dried in the open air. When the surface of the shreds has become dry, it is packed for shipment.’
‘Other forms are manufactured by scraping the epidermis, the remaining green covering, and the thick white cores of the fronds, etc. These scrapings take the form of exceedingly thin and delicate filmy sheets and strips.’
‘Dried ‘kombu’ is used by itself as confectionery and also in the candied state. It is also ground into fine powder to be used in sauces and soups and to be sprinkled on boiled rice like curry powder or to be mixed with other ingredients for making cakes, etc. When boiling water is poured on a quantity of the finely chopped ‘kombu’ a sort of substitute for tea is obtained.’
‘‘Kombu’ is used directly in various ways, not only by itself, but with other ingredients in the preparation of stocks. Bean-curd prepared with this stock is much appreciated by ‘saké’ drinkers. ‘Kombu’ is also cooked in soy and salt and used as a pickle. It is also used as a pickle for putting into ‘miso’ (salted bean-paste, ‘saké,’ or ‘mirin’-lees, and rice-bran mash (‘nukamiso’). ‘Kanten’ made from Chondrus elatus , is also used in this way in Chiba Prefecture.’
Gelidium, Ceramium (Campylaephora) hypnaeoides, C. boydenii and various species of Gracelaria are used in the manufacture of agar page 53 — or what the Japanese call ‘kanten.’ The process will be dealt with in a later article, but the end-product has for centuries been one of the favourite sea-foods of the Japanese and Chinese. ‘Kanten’ is used for making jellies and as a thickener of soups, sauces and gravies — in much the same way as we use flour and cornflour. It has found its way into many forms of food now — even into puddings and desserts.
Because of our ready access to good soil and our ability to use this to cultivate vegetables, we never think of the possibility of using a stretch of rocky coastline as a site for cultivating plants. However, this idea has become a reality and has been practised for centuries especially by the Japanese, and also by the Filopinos in the North of the Phillipines, by the Chinese at Lienyunkang and possibly by the Koreans. (This reference to seaweed culture by the Chinese is the only one known to the writer, and no further detail can be given. Knowledge of this comes solely from a photograph in a book entitled ‘People's Communes,’ edited by the Ministry of Agriculture, People's Republic of China). Porphyra tenera (amanori) is a favourite seafood in Japan and demand has always exceeded supply. For this reason a technique has been developed for the cultivation and harvesting of this red seaweed. To understand the mechanics of the process, it is necessary to be familiar with certain features of the life-cycle — which is as follows.
There are two different generations in this life-cycle — a leafy thalloid plant which is the main macroscopic plant called Porphyra ; and a filamentous microscopic phase referred to generally as Conchocelis (for reasons which need not concern us in this article). In tracing this life-cycle let us start with the leafy thallus. In the early spring with the approach of long days, this phase begins to senesce and the plant body forms carpospores; with increasing senescence the tissues begin to disintegrate and finally the carpospores are released into the open water. These germinate in the late-spring to early-summer to form the filamentous Conchocelis phase which needs the long days of summer to mature. With the shortening days of the late-summer, this phase forms monospores which when released germinate to form the leafy Porphyra. This grows throughout the short days of autumn and winter and with the onset of the lengthening days of spring begins to form carpospores and degenerate. And so the cycle carries on. The edible phase is the leafy Porphyra which grows over the autumn and winter.
Now let's see how the practice fits the theory. In late summer fishermen plant out bundles of twigs and bamboo shoots by embedding them very securely in holes which they make in the mud. This is done at low tide. These close rows of brush intercept and form a place of attachment for the monospores released from the Conchocelis page 54 phase at the end of summer. It has been found that the monospores germinate better under high saline conditions, and thus the brush is planted in areas where the salinity is high. The monospores germinate; and while the plants are still young the brush is transferred to areas of low salinity near a river mouth, since experience has taught these folk that the highest quality of amanori is grown in areas of low salinity and higher nitrogen. The fresh water carries fair quantities of nitrogenous fertiliser which produces amanori of suitable succulence. The young plants grow throughout the autumn and early winter and are harvested in mid-winter (December-January) when mature and before degeneration starts. The plants are merely pulled off the brush. Some plants escape harvest and under the environmental stimulus of lengthening days form carpospores from about the end of January to mid-February. These germinate to form the Conchocelis phase — which grows during the summer on stones, gravel and other bottom detritus. During the summer the fishermen remove the old brush and set out new material — in time to intercept during late-summer the released monospores from the Conchocelis phase which has received its reproductive cue from the shortening days. Fig 1 sets out the cycle in a diagrammatic form — which shows more clearly the coincidence of practice and theory. The only point to remember about the coincidence is that the practice was worked out centuries before the theory was known!
The harvesting of amanori is carried out in mid-winter by women and girls. It appears that Japanese women, like their European counterparts, can tolerate more cold than the men: conceivably, this is why the harvesting is a female occupation. One other interesting point is that in mild winters trouble is experienced with a marine fungus attacking the amanori thallus — a marine example this time of the troubles which beset those who engage in intensive and close cultivation of one particular plant. It seems axiomatic that no matter where one indulges in a monoculture — either on land or in the sea — the ogre of parasitism will surely appear.
When harvested, the amanori fronds are washed in tubs of fresh water to remove sand and other adhering matter. The plants are sorted for quality, chopped finely and spread out uniformly on bamboo-mats to dry in the air. These sheets are then baled and sold. In this form they are sold under the name of ‘asakusanori.’ Before being eaten, the asakusanori is crisped over a fire in which process it changes colour from dark brown to green. It is rubbed between the hand and dropped into soups or sauces to which it imparts a pleasant flavour.
.jpg)
A diagram showing how the life-cycle of Porphyra is related to the seasons of the year and the practice of the fishermen.
‘The field work of nori-culture begins in the autumn when nets are hung on racks at ground level just as the spores emerge into the water. Since the harvest of the season depends greatly upon the abundance of the spore attachment, the relationship between the occurrence of the spores and hydrographical as well as meteorological conditions has been studied over a period of many years. Attempts have also recently been made to make quantitative determinations of the spores in sea-water. To give growers the maximum benefit from those various researches, the local experimental stations often announce over the radio the best time for collection.’
‘Artificial seeding of the nori spores is carried out to obtain spores for the season from the carpospores cultured since the preceding winter. The result of this research, however, still remains to be seen. Another method of artificial seeding recently found is connected with a species of laver, Porphyra yezoensis , whose distribution is limited to the northern part of Japan. The monospore formed on the summer plantlet of this laver is made to attach and grow on the collecting net.’
‘The height of the spore collector on the racks is adjusted in accordance with the growing stages of the laver and the seasonal fluctuation of the current and water temperatures so that the growth of the laver may be promoted, avoiding as much as possible any damage by disease. Fertilisers are also used in the growing areas alongside the spore collector.’
Earlier, it was mentioned that Porphyra can be attacked by a fungus in warm winters. The only treatment known so far for combating this disease is to move the racks to higher ground so that the seaweed will remain out of the water for about three hours each day. This is so simple but so ingenious, since the seaweed can stand a limited amount of exposure to air because of its mucilaginous covering, but the fungus (presumably, like most of its class) lacks this covering and its cells are desiccated and therefore killed.
In what other way could this fungus be controlled? Ordinary methods of using fungicides as practiced on land are just not applicable in the sea because of the physical nature of the page 57 environment. One cannot imagine applying a fungicide in wettable powder form, or as an emulsion to an area of seaweed under water. How would the fungicide stick to the alga in a liquid medium? And what would happen when the tide goes out? The constant surge of the water would prevent the chemicals from settling on the diseased plants to any great extent. If the tidal rise and fall was such that the beds of Porphyra were exposed at low tide, presumably a spray could be applied then before the tide came in; but would the chemical adhere to the algal surface when the tide did come in? Would sea-water inactivate our present fungicidal compounds? Under the circumstances, the locals have evolved by far the best form of control and possibly the only one for a problem such as this.
A rougher type of cultivation was practised by the Irish for cultivating Fucus vesiculosus , which they collected and used as a fertiliser (Newton 1951).
One further curious point that emerges from this study of seaweeds as food is that people in different parts of the world have picked on one particular genus of seaweed to eat — namely Porphyra. Maoris in New Zealand gather and relish it. The Chinese in New Zealand collect it and send it home to China as do the Chinese in California. The Chinese in southeastern Alaska collected and ate Porphyra laciniata. The Japanese, as we have seen, farm several species of Porphyra. The Koreans eat it, and it is farmed in North Luzon in the Phillipines. It was eaten by people around the western European coasts, in England and Wales, and also in Chile. Apart from any appeal to the palate, all the members of this genus may be like Porphyra laciniata which, as we have seen earlier, has such a high total nitrogen figure. It is an interesting thought that these different peoples, without the aid of analytical chemists to tell them what is good for them to eat, should have selected in each case the local species of a genus that possibly is the most nutritious seaweed available, because of its high nitrogen content and its stock of major and minor elements. Funny how empirically-determined practice is so often substantiated by research! And yet we are all too often inclined to denigrate it because it was evolved by a non-academic society.
Earlier, we saw that China scarcely figures at all in Table I either as a user of seaweeds for food or as a substrate for their occurrence. This situation contrasts strongly with that of her near neighbours, Japan and Korea; and the disparity merits closer investigation.
The earliest extant writings reveal the importance and esteem with which seaweeds were regarded by the ancients of China, who have eaten these plants since time immemorial. It has been said that the Japanese acquired the habit of using seaweed as food from the Chinese — in much the same way as they learnt the techniques of flower-arranging, development of ming trees, and other things cultural as well as horticultural. In those early days ‘the term for algae was also used in a complimentary sense, as in praise of the thinking of a learned man, to signify that his thoughts were ordered as systematically as the parts of an alga’ (Kirby 1950). In a book by Sze Ten in about 600 B.C. it is written that ‘some algae are a delicacy fit for the most honourable guest, even for the king himself.’ The Chinese attitude to seaweeds differs completely from that of the ancients of Rome who regarded these plants as the most loathsome and useless of the biological world. In fact the Romans used the term ‘seaweed’ as a synonym for the nadir on their scale of what was useful and useless or obnoxious. Hence the reference by Vergil in his Eclogues VII, line 42: ‘horridior rusco, proiecta vilior alga’ — prickly as butcher's broom and useless as seaweed which has been cast up. Horace in his Fifth Satire reckons that family and virtue, without wealth, are as worthless as seaweed; and in his Ode to Aelius he speaks of ‘inutilis alga’ — worthless seaweed (Brook).
In view of the esteem bestowed by the Chinese on seaweeds, it is surprising that an industry centred around the latter's cultivation and harvesting was never developed to the extent of achieving mention in the history books. One imagines that the early knowledge of seaweeds’ delectability and their use as effective herbal medicines would surely have led the inhabitants to ensure a good supply to meet this type of luxury demand; and yet no indications of this are found. This enigma becomes more complex when we come to look for present-day references to the marine algal flora of China. There are very few. In Table IV those seaweeds that are eaten and sold in the markets are listed (Kirby 1950). But when we come to look for a source of these seaweeds in China, we find an unbelievable paucity of indigenous marine algae along her coast. This is all the more intriguing when we realise that the country possesses about 5,000 miles of coastline. Of course we are not entitled to believe that the last word has been written on the incidence of seaweeds around the China coast.
References : Cotton, Gepp, Grubb, Kirby (1950), Tseng and Tang.
before considering the oddities of distribution, we must become acquainted with certain physical features of the coastal land-form and sea-water characteristics because these two things seem to explain much that is puzzling.
The seas in the immediate vicinity of the Chinese coastline are dominated by the huge outflow of fresh water from the major rivers — especially the Yangtze Kiang and the Hwang Ho whose anglicised name (Yellow River) has been applied to the sea surrounding its mouth (the Yellow Sea). The southern end of the Yellow Sea also receives silt and fresh water in even greater quantity from the Yangtze, which emerges close to Shanghai. Immediate effects of all this fresh water are that the salinity of the sea is altered, as well as its transparency and the nature of its floor. Thus, much of the coast of Northern China is unfavourable for a good seaweed flora due to decreased salinity and transparency of the water, and also to the lack of a suitable substratum. Cotton remarked that the Gulf of Chihli (the upper part of the Yellow Sea) is for the most part muddy. The coastline of this Gulf round to the Shantung Peninsula and south of this peninsula down to the mouth of the Yangtze is deltaic in origin, having been built up from silt brought down by these rivers. Shantung however is a rocky promontory. Little wonder that not many algae grow page 60 when they lack a firm substratum for attachment: what substratum is there is being covered over by new layers of silt, since the coastline rapidly encroaches on the continental shelf. It has been estimated ‘that the Yangtze-Kiang alone deposits annually sufficient sediment to create in the Pacific each year a new island, 1 mile square and 15 fathoms deep, while the floor of the Gulf of Pei-chi-li (i.e. Chihli — H.W.J.), on the shores of which Pei-tai ho is situated, is silt from the Hwang Ho’ (Grubb). Coming further south and then west from Shanghai — that is from Chekiang to the large island of Hainan, the coastline is again rocky without being interrupted by any more large rivers — except the Siang Ho which meets the sea near Hong Kong.
The second feature to consider is the geological nature of this shoreline. Most of the coast bordering the Yellow Sea is composed of alluvium — except for the Shantung peninsula. Grubb, describing the substrate near the Pei-tai-ho, said that the ‘coast is alluvial, with a few jutting out reefs of rocks. The strata in this district appear to be of quaternary or tertiary origin, and the rocks are a hard coarsely-grained granite. They do not offer any very satisfactory foothold for algae, and the flora, even in rock-pools, is confined almost wholly to species of Corallina, Hypnea , and Gelidium .’ Gepp quoted a letter from a surgeon of the Royal Navy who collected some seaweeds on an island close to Wei-hai-wei: ‘the rocks are metamorphic, consisting of beds of quartzite, gneiss, crystallite, and limestone cut across by dykes of volcanic rock and granite. Mica abounds everywhere. Where the seaweeds were found, the rocks were mainly granite and gneiss.’
The third feature contributing to this paucity of seaweeds is water temperature. At its western extremity the Pacific North Equatorial current turns north along the eastern side of the Phillipines and becomes the Kuro Siwo, the ‘Black Current’ or Japan stream. This forks, and one part comes up on the eastern side of Formosa and Japan as far as the heel of Honshu, then turns east across the North Pacific (Grubb). The other arm of the Kuro Siwo flows on the western side of Formosa and bathes the coast of China. As one would expect, this is a warm current having a mean temperature of 80° F.— which is about 5°-15° warmer than the surrounding water (Grubb). This temperature therefore effectively excludes the cool-water seaweeds of the Fucales and Laminariales.
It will now be appeciated how the interplay of these ecological factors helps to reduce the size of the Chinese seaweed flora. When we refer again to Table IV we find a number of algae sold in the markets which are not found anywhere on the China coast as far as the present records show us. These include Ecklonia cava, Laminaria spp., Chondrus elatus, Eucheuma papulosa and E. spinosum. It is obvious these must have been imported — more than likely from page 61 Japan, since this country would probably be the nearest source of the cool-water weeds. Actually, Japan has for some time enjoyed a large export business in seaweed products to China. Imports by China of all kinds of seaweeds during 1935-37 averaged about 326,000 tons, most of which came from Japan (Kirby 1950). Let's assume the imported material averaged about 15% of moisture and the fresh wet weed about 45%. This means that the import figure quoted above could be equivalent to more than a million tons wet weight of seaweed! The processing of a million tons of seaweed per year amounts to a sizeable industry — especially when it is remembered that there is little mechanization used in the early preparative stages and that all handling must therefore be manual.
Embassy exchanges between China and Japan have been recorded for almost two thousand years (Reischauer), and it is known that the establishment of trade relations has always been one of the aims of the Japanese embassies since the first mission went to China in 57 A.D.. Over the period 838-847 A.D. a diary was kept by a Japanese Buddhist monk who accompanied one of these embassies. In this he mentioned that Kombu was taken to China as one of the articles for which the Japanese hoped to develop a market. It could be that trade in seaweeds between these two countries has been occurring for well over a thousand years. When we couple production for Japan's home market with that needed to provide such a large export quantity (assuming present day exports to be about the same as previously!. we are forced to realise how large the seaweed industry in Japan must be. However, this is getting more into economics; and although it is intended to look at the economic aspect of seaweeds, this must be delayed a while.
So we will close this section now, leaving you with the thought that people should not be like the Romans and regard seaweeds as utterly useless: on the contrary, these plants can supply all the minerals and most if not all the vitamins needed by the human body. Spinach cannot do any more than this! Having the population explosion in mind, one need not romance too wildly to envisage the use of small factory-ships to collect the cold-water seaweeds and process them as food additives.
And here is a special thought for those to whom the use of artificial fertilisers, hormone weedkillers and pest-control chemicals is an anathema. The eating of seaweeds would provide a never-ending supply of ‘pure and wholesome food’ — unsullied by pesticidal members of the Periodic Table, uncontaminated by chlorinated hydrocarbons and unpolluted by organo-phosphorus compounds. Being plants, the seaweeds do not ingest food that has already been elaborated; they start from the ground floor up, so to speak, and build their own complex molecules from simple chemicals obtained from the surrounding sea. They therefore do not come into contact with such compounds as DDT through being a link in a food page 62 chain in the same way as do members of the zoological world. Thus one would not expect to find a build-up of pesticidal chemicals in this type of plant. Since chlorinated hydrocarbons such as DDT are fat-soluble, they will concentrate in fatty or oily tissues. We saw earlier that seaweeds seem to have low fat contents. In view of these two facts, it is inconceivable that seaweeds could be contaminated to the degree found in certain marine fish. For this reason, the seaweeds may constitute the last major section of the botanical world to still retain a pristine chemical purity and because of this they should be regarded as highly suitable fare for those worried about Man's latest weapons against the insect legion.
Barry, V. C. , 1938. The Preparation, Properties, and Mode of Occurrence of Laminarin. Sci. Proc. Roy. Soc. Dublin 21: 615-22.
—— 1941. The Hydrolysis of Laminarin. Sci. Proc. Roy. Dublin Soc. 22: 423-429.
Bersamin, S. V. and others, 1961. Some Seaweeds Consumed Fresh in the Phillipines. Proc. Indo-Pacific Fisheries Council 9th Session, Section 2: 115-119.
Black, W. A. P., and Mitchell, R. L., 1952. Trace Elements in the Common Brown Algae and in Sea Water. J. Mar. Biol. Assoc. 30: 575-584.
Bowen, H. J. M., 1956. Strontium and Barium in Sea Water and Marine Organisms. J. Mar. Biol. Assoc. 35: 451-460.
Brook, A. J., 1949. The Seaweeds and Their Uses. New Biology 7: 89-103.
Cantarow, A., and Schepartz, B., 1962. Biochemistry (3rd Edition). Saunders, Philadelphia.
Chapman, V. J. 1950. Seaweeds and Their Uses. Methvens, London.
Citharel, J., and Villeret, S., 1961. Researches on Nitrogen Metabolism of a Number of Marine Algae from the Breton Coast. Fourth International Seaweed Symposium. (Proceedings published by Pergamon Press, London 1964) pp. 291-300.
Collado, E. G., 1926. Quoted by Zaneveld 1955.
Cotton, A. D., 1915. Some Chinese Marine Algae. Bull Misc. Inform. Royal Botanical Gardens, Kew 1915 (3): 107-113.
Ericson. L.-E., 1952. Uptake of Radioactive Cobalt and Vitamin B 12 by some Marine Algae. Chem & Ind. 1952: 829-30.
Fogg, G. E., 1952. The Metabolism of Algae. Methvens, London
Galli. D. R., and Giese, A. C., 1959. Carbohydrate Digestion in a herbivorous Snail, Tegula funebralis. Jour. Exptl. Zoo. 140: 415-440.
Gepp, E. S., 1904. Chinese Marine Algae Jour. Bot. 42: 161-165.
Grubb, V. M., 1932. Marine Algae of Korea and China, with Notes on the Distribution of Chinese Marine Algae. Jour. Bot. 70: 213-219, 245-251.
Hartley, D., 1954. Food in England. Macdonald & Co. London.
Haug, A., and Larsen, B., 1955. Carotene Content of some Norwegian Seaweeds, and Observations on the Breakdown of Carotene in Seaweeds and Seaweed Meal. Second Internation Seaweed Symposium (Proceedings published by Pergamon Press, London 1956) pp. 16-22.
Hendrick, J., 1916. The Value of Seaweeds as Raw Materials for Chemical Industry. J. Soc. Chem. Indust. 35: 565-74.
Johnston, H. W. , 1965. The Biological and Economic Importance of Algae, Part I. Tuatara 13 (2): 90-114.
Kirby, R. H. , 1950. Seaweeds in Commerce Part I. Colonial Plant and Animal Products Vol. I (3): 183-216.
—— 1951. Seaweeds in Commerce Part III. Colonial Plant and Animal Products Vol. II (1): 1-22.
Lombard, P. E., 1962. Seaweeds in the Ration of Growing Baconers. Sth. Afric. Jour. Agric. Sci. 5 (3): 373-380.
Lundin, H., and Erickson, L.-E., 1955. On the occurrence of vitamins in Marine Algae. Second International Seaweed Symposium. (Proceedings published by Pergamon Press, London 1956)) pp. 39-43.
Marshall, S. M., and Orr, A. P., 1951. ‘The Artificial Culture of Marine Algae’ in Seaweed Utilization by L. Newton, see below.
Mautner, H. G., 1954. The Chemistry of Brown Algae. Econ. Bot. 8: 174-192.
Meeuse, B. J. D., 1962. ‘Storage Products’ in Physiology and Biochemistry of Algae. Editor R. A. Lewin, Academic Press.
Miller, C. D., 1927. Food Values of Poi. Taro and Limu. Bernice P. Bishop Museum Bulletin 37.
Ministry of Agriculture (China), Editor. 1960. People's Communes. Foreign Languages Press, Peking.
Newton, L. , 1951. Seaweed Utilisation. Sampson Low, London.
—— 1963. ‘Uses of Seaweeds’ in Vistas in Botany, Vol. 2, Applied Botany. Pergamon Press, London.
Nisizawa, K. , 1938. Physiological Studies on Laminarin and Mannitol of Brown Algae. 1. Diurnal Variation of Their Contents in Eisenia bicyclis. Sci. Repts. Rokyo Kyoiku Bunrika Daigahu Sech. B3 289-301.
—— 1939. J. Chem, Soc. Jap. 60: 1020 — Okamura, K., 1933. Uses of Algae in Japan. Proc. 5th Pacific Sci. Congress IV: 3153-3161.
Provasoli, L., 1963. ‘Organic Regulation of Phytoplankton Fertility’ in The Sea, Volume 2. Editor M. N. Hill . Interscience Publishers, London.
Reischauer, E. O., 1955. Ennin's Travels in T'ang China. Ronald Press Co., New York.
Round, F. E., 1965. The Biology of the Algae. Arnold Ltd., London.
Saiki, T., 1906. The Digestibility and Utilization of some Polysaccharide Carbohydrates Derived from Lichens and Marine Algae. J. Biol. Chem. 2: 251-265.
Spector, W. S., Editor, 1956. Handbook of Biological Data. Saunders, Philadelphia.
Suto, S., 1955. Seaweed Production and Phycological Research in Japan. 2nd International Seaweed Symposium (Proceedings published by Pergamon Press, London 1956).
Tiffany, L. H., 1958. Algae the Grass of Many Waters. Charles C. Thomas, Illinois U.S.A. 2nd. Edition.
Tseng, C. K., and Tang, P. S., 1936. On the Occurrence of Two Laminariaceous Plants on China Coast with a Note on Their Iodine Content. Lingnan Science Jour. 15 (2): 214-224.
Wilson, S. H., and Fieldes, M., 1941. Studies in spectographic analysis II. Minor Elements in a Seaweed ( Macrocystis pyrifera ). N. Z. Jour. Sci. Tech. 23B: 47-48.
Zaneveld, V. S., 1940. Economic Marine Algae of Tropical South and East Asia and their utilization. Indo-Pacific Fisheries Council Special Publication No. 3. Bangkok: pp. 1-55.
Home | Advanced Search | About | Help
© 2016 Victoria University of Wellington | Contact us | Conditions of use
- Bihar Board
SRM University
Bseb 12th result.
- Bihar Board Result 2024
- UP Board Result 2024
- CBSE Board Result 2024
- MP Board Result 2024
- Rajasthan Board Result 2024
- Shiv Khera Special
- Education News
- Web Stories
- Current Affairs
- नए भारत का नया उत्तर प्रदेश
- School & Boards
- College Admission
- Govt Jobs Alert & Prep
- GK & Aptitude
- general knowledge
- GK for Exams
What is the Economic Importance of Algae?
In this article, we have given a short note on the economic importance of the algae along with their typology and classification, which is very useful for competitive examinations like upsc-prelims, ssc, state services, nda, cds, and railways, etc..
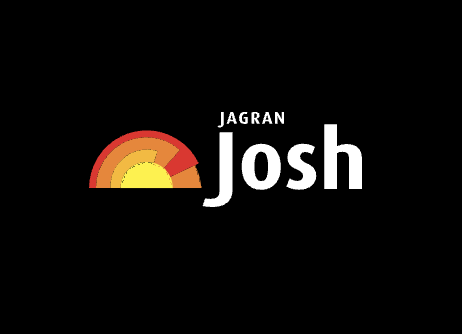
The term "algae" covers many different organisms capable of producing oxygen through photosynthesis. They belong to the kingdom of Protista and occur in a variety of forms and sizes. They can exist as single, microscopic cells; they can be macroscopic and multicellular; live in colonies, or take on a leafy appearance as in the case of seaweeds such as giant kelp.
Types of Algae
The habitat distribution of the Algae can be determined by their pigment. Although the majority of the Algae are found in aquatic habitats that might be in freshwater or marine water, only a few species are found in extreme conditions like snow, ice, or hot spring. There are mainly four types of Algae which are discussed below:
1. Blue-Green Algae: They are also known as Cyanobacteria . They appear in blue-green colour and contain chlorophyll 'a', 'b', and phycobilins .
2. Green Algae: They belong to the family of phylum Chlorophyta and contain chlorophyll 'a', 'b', carotenoids, and xanthophylls . They are unicellular and multicellular. They mostly abide in freshwater environments, although a few species can be found in the ocean.
3. Red Algae: They belong to the family of Rhodophyta and contain chlorophyll 'a',’d’, carotenoids, xanthophylls, and phycobilins . They are used as a stabilizer in milk products.
4. Brown Algae: They belong to the family of Phaeophyceae , and contain chlorophyll 'a', 'c' , and fucoxanthin pigment. They are used in order to stabilize, thicken, and modify various food products.
They are categorized as aquatic (planktonic, benthic, marine, freshwater, lentic, lotic), terrestrial, aerial (subaerial), lithophytic, halophytic (or euryhaline), psammon, thermophilic, cryophilic, epibiont (epiphytic, epizoic), endosymbiont (endophytic, endozoic), parasitic, calcifilic or lichenic (phycobiont).
What is Eutrophication?
Economic importance of Algae
These eukaryotic marine organisms have no roots, flowers, and stem. It plays important role in alkaline reclaiming which is used as a soil binding agent. They are economically important in a variety of ways which are discussed below:
1. Food: Algae are a healthy source of carbohydrates, fats, proteins, and vitamins A, B, C, and E as well as the minerals like iron, potassium, magnesium, calcium, manganese, and zinc . Hence, people of countries like Ireland, Scotland, Sweden, Norway, North and South America, France, Germany, Japan, and China uses it as a food ingredient.
2. Fodder: Algae are also used as fodder to feed livestock such as cattle and chickens. is utilized as a grain in different nations, including the northern European nations of Denmark, Sweden, Norway, etc.
3. Pisciculture: In fish farming, Algae plays a very important role because it helps in the production process. Fish used plankton and zooplankton as food. It helps in maintaining the health of the marine ecosystem because algae are naturally absorbent of carbon dioxide and also provide oxygen to the water.
4. Fertilizer: Algae are rich in minerals and vitamins. So they also used as liquid fertilizer which helps in the repairing level of nitrogen present in the soil.
5. Reclaiming Alkaline: Blue-Green Algae helps in the reduction of a high concentration of alkalinity in the soil.
6. Binding Agent: Algae act as binding agents against natural processes such as erosion.
7. Biological indicator: Algae are very sensitive. If there is a slight change in the environment their pigments changes or might get died. Water pollution is checked with the help of Algae like Euglena and Chlorella .
Get here current GK and GK quiz questions in English and Hindi for India , World, Sports and Competitive exam preparation. Download the Jagran Josh Current Affairs App .
- Where are most algae found? + Most species of algae live in the sea, in lakes, or in ponds. Some single-celled green algae live in moist conditions on land, such as on tree trunks, on the surface of the soil, or on damp brickwork. Others live inside lichens.
- What is the major difference between plants and algae? + Algae may be unicellular, colonial, or multi-cellular. Plants, on the other hand, are only multi-cellular.
- How do you classify algae? + There is three main Algae classification: Chlorophyceae – These are called green algae Phaeophyceae – Also called brown algae Rhodophyceae – They are the red algae
- What do algae have in common with plants? + Plant-like protists are called algae. They include single-celled diatoms and multicellular seaweed. Like plants, algae contain chlorophyll and make food by photosynthesis.
- IPL Schedule 2024
- Bihar Diwas
- IPL Opening Ceremony 2024
- Holi 2024 Date
- IPL 2024 Points Table
- Lok Sabha Election Date 2024
- IPL Channel Number List 2024
- BJP Candidate List 2024
- CSK vs RCB Head to Head
- Bihar Board 12th Result 2024
Latest Education News
Bihar Board 12th Result 2024: Check बिहार बोर्ड इंटर रिजल्ट, Official Link at biharboardonline.bihar.gov.in
Bihar Board Result 2024 Class 12 Link: List of Direct Official Sites to Check BSEB Inter Result Online
Bihar Board 12th Result 2024 OUT Live Updates: BSEB Inter Result Declared; Over 5 Lakh Students Got 1st Division, Check Pass Percentage, Toppers List
Optical Illusion: Find the hidden owl in the picture in 10 seconds!
Government Exams Deferred Due to Lok Sabha Elections 2024: List of UPSC, State PSC, SSC, and Bank Exams Postponed
CGPSC State Service Prelims Result 2024 Out at psc.cg.gov.in: 3597 Qualify for mains, Here's download link
WBPSC Food SI Question Paper 2024: Direct Download Link to Shift-Wise Papers
CBSE Class 12 Accountancy Answer Key 2024 and Question Papers, Download PDF All SETs
Bihar Sakshamta Pariksha Result 2024: Where and How to Check the Merit List
How to Check Voter Application Status?
Bihar Board 12 Vocational Result 2024: BSEB Class 12th Vocational Result Date and Time at biharboardonline.bihar.gov.in
BTEUP Result 2024: यूपी पॉलिटेक्निक विषम सेमेस्टर परिणाम bteup.ac.in पर, इस लिंक से करें चेक
Bihar Board 10th Result 2024 Likely by March 30? BSEB Class 12 Results Out
Bihar Sakshamta Pariksha Result 2024: जानें कैसे चेक करें बिहार सक्षमता परीक्षा रिजल्ट
Bihar Board 12th Result Analysis 2024: 87.21% Passed, Girls Outshine Boys
Find 3 differences between the fish in the sea pictures in 14 seconds!
Calicut University Result 2024 OUT at results.uoc.ac.in: Direct Link to Download UG, PG Marksheet
BSEB Bihar Board 12th Result Analysis 2024: बिहार बोर्ड 12वीं परीक्षा में 87.21% पास, लड़कियों ने मारी बाजी
biharboardonline.bihar.gov.in BSEB Result 2024 Released: Check Bihar Board 12th Result Online with Roll Number and Roll Code
Bihar Board 12th Toppers 2024 Revealed: Tushar in Arts, Priya in Commerce, and Mrityunjay Kumar Tops in Science Stream; Check Full List Here
Write a note on economic importance of algae and gymnosperms.
The economic importance of algae: (i) many species of algae are used as food. chlorella and spirulina are a rich source of proteins. (ii) agar is obtained from gelidium algae . it is used in jellies, ice-cream preparation, and laboratory culture media. (iii) carrageenin is obtained from red algae. it is used in chocolates, paints, and toothpaste manufacturing. it is also used as medicine for treating worm infection. the economic importance of gymnosperms: (i) an anti-cancer drug, taxol is obtained from taxus. (ii) ephedrine is obtained from ephedra. it is used for treating asthma and bronchitis. (iii) many species of gymnosperms are used as food. (iv) p ine and cedar act as sources of the soft wood that is used for construction and packing..
Talk to our experts
1800-120-456-456
Economic Importance of Algae
- Economic Importance Of Algae
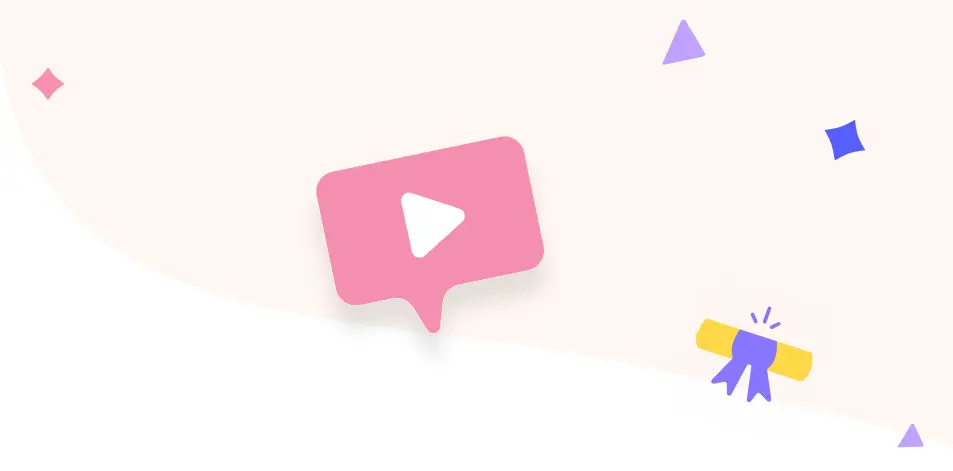

What is Algae?
Group of aquatic organisms belonging to the kingdom of Protista and capable of photosynthesis are referred to as algae. They can be found in a vast array of sizes and shapes. Some instances of algae that we encounter in our daily lives are seaweeds, algal bloom, and pond scums.
Algae are a diverse group of organisms that include mainly eukaryotes. Additionally, these organisms can be unicellular or multicellular. These unique features are responsible for some significant economic importance of Algae.
Apart from discussing the biological and economic importance of algae, we will also discuss the different types of algae.
What are the Economic Importance of Algae?
Because of their unique features, algae are of immense importance to human beings and nature alike.
Some of the Uses of Algae are –
It has been observed that Algae are economically important in a variety of ways. For instance, the natural substance can be used as a food source, fodder, fish farming, and as fertilizer. It also plays a key role in alkaline reclaiming and can be used as a soil binding agent as well as can be used in a variety of commercial products.
Fish Culture- Some fishes such as Tilapia mossambica feed on certain types of algae. Growing those varieties of algae has successfully facilitated fish culture in India.
Treatment of Sewage Plant- Algae has proved to be very useful in the treatment of sewage plants. It is because they produce oxygen through the process of photosynthesis, which helps in the rapid decomposition of sewage.
Used As Food for Humans- One of the most crucial economic importance of algae is that some species have been used as food for a long time in some parts of the world. Algae are rich in nutrients such as minerals, proteins, essential vitamins A, B, C, E, etc.
For instance, in Japan, a food item called ‘kombu’ is made from laminaria algae. Other varieties such as Porphyra and monostroma are also used to develop food items. A type of seaweed called the Irish moss or carragheen was used as an ingredient to make blancmanges.
Cattle Fodder- Algae species like Laminaria saccharina, Pelvetia, Ascophyllum, etc. are also useful as fodder for cattle in countries like Iceland and Scandinavia.
Natural Soil Fertilizers- It is used as a bio-fertilizer for crops since it can improve the nitrogen content of the soil. Another agricultural importance of algae is that it also enhances soil’s capacity to hold water. Blue-green algae or cyanobacteria are commonly used for fertilization.
Medicinal Purposes- Apart from food, algae have also been used for medicinal purposes by humans for a long time. Algae varieties like sargassum and Laminariales were used to treat conditions like goiter and glandular complications in many eastern countries. The component agar which was used as a laxative to treat stomach disorders is extracted from algae called agarphytes, gelidium, pterocladia, etc. Furthermore, research is being carried out regarding other medicinal properties of ingredients in algae.
Features of Algae
Listed below are some of the general features of algae:
The general features or characteristics of algae are they don’t have steam, roots, or leaves but also have chlorophyll and other pigments for carrying out photosynthesis. Most algae are known as photoautotrophic which carry photosynthesis. In addition to this if we consider the feature of Reproduction in algae then this process occurs in both asexual and sexual forms.
Some Interesting Features of Algae Have Been Discussed Below-
The majority of algae discovered till now are aquatic. They can be found in several habitats such as freshwater, saltwater, moist rocks, soil, and so on. Nonetheless, some can survive on surfaces outside water such as tree trunks, snowbanks, hot springs, etc.
Algae, like plants, are capable of photosynthesis which means they are primary producers in an aquatic environment.
Reproduction in algae can be vegetative, sexual, asexual, or a combination of both. Vegetative reproduction includes fragmentation, cell division, or fission, whereas asexual reproduction refers to the formation of naked or newly walled spores.
Sexual reproduction in algae is regulated by several environmental factors such as availability of inorganic nutrients, temperature, salinity. When these factors become unfavorable, it induces sexual reproduction.
One of the main differences between algae and plants is that the former does not have connective vascular tissues which facilitate the transportation of water and minerals, like the latter. Hence, algae absorb water via their cells and do not have structures like roots, leaves, and stem-like plants
Effects of Algae
Volvocales, Chlorococcales, Myxophyceae, and several others occur in water in great numbers which color the whole water either green or blue-green and cause the death of fishes.
Algal blooms can reduce the ability of fish and other aquatic life to find food and can cause entire populations to leave an area or even die. Harmful algal blooms cause thick, green muck that impacts clear water, recreation, businesses, and property values.
Types of Algae
It is known that Algae belong to the kingdom of Protista and are also known as simple photosynthetic organisms. Hence; Based on the occurrence of pigments and food reserves, algae are classified into different types, namely blue-green algae (BGA), green algae, red algae, and brown algae.
Several different types of Algae are found in nature. Some of these can be classified based on their color. These are–
Rhodophyta (Red algae).
Phaeophyta (Brown algae).
Chlorophyta (Green algae).
Pyrrophyta (Fire algae).
Chrysophyta (Golden brown algae).
Euglenophyta (euglenoids).
Xanthophyta (yellow-green algae).
Rhodophyta (Red Algae):
Rhodophyta, commonly known as red algae, are characterized by their red pigmentation due to the presence of phycoerythrin pigment.
These algae are predominantly marine, often found in deeper waters, and play a significant ecological role in coral reef ecosystems.
Red algae have diverse forms, ranging from filamentous to branched structures, and are known for their economic importance in industries such as agar production.
Examples: Porphyra, Gelidium, Corallina
Phaeophyta (Brown Algae):
Phaeophyta, or brown algae, are multicellular seaweeds characterized by their brownish coloration due to the presence of fucoxanthin pigment.
These algae are primarily marine and are commonly found in cooler coastal waters, where they form large underwater forests known as kelp forests.
Brown algae have complex structures and are economically valuable for their use in food products, fertilizers, and even in the cosmetic industry.
Examples: Macrocystis, Sargassum, Laminaria
Chlorophyta (Green Algae):
Chlorophyta, or green algae, are a diverse group of photosynthetic organisms that share many characteristics with land plants.
These algae can be found in various aquatic environments, including freshwater, marine habitats, and even in damp terrestrial habitats.
Green algae are important as primary producers in aquatic ecosystems and serve as model organisms for studying photosynthesis and cell biology.
Examples: Chlamydomonas, Volvox, Spirogyra
Pyrrophyta (Fire Algae):
Pyrrophyta, commonly known as fire algae or dinoflagellates, are single-celled organisms that are often bioluminescent, giving the appearance of glowing water at night.
These algae can be found in both freshwater and marine environments and play crucial roles in marine food webs as both primary producers and consumers.
Some species of dinoflagellates can produce harmful algal blooms (HABs) that release toxins, posing risks to marine life and human health.
Examples: Noctiluca, Ceratium, Gymnodinium
Chrysophyta (Golden brown Algae):
Chrysophyta, or golden brown algae, are a diverse group of photosynthetic protists known for their characteristic golden-brown coloration.
These algae are primarily freshwater organisms, although some species can be found in marine and soil habitats.
Golden brown algae are important contributors to freshwater ecosystems as primary producers and are also utilized in research as model organisms for studying ecological and evolutionary processes.
Examples: Diatoms, Synura, Mallomonas
Euglenophyta (Euglenoids):
Euglenophyta, or euglenoids, are a group of single-celled organisms that are characterized by their unique motility and the presence of a pellicle, a proteinaceous cell covering.
These organisms are primarily found in freshwater habitats, where they can be both autotrophic and heterotrophic, depending on environmental conditions.
Euglenoids have a diverse range of ecological roles, serving as primary producers in some environments and as consumers in others, and are of interest in research due to their evolutionary significance.
Examples: Euglena, Phacus, Euglenopsis
Xanthophyta (Yellow-green Algae):
Xanthophyta, or yellow-green algae, are a group of photosynthetic protists characterized by their yellow-green pigmentation, which is due to the presence of carotenoid pigments.
These algae are primarily freshwater organisms, often found in stagnant or nutrient-rich waters, although some species can also be found in marine habitats.
Yellow-green algae play roles as primary producers and are important contributors to the ecology of freshwater habitats, where they serve as food sources for various organisms.
Examples: Vaucheria, Tribonema, Botrydium
Harmful Effects of Algae
As we saw in the previous section, there are several economic importance of algae. However, not all algae are useful, some species can be quite toxic to the environment. The growth of algal blooms in large quantities can disrupt the balance of aquatic habitat and result in toxin production. Drinking water from an algae-infested water body can lead to casualties, especially for animals and cattle. Apart from drinking, it cannot be used for recreational or agricultural purposes.
While preparing for NEET , you should focus on understanding the underlying concepts of each topic, such as the economic importance of Algae instead of memorizing terms and definitions.
Refer to specialized study guides for NEET, which will enable you to gain more clarity on a topic. It will allow you to retain more information on the day of the examination. Furthermore, divide your time equally between physics, chemistry, and biology.
Apart from a revision of concepts like the economic importance of algae botany, sit for as many mock tests as is possible. It will help you improve your speed as well as accuracy.
Difference Between Plants and Algae
Here are the few differences between plants and algae:
The economic importance of algae cannot be overstated. From being valuable sources of food and oxygen production to serving as key players in various industries such as pharmaceuticals, cosmetics, and biofuels, algae plays a crucial role in both ecological balance and human welfare. As we continue to explore and harness the potential of algae, it is evident that these simple organisms hold immense promise for sustainable development and innovative solutions to pressing global challenges. Therefore, further research and investment in algae-based technologies are essential to unlock their full economic potential and pave the way for a greener, more sustainable future.
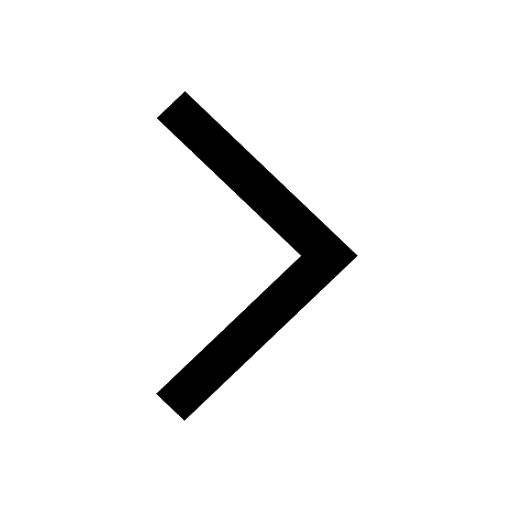
FAQs on Economic Importance of Algae
1. Why are Algae Not Considered to Be a Part of Kingdom Plantae?
Algae do not have stems, roots, and leaves like plants. As a result, they don’t possess vascular tissues that facilitate the transport of water and nutrients from roots to leaves. However, these unique features are responsible for the economic importance of Algae.
2. What are the Various Modes of Asexual Reproduction Seen in Algae?
The different modes of asexual reproduction seen in algae are zoospores, aplanospores, tetraspores, akinetes, exospores, endospores.
3. What are Some of the Features of Chlorophyta or Green Algae?
These types of algae are mostly found in freshwater environments. They contain chloroplasts and can carry out photosynthesis. The cell walls of green algae are composed of cellulose. Examples of sea algae are sea lettuce, dead man’s fingers, horsehair algae.
4. What Causes Algae?
Causes for algae in ponds and lakes are many; however, some are light exposure levels, pollution, turbidity, movement of water, temperature, water chemistry, ecosystem disturbance, availability of nutrients, etc.
5. How can algae be harmful to humans?
Direct skin contact with algal toxins can cause skin and eye irritation. Drinking algae-affected water or consuming food such as fish or shellfish containing toxins can cause gastroenteritis, which can induce vomiting, diarrhea, fevers, and headaches. These toxins may also affect the liver or nervous system.
6. Are Algae Plants Or Protists?
Algae are considered protists, plant-like protists a grab-bag category which are related organisms that are grouped on the basis of not being animals, plants, fungi, bacteria, or archaeans.
7. Can Algae cause disease?
Algae can cause human diseases by directly attacking human tissues, although the frequency is rare. Protothecosis, caused by the chloroplast-lacking green alga, Prototheca, can result in waterlogged skin lesions, in which the pathogen grows.
8. How can you tell if algae are toxic?
Toxic algae can look like foam, scum, or mats on the surface of the water said, Schmale. Other algae named cyanobacteria referred to as blue-green algae naturally occur in all freshwater ecosystems which release algal toxins that can be harmful to aquatic and human life.
9. What Are the Economic Importance of Algae?
The most important five economic importance of algae include:
Food: Some species of algae, such as nori and spirulina, are consumed by humans as food due to their high nutritional value.
Oxygen Production: Algae are primary producers that contribute a significant amount of oxygen to the atmosphere through photosynthesis.
Pharmaceuticals: Algae are a potential source of bioactive compounds used in the pharmaceutical industry for the development of drugs and medicines.
Cosmetics: Algal extracts are utilized in cosmetics and skincare products for their moisturizing, antioxidant, and anti-aging properties.
Biofuels: Certain species of algae can be cultivated for the production of biofuels such as biodiesel and bioethanol, offering a renewable and sustainable alternative to fossil fuels.
10. What is the economic importance of algae and gymnosperm?
The economic importance of algae and gymnosperms lies in their diverse applications across various industries:
A. Food and Agriculture: Some algae species are consumed as food, while gymnosperms like pine trees provide timber and resin used in construction and furniture making.
B. Pharmaceuticals: Both algae and gymnosperms contain bioactive compounds with medicinal properties, serving as sources for drug development in the pharmaceutical industry.
C. Environmental Remediation: Algae and gymnosperms play crucial roles in carbon sequestration, soil stabilization, and water purification, contributing to ecosystem health and sustainability.
D. Bioenergy: Algae and gymnosperms are potential sources of biofuels, offering renewable and eco-friendly alternatives to fossil fuels.
E. Ornamental and Horticultural Use: Gymnosperms such as bonsai trees and algae like seaweed are cultivated for ornamental purposes and in horticulture.
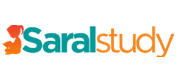
Write a note on economic importance of algae and gymnosperms.
Economic importance of algae
Algae have diverse economic uses. They perform half of the total carbon dioxide-fixation on earth by photosynthesis, acting as the primary producers in aquatic habitats.
(a) Food source: Many species of marine algae such as Porphyra, Sargassum, and Laminaria are edible. Chlorella and Spirulinaare rich in proteins. Thus, they are used as food supplements.
(b) Commercial importance: Agar is used in the preparation of jellies and ice-cream. It is obtained from Gelidium andGracilaria. Carrageenin is used as an emulsifier in chocolates, paints, and toothpastes. It is obtained from the red algae.
(c) Medicines: Many red algae such as Corallina are used in treating worm infections.
Economic importance of gymnosperms
(a) Construction purposes: Many conifers such as pine, cedar, etc., are sources of the soft wood used in construction and packing.
(b) Medicinal uses: An anticancer drug Taxol is obtained from Taxus. Many species of Ephedra produce ephedrine, which can be used in the treatment of asthma and bronchitis.
(c) Food source: The seeds of Pinus gerardiana (known as chilgoza) are edible.
(d) Source of resins: Resins are used commercially for manufacturing sealing waxes and water-proof paints. A type of resin known as turpentine is obtained from various species of Pinus.
Popular Questions of Class 11 Biology
- Q:- Describe briefly the four major groups of Protozoa.
- Q:- Why are living organisms classified?
- Q:- State two economically important uses of: (a) Heterotrophic bacteria (b) Archaebacteria
- Q:- What is the difference between direct and indirect development?
Define a taxon. Give some examples of taxa at different hierarchical levels.
- Q:- Describe the important properties of enzymes.
What are the steps involved in formation of a root nodule?
- Q:- How is a key helpful in the identification and classification of an organism?
- Q:- Illustrate the taxonomical hierarchy with suitable examples of a plant and an animal.
- Q:- What is stomatal apparatus? Explain the structure of stomata with a labelled diagram.
Recently Viewed Questions of Class 11 Biology
- Q:- Draw a standard ECG and explain the different segments in it.
Name two cell-organelles that are double membrane bound. What are the characteristics of these two organelles? State their functions and draw labelled diagrams of both.
- Q:- What is the significance of juxtaglomerular apparatus (JGA) in kidney function?
- Q:- What is G0 (quiescent phase) of cell cycle?
- Q:- Find out what do the terms algal bloom and red-tides signify.
List the hormones secreted by the following: (a) Hypothalamus (b) Pituitary (c) Thyroid (d) Parathyroid (e) Adrenal (f) Pancreas (g) Testis (h) Ovary (i) Thymus (j) Atrium (k) Kidney (l) G-I Tract
- Q:- Match the items of column I with those of column II: Column I Column II (a) Ammonotelism (i) Birds (b) Bowmans capsule (ii) Water reabsorption (c) Micturition (iii) Bony fish (d) Uricotelism (iv) Urinary bladder (e) ADH (v) Renal tubule
- Q:- Can you attempt building models of biomolecules using commercially available atomic models (Ball and Stick models).
- Q:- Both a short day plant and a long day plant can flower simultaneously in a given place. Explain.
- Q:- Draw the labelled diagram of the following: (i) Gram seed (ii) V.S. of maize seed
10 Comment(s) on this Question
This answer help us in many ways. So very very thanku to you for giving this easy and short answer
Thanks for this amazing answer,,,ðð
Ur answer r too big try to write small answer which is easy to learn and write
Very clear and thanks
Try to write short answer which helpfull to learn easily sir this answer is right but it is so long .....
I want uses of algae
Write a Comment:
- The Living World
- Biological Classification
- Plant Kingdom
- Animal Kingdom
- Morphology of Flowering Plants
- Anatomy of Flowering Plants
- Structural Organisation in Animals
- Cell: The Unit of Life
- Biomolecules
- Cell cycle and Cell Division
- Transport in Plants
- Mineral Nutirtion
- Photosynthesis in Higher Plants
- Respiration in Plants
- Plant Growth and Development
- Digestion and Absorption
- Breathing and Exchange of Gasses
- Body Fluids and Circulation
- Excretory products and their Elimination
- Locomotion and Movement
- Neural Control and Coordination
- Chemical Coordination and Integration
NCERT Solutions
- NCERT Solutions for Class 12
- NCERT Solutions for Class 11
- NCERT Solutions for Class 10
- NCERT Solutions for Class 9
- NCERT Solutions for Class 8
- NCERT Solutions for Class 7
- NCERT Solutions for Class 6
Quick Links
- NCERT Sample Papers
- NCERT Books
- CBSE Syllabus
- Scholarship for Students
- NCERT Exemplar
- CBSE Topper Answer Sheets
- CBSE Board Paper
- Multiplication Tables 2 to 30
- Latest Blog
- © 2024 SaralStudy
- Privacy Policy
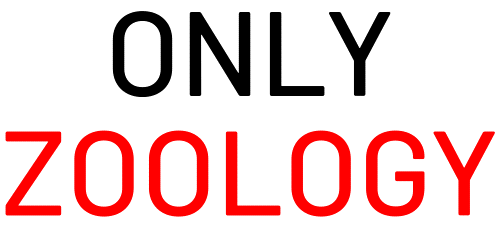
12 Economic Importance of Algae (With examples & FAQs)
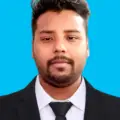
From tiny, single-celled microalgae to massive seaweeds, algae are a diverse collection of aquatic animals. They can be found in many different locations, such as freshwater and saltwater bodies, as well as moist areas of land.
As primary producers that use photosynthesis to create organic matter from carbon dioxide and sunshine, algae are essential to the functioning of the Earth’s ecosystem. This process, which forms the foundation of the food chain for many aquatic creatures, is crucial for maintaining life on Earth.
A few kinds of algae are also utilized commercially. For instance, certain microalgae species are utilized to produce biofuels, while others are used to make medications and dietary supplements.
However, some types of algae can also cause harmful algal blooms, which can lead to the production of toxins that can be harmful to humans and wildlife. These blooms can also lead to oxygen depletion in the water, which can have negative impacts on aquatic ecosystems.
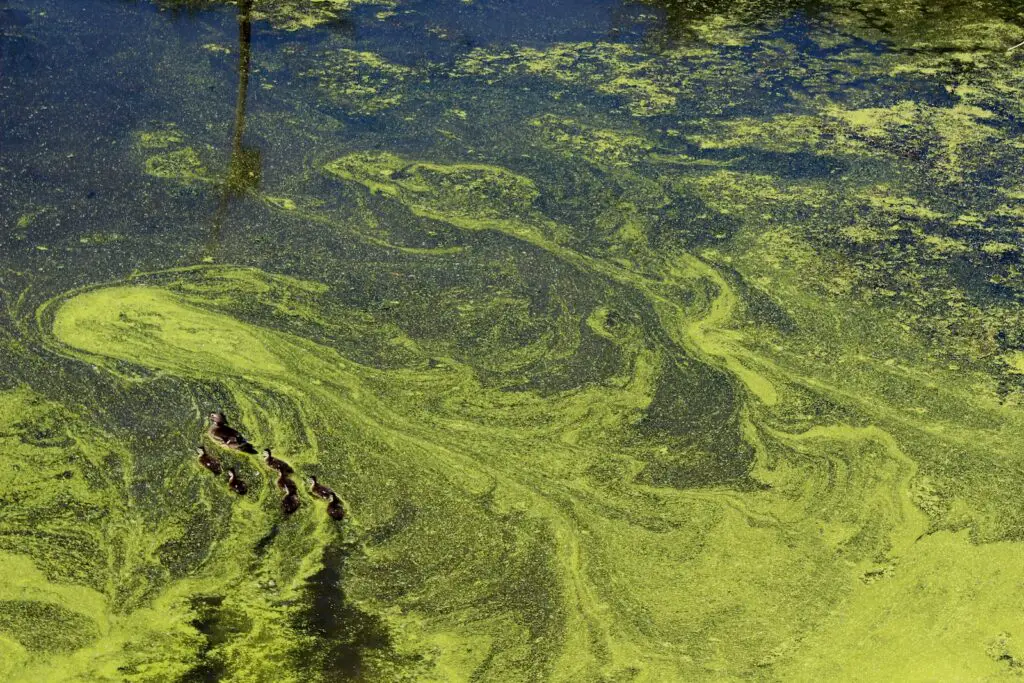
Here are the 12 Economic Importance of Alage with Examples. Check These Out :
1. algae as a source of biofuels:.
Since they can be cultivated fast and effectively with little land and other resources, algae are a viable source of biofuels. They contain a lot of oil, which can be recovered and used to make biodiesel or other fuels.
A sustainable substitute for fossil fuels, algae-based biofuels have the potential to lower greenhouse gas emissions.
Algae-based aviation fuel is a type of biofuel made from algae. Virgin Atlantic Airways carried out a test flight in 2012 using a mixture of 20% jet fuel made from algae and 80% regular jet fuel.
Please enable JavaScript
The strain of algae used to make the algae-based fuel was genetically altered to produce more oil. The viability of employing algae-based biofuels in commercial aircraft was proved by this test flight.
2. Algae as a source of nutritional supplements:
Because they are a good source of vitamins, minerals, and other nutrients, algae are often employed as a source of dietary supplements. In health food stores, several kinds of algae, like spirulina and chlorella, are offered as well-liked nutritional supplements.
A blue-green algae with a high protein, vitamin, and mineral content is spirulina. It is frequently consumed as a dietary supplement to promote immune system health and increase energy.
A green algae that is high in nutrients and protein is called chlorella. It is frequently used to enhance digestive health and as a cleansing agent.
Because of their great nutritional content, spirulina and chlorella are both regarded as superfoods. They can be found in many different formulations, including as pills, capsules, and powders. Because more people are interested in natural, plant-based sources of nutrition, the market for nutritional supplements based on algae is anticipated to continue expanding.
3. Algae in the aquaculture industry:
The aquaculture sector, which comprises the rearing of fish, shellfish, and other aquatic animals, includes algae as a significant component. Many different aquatic species, such as shrimp, oysters, and clams, eat algae as food. Certain varieties of algae, like brown algae, can offer specific fish and shellfish species with a natural habitat.
There are many benefits to using algae as a feed source in aquaculture. Algae can be farmed quickly and responsibly, and they are a natural food supply. They also contain a variety of nutrients that are crucial for the development and well-being of aquatic animals, such as protein, carbs, and vitamins. In the upcoming years, as the demand for seafood keeps rising, more algae will likely be used as a feed source in aquaculture.
4. Algae in the cosmetic industry:
As a result of their capacity to hydrate and nourish the skin, algae are likewise utilised in the cosmetic business. Amino acids, fatty acids, and polysaccharides are just a few of the components that algae possess that are good for the skin. These substances may enhance skin suppleness, minimise the look of fine lines and wrinkles, and shield the skin from damaging elements.
Certain varieties of algae, such kelp, and spirulina, are added to cosmetics like serums, moisturizers, and face masks. As customers’ interest in natural and eco-friendly beauty products grows, algae-based skincare products are rising in popularity. To enhance the health and luster of hair, algae are often used in hair care products including shampoos and conditioners.
5. Algae in the food industry:
Algae have been used as a food source for ages, especially in Asia. In many places of the world today, algae are used as a source of food and food additives. Algae can be used to generate a range of food products, such as snacks, noodles, and beverages, and they are high in protein, fibre, and other nutrients.
Nori, a kind of seaweed used to make sushi, is an illustration of a food item made from algae. Agar, a gelatinous substance formed from red algae that is used as a gelling agent in many different food products, including jellies and candies, is another illustration. As consumers show a greater interest in plant-based diets and sustainable food sources, algae-based food products are predicted to grow in popularity.
6. Algae in the pharmaceutical industry:
Because of their capacity to create bioactive chemicals with potential therapeutic effects, algae are exploited in the pharmaceutical sector. These substances have the ability to treat a wide range of illnesses, such as viral infections, inflammation, and cancer. Many medications made from algae are already available on the market and more are being researched for a variety of ailments.
Spirulina extract, a natural supplement used to cure a number of illnesses, such as liver disease and allergies, is an illustration of an algae-based medication. Another such is carrageenan, a substance derived from red algae that thickens numerous pharmaceutical goods including topical lotions and cough syrups.
7. Algae in the wastewater treatment industry:
Because they can remove nutrients and contaminants from wastewater, algae are utilized in the wastewater treatment sector. Water-polluting substances like nitrogen and phosphorus can be eliminated by growing algae in wastewater. Algae can also be used to filter wastewater of heavy metals and other contaminants.
Algae may be used to remediate wastewater and offers a number of benefits. Algae may be cultivated using carbon dioxide and sunlight and are a sustainable and natural way to remediate wastewater. Moreover affordable, algae-based wastewater treatment systems provide biomass that may be used for a variety of projects, including the manufacture of biofuels.
The demand for environmentally friendly wastewater treatment techniques is predicted to expand, which will likely lead to an increase in the usage of algae in wastewater treatment.
8. Algae in the animal feed industry:
Animals of all kinds, including cattle and pets, eat algae as food. Animal meals made from algae are full of nutrients including protein, vitamins, and minerals and can help animals grow and stay healthy. Traditional animal feeds, which frequently depend on soybean or corn, are less sustainable than animal feeds based on algae.
Spirulina is an example of an algae-based animal feed that is added to fish and poultry diets as a supplement. Another illustration is chlorella, which is added to the feed of pigs and cows. The need for sustainable and plant-based animal products among consumers is predicted to increase the appeal of algae-based animal feeds.
9. Algae in the water purification industry:
Due to their capacity to reduce pollution and enhance water quality, algae are utilised in the water treatment sector. The removal of fertilisers, metals, and other contaminants from water can be accomplished by growing algae in sizable ponds or tanks. Algae can be used to purify water of dangerous germs and viruses.
Algae can be used to clean water and has several benefits. Water filtration systems based on algae are organic and environmentally friendly, and they may be used to clean both freshwater and ocean water. Systems for purifying water using algae are also economical and environmentally friendly. As the need for clean water sources rises, more algae will likely be used in water purification.
10. Algae in the carbon capture industry:
The ability of algae to absorb carbon dioxide from the atmosphere is being studied. Algae have the ability to carry out photosynthesis, which is the process by which they use light to change carbon dioxide into organic matter. Algae absorb carbon dioxide from the atmosphere as they expand, and this carbon can be harvested and used for a number of different things.
There are various benefits of using algae for carbon capture. Algae-based carbon capture systems are organic and environmentally friendly, and they don’t need expensive machinery or a lot of energy.
Systems that use algae to capture carbon can also yield useful byproducts like biofuels and animal feed. As the need for eco-friendly responses to climate change increases, it is anticipated that the development of algae-based carbon capture systems will continue.
11. Algae in the cosmetic industry:
Because they can enhance the health and appearance of skin, algae are utilised in the cosmetics business. Algae-based cosmetics can be used to hydrate and safeguard the skin because they are high in vitamins, minerals, and antioxidants. Cosmetics made from algae are also more environmentally friendly than conventional cosmetics, which frequently use artificial components.
Seaweed extract, which is used in numerous skin care products to increase skin elasticity and lessen wrinkles, is an illustration of an algae-based cosmetic product. Another illustration is the microalgae-derived substance alguronic acid, which is a common ingredient in anti-aging treatments.
Algae-based cosmetics are anticipated to grow in appeal as customer interest in organic and environmentally friendly beauty products increases.
12. Algae in the paper industry:
In the paper industry, algae are being investigated as a potential supply of paper. Paper made from algae is more environmentally friendly than paper made from wood pulp, which leads to deforestation and other environmental problems. Algae can be cultivated on non-arable soil, gathered, and processed to make paper.
Algae can be cultivated quickly, making it a resource that is highly renewable, which is one benefit of using it as a source for paper. Algal growth can be collected in a matter of weeks, unlike tree growth, which takes several years. Paper made from algae also has a lower environmental impact because it requires less energy and chemicals to process.
Paper products including writing paper, newsprint, and packing materials can all be made from algae. Algae-based paper may be created in a variety of colours and textures, making it appropriate for a variety of uses.
Although algae-based paper is still in its infancy, there has been a lot of research and advancement in this field. As the demand for environmentally friendly paper goods rises, more businesses are anticipated to start making paper products using algae as a base.
One example of a company that is producing algae-based paper products is AlgaePap, a startup based in Singapore. AlgaePap has developed a process for using algae to produce paper products that are sustainable, recyclable, and biodegradable. The company’s paper products are made using a combination of algae, plant fibers, and other natural materials.
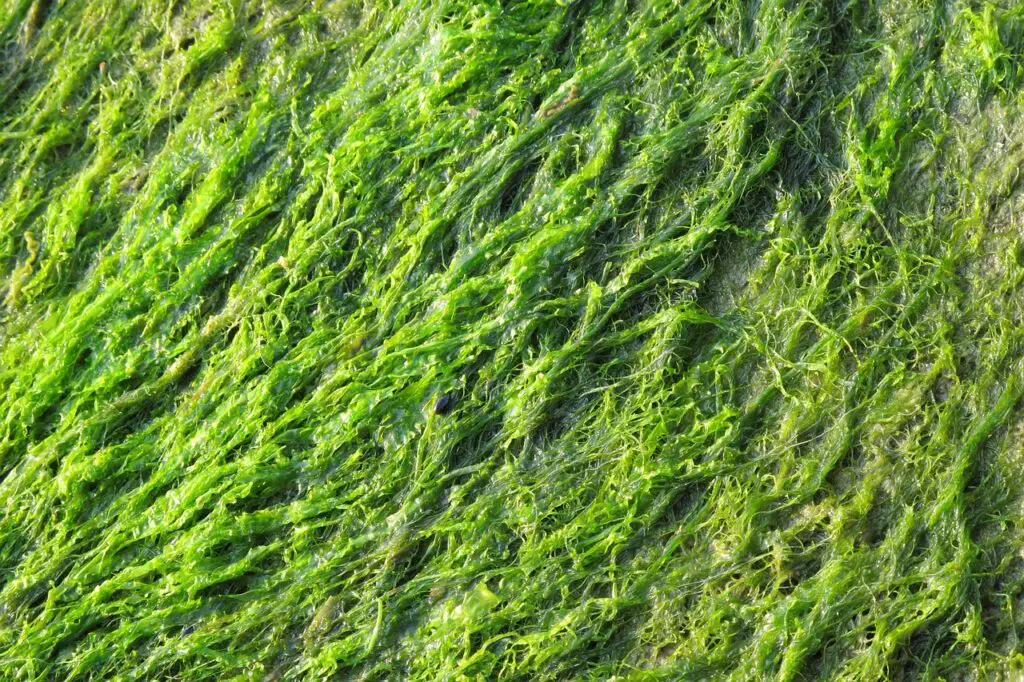
FAQs about the importance of Algae
What is the ecological importance of algae.
As the principal producers in aquatic ecosystems, algae serve as the foundation of the food web for a wide variety of aquatic creatures. By photosynthesis, they also play a significant part in the global carbon cycle by absorbing a lot of carbon dioxide.
Can algae be used as a source of food?
Indeed, a variety of algae species are edible and are eaten by people as dietary supplements, sushi, and other kinds of seaweed. In aquaculture, algae are also employed as a food source for fish and other aquatic creatures.
Are algae used in the production of biofuels?
Indeed, certain microalgae species can be utilised to make biofuels like biodiesel and bioethanol. These biofuels are viewed as a more environmentally responsible and sustainable substitute for conventional fossil fuels.
Can algae be harmful to the environment?
It is true that some types of algae can produce toxic algal blooms that can harm both people and wildlife by depleting the water’s oxygen supply. Nonetheless, some types of algae have valuable ecological and industrial use.
What is the importance of algae in the pharmaceutical industry?
A range of bioactive substances found in algae may be used therapeutically. As an illustration, substances isolated from specific species of algae have been demonstrated to have anti-inflammatory, antiviral, and antioxidant characteristics, and they are now being researched for their possible use in the creation of novel medications.
What is the role of algae in carbon sequestration?
Algae play a significant role in the Earth’s carbon cycle because they absorb a lot of carbon dioxide through photosynthesis. Moreover, some types of algae may store carbon as calcium carbonate, which can be used to lessen the consequences of ocean acidification.
How are algae used in wastewater treatment?
Since they can remove excess nutrients like nitrogen and phosphorus from wastewater, algae are employed in wastewater treatment. Using algae to break down contaminants and turn them into biomass is a method known as bioremediation.
Can algae be used to create bioplastics?
Indeed, some types of algae have polysaccharides that can be used to make plastics that degrade naturally. These bioplastics are thought to be more environmentally friendly and sustainable than conventional plastics, which come from non-renewable resources and disintegrate slowly.
What is the role of algae in soil health?
Because they help to create soil organic matter, algae are crucial to the health of the soil. This organic matter feeds plants with nutrients, enhances the soil’s ability to retain water, and encourages microbial activity in the ground.
How are algae used in cosmetics?
Because algae contain a lot of antioxidants, minerals, and vitamins, they are used in cosmetics. Several skincare products, such as face masks, moisturisers, and anti-aging lotions, contain algae extracts. In order to nourish and strengthen hair, algae extracts are often employed in hair care products.
MORE RELATED POSTS
- How Do Humans Use Cnidarians? – (List of 12 Importance of Cnidarians)
- Why are euglenas green? Is euglena a green algae?
- Biological, Economical, And Medicinal Importance of Sponges
- Biological, Economical, And Ecological Importance of Amoeba
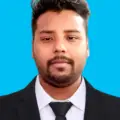
This post is written by Ronit Dey. Ronit Dey is a graduate in Zoology. Here, he has started sharing a lot of things that he has seen, learned, and researched so far related to Zoology. You can read more about here at the About page.

Write a note on the economic importance of algae?
Algae : algae include a large group of eukaryotic, photosynthetic life forms. habitat distribution is determined by their pigment. economic importance of algae: food source healthy source of carbohydrates, fats, proteins, and vitamins a, b, c, and e. used as food supplements. marine algae such as porphyra, sargassum, and laminaria. medicines algae like coralline is used to treat infections caused by worms. biological indicator algae like euglena and chlorella help in checking water pollution. pisciculture in fish farming, algae help in the production process..


IMAGES
VIDEO
COMMENTS
Algae is Used as Medicine. Industrial Utilization of Algae. Economic Importance # 1. Algae Constitute the Link of Food Chain: Both fresh and salt waters contain an enormous variety of algae which constitute the fundamental or primary link of many diverse food chains. Algae synthesize organic food stuffs, just as do the plants of the land.
Algae refers to a wide range of eukaryotic marine organisms, all of which engage in the process of photosynthesis. Ranging in size from unicellular microalgae to giant kelp, these aquatic plants are characterized by their lack of flowers, formal roots, leaves, or even stems. Algae are economically important in a variety of ways.
Brown algae (Phaeophyta) includes Kelps (Macrocystis, Laminaria) and Ascophyllum, Fucus, and Sargassum. Alginic Acid (Alginate) is a colloidal product used for thickening, suspending, stabilizing, emulsifying, gel-forming, or film-forming, as required. About half of the alginate produced is used for making ice cream and other dairy products, the rest is used in other products, including ...
The blue-green algae can be used to accomplish this cycle. Binding agent. Soil can be held together by algae. In protecting against natural processes such as erosion, the effectiveness of algae to help in healthy soil formation is important. Also Read: Examples of Green Algae
This chapter considers the economic importance of algae with particular reference to Australia and the value of mic-roalgae and microalgal products and the negative economic impacts of algal blooms, etc. Many algae are or significant economic value due to their biological role in ecosystems and as sources of commercially significant products, while others have an economic cost caused by their ...
Economic Importance of ALGAE 1. Food: Algae have been in use as human food for centuries in various parts of the world, including Scotland, Ireland, Norway, Sweden, France, Germany, North and South America, China, and Japan. Algae are taken in several ways according to the choice and taste of the people. They may be taken as a salad, cooked ...
The blue-greens are chiefly important in fresh-waters; they are responsible, for example, for the formation of extensive limestone deposits around hot springs and glaciers. The red algae are the most important calcareous algae of the seas; in particular, they play a significant role in the construction of coral reefs and islands.
Despite being a comparatively new branch of agriculture, algae production is often considered to be a solution to many food security-related problems, such as land scarcity, climate change, inefficient and unsustainable fertilizer usage, as well as associated nutrient leakage and water pollution. Algae can be cultivated independent of arable land and, especially in the case of many microalgae ...
The analysis of the food market as a dynamic socio-economic system, as well as consideration of the content and features of the market allowed the authors to identify the most important problems ...
The Biological and Economic Importance of Algae, Part 2. by H. W. Johnston. Botany Department, Victoria University of Wellington. In the First Article of this series (Johnston 1965), we dealt with the importance of algae as producers of organic matter—the fuel of the biological world. While this is of fundamental importance to Man, it is not ...
Solution 1. Economic importance of algae is as follows: The group Algae plays both economically beneficial as well as harmful roles. Beneficial importance : (i) People of coastal countries have been using sea weeds & certain other algae as source of food, e.g., Porphyra, Ulva, Laminaria, etc. (ii)Some algae are used as food for marine as well ...
Verified by Toppr. Algae have diverse economic uses. They perform half of the total carbon dioxide-fixation on earth by photosynthesis, acting as the primary producers in aquatic habitats. (a) Food source: Many species of marine algae such as Porphyra, Sargassum, and Laminaria are edible. Chlorella and Spirulina are rich in proteins.
Economic importance of Algae - definition. Algae are used as food as they are rich in carbohydrates, vitamins and few other inorganic substances. For example, Spirogyra is the chief source of food. Algae release lot of oxygen into water as a by-product of photosynthesis. This is the source of dissolved oxygen for aquatic organisms.
It plays important role in alkaline reclaiming which is used as a soil binding agent. They are economically important in a variety of ways which are discussed below: 1. Food: Algae are a healthy ...
In various ways, there is a huge economic importance of algae. The first example is that we can use the natural substance from algae as a good source of food, fodder, in fish farming, and also used as a fertiliser. Algae also plays a key role in the process of alkaline reclaiming which can be used as an agent known for soil binding, and it is ...
The economic importance of algae: (i) Many species of algae are used as food. Chlorella and Spirulina are a rich source of proteins. (ii) Agar is obtained from Gelidium algae. It is used in jellies, ice-cream preparation, and laboratory culture media. (iii) Carrageenin is obtained from red algae. It is used in chocolates, paints, and toothpaste ...
The most important five economic importance of algae include: Food: Some species of algae, such as nori and spirulina, are consumed by humans as food due to their high nutritional value. Oxygen Production: Algae are primary producers that contribute a significant amount of oxygen to the atmosphere through photosynthesis. Pharmaceuticals: Algae are a potential source of bioactive compounds used ...
Economic importance of algae. Algae have diverse economic uses. They perform half of the total carbon dioxide-fixation on earth by photosynthesis, acting as the primary producers in aquatic habitats. (a) Food source: Many species of marine algae such as Porphyra, Sargassum, and Laminaria are edible. Chlorella and Spirulinaare rich in proteins.
To enhance the health and luster of hair, algae are often used in hair care products including shampoos and conditioners. 5. Algae in the food industry: Algae have been used as a food source for ages, especially in Asia. In many places of the world today, algae are used as a source of food and food additives.
Q. Write a note on economic importance of algae and gymnosperms. Q. Algae have great economic importance to man because. Q. Describe the economic importance of algae with suitable examples. (any five) Q. Write a short note on Algae. View More.
Algae can be both beneficial and harmful to mankind. Algae are primarily producers of food in a very large ways and are used as food, fodder and manures. Many industrial products e.g., agar-agar carrageenin, iodine are obtained from algae. Algae can sometimes be harmful to man causing damage to ships, buildings and by causing diseases in plants ...
Handwritten notes 📝available.....Economic importance of algae ︎Useful importance ︎Harmful importance If you find any doubt then you can ask me in the co...
Bryophytes are of great ecological importance due to following reasons: (a) Pioneer of the land plants. Bryophytes are pioneer of the land plants because they are the first plants to grow and colonize the barren rocks and lands. ADVERTISEMENTS: (b) Soil erosion. Bryophytes prevent soil erosion.