- University of Notre Dame
- Blog Network

Notes on Teaching and Learning
Shutting Down on 1/6/2022 – New Posts are at Learning.ND.edu
Problem-Based and Inquiry-Based Learning: What’s the difference?
Sep 30th, 2019 by Kathryn Mulholland
“If your goal is to engage students in critical thinking… you need to present interesting challenges to solve, rather than simply explaining how other smart people have already solved those challenges.” – Therese Huston
Problem-Based Learning (PBL) and Inquiry-Based Learning (IBL) are both student-centered teaching pedagogies that encourage active learning and critical thinking through investigation. Both methods offer students interesting problems to consider. And research shows that both PBL and IBL are effective models of learning.
So, what’s the difference between the two?
According to Banchi and Bell [4], there are four different levels of inquiry.
- Confirmation Inquiry: Students confirm a principle through an activity when the results are known in advance.
- Structured Inquiry: Students investigate a teacher-presented question through a prescribed procedure.
- Guided Inquiry: Students investigate a teacher-presented question using student designed or selected procedures.
- Open Inquiry: Students investigate questions that are student formulated through student designed or selected procedures.
Most academics define Inquiry-Based-Learning as a pedagogy that is based on one of these levels. So IBL can be as methodical as guiding students through a procedure to discover a known result or as free-form as encouraging students to formulate original questions. For example, in a Physics laboratory, suppose the topic is Newton’s Second Law of Motion. The lab instructions could define a procedure to record the mass and impact force of various objects. Multiplying the mass by the acceleration due to gravity, the students should recover the force they recorded, thus confirming Newton’s Second Law.
Problem-Based-Learning can be classified as guided inquiry where the teacher-presented question is an unsolved, real-world problem. For example, in a Middle Eastern Studies course, the main problem posed by the instructor could be “Propose a solution to the Israeli–Palestinian conflict.” This question will motivate the study of the history of the region, the theological differences between Judaism and Islam, and current events. At the end of the semester, students would be expected to present and justify their solution.
Therefore, using the definition above, PBL is a type of IBL .
PBL is great because it motivates course content and maximizes learning via investigation, explanation, and resolution of real and meaningful problems. At any level, inquiry can be an effective method of learning because it is student-centered and encourages the development of practical skills and higher-level thinking.
As you plan for your next class, I invite you to reflect on your method of content delivery. Is it motivated? How? Would your students benefit from a day based on inquiry?
References.
- Inquiry Based Learning. University of Notre Dame Notes on Teaching and Learning. https://sites.nd.edu/kaneb/2014/11/10/inquiry-based-learning/ .
- Problem-Based Learning. Cornell University Center for Teaching Innovation . https://teaching.cornell.edu/teaching-resources/engaging-students/problem-based-learning .
- Hmelo-Silver, Cindy E.; Duncan, Ravit Golan; Chinn, Clark A. (2007). “Scaffolding and Achievement in Problem-Based and Inquiry Learning: A Response to Kirschner, Sweller, and Clark (2006)”. Educational Psychologi st. 42 (2): 99–107. doi : 10.1080/00461520701263368 .
- Banchi, H., & Bell R. (2008). The many levels of inquiry. Science and Children.
Posted in Uncategorized
Comments are closed.
If you have questions about a topic related to teaching or learning, please email [email protected] or call 631-9146.
- Search for:
Related Sites
Kaneb Center – home page Notre Dame Learning
- February 2021
- December 2020
- November 2020
- October 2020
- September 2020
- August 2020
- February 2020
- January 2020
- December 2019
- November 2019
- October 2019
- September 2019
- August 2019
- February 2019
- January 2019
- November 2018
- October 2018
- September 2018
- August 2018
- February 2018
- January 2018
- December 2017
- November 2017
- October 2017
- September 2017
- August 2017
- February 2017
- January 2017
- December 2016
- November 2016
- October 2016
- September 2016
- August 2016
- February 2016
- January 2016
- December 2015
- November 2015
- October 2015
- September 2015
- August 2015
- February 2015
- January 2015
- December 2014
- November 2014
- October 2014
- September 2014
- August 2014
- February 2014
- January 2014
- December 2013
- November 2013
- October 2013
- September 2013
- February 2013
- January 2013
- November 2012
- October 2012
- September 2012
- August 2012
- February 2012
- January 2012
- January 2011
- November 2010
- September 2010
- August 2010
Notes on Teaching and Learning © 2024 All Rights Reserved.
Free WordPress Themes
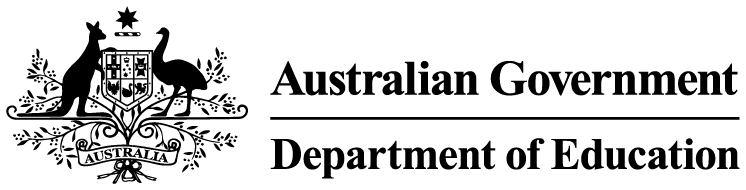
- Good to Great Schools Australia Pilot Program
- Online teaching and learning resources to support mathematics and numeracy
- Support for Arts and Languages
- Support for Humanities and Social Sciences
- Support for Literacy and Numeracy
- National STEM School Education Strategy 2016–2026
- STEM Education Resources Toolkit
- STEM program evaluations in the early years and schooling
- Research into Best Practice Models in mathematics teaching and learning
- Support for Social Cohesion
- Year 1 Phonics Check
Inquiry-based learning
On this page:, what is it.
Inquiry-based learning is an education approach that focuses on investigation and problem-solving. Inquiry-based learning is different from traditional approaches because it reverses the order of learning. Instead of presenting information, or ‘the answer’, up-front, teachers start with a range of scenarios, questions and problems for students to navigate.
Inquiry-based learning prioritises problems that require critical and creative thinking so students can develop their abilities to ask questions, design investigations, interpret evidence, form explanations and arguments, and communicate findings.
How does it help?
Students learn key STEM and life skills through inquiry-based learning. Inquiry-based learning also promotes:
- Social interaction. This helps attention span and develops reasoning skills. Social interaction encourages students to generate their own ideas and critique in group discussions. It develops agency, ownership and engagement with student learning .
- Exploration. This allows students to investigate, design, imagine and explore, therefore developing curiosity, resilience and optimism.
- Argumentation and reasoning. This creates a safe and supportive environment for students to engage in discussion and debate. It promotes engagement in scientific discussion and improves learning of scientific concepts. It encourages students to generate questions , formulate positions and make decisions.
- Positive attitudes to failure. The iterative and evaluative nature of many STEM problems means failure is an important part of the problem-solving process. A healthy attitude to failure encourages reflection, resilience and continual improvement.
How do you do it?
- set a challenge for students
- encourage active student investigations
- make generalisations
- For more information on inquiry-based learning and examples of classroom strategies. Griffith University has prepared a useful resource.
Want to know more?
Research reports.
- STEM Education: A review of the contribution of the disciplines of science, technology, engineering and mathematics - Science Education International Vol. 27, Issue 4, 2016, 530-569
- Opening up pathways : Engagement in STEM across the Primary-Secondary school transition. A review of the literature concerning supports and barriers to Science, Technology, Engineering and Mathematics engagement at Primary- Secondary transition. Commissioned by the Australian Department of Education, Employment and Workplace Relations. June, 2008
- Studies in Science Education - Volume 44, 2008 - Issue 1 - Students' questions: a potential resource for teaching and learning science
- From concept to classroom Translating STEM education research into practice - Australian Council for Educational Research - June 2016
Case study: reSolve: Mathematics by Inquiry
reSolve: Mathematics by Inquiry is a national program to help teachers adopt inquiry-based methods when teaching primary and secondary mathematics. The reSolve approach encourages students to ask questions, test ideas, seek meaning and explain reasons. reSolve provides classroom resources, professional learning modules and a protocol that underpins excellent inquiry-based teaching and learning. It also trains ‘reSolve Champions’: teachers and leaders who take the messages and resources of reSolve into the wider mathematics teaching community. Approximately 300 teachers and leaders have either completed or are undertaking a 12-month professional learning program to become reSolve Champions. The reSolve program is funded by the Australian Government Department of Education Skills and Employment.
- Lesson Sequencing Strategies: A Comprehensive Overview
- Understanding Direct Instruction Methods
- Engaging Cooperative Learning Games
- Physical Science Lesson Plans for 6-8 Learners
- Classroom Management
- Behavior management techniques
- Classroom rules
- Classroom routines
- Classroom organization
- Assessment Strategies
- Summative assessment techniques
- Formative assessment techniques
- Portfolio assessment
- Performance-based assessment
- Teaching Strategies
- Active learning
- Inquiry-based learning
- Differentiated instruction
- Project-based learning
- o2c-library/governance/arc-organisation-reports/final%20report.pdf
- Learning Theories
- Behaviorism
- Social Learning Theory
- Cognitivism
- Constructivism
- Critical Thinking Skills
- Analysis skills
- Creative thinking skills
- Problem-solving skills
- Evaluation skills
- Metacognition
- Metacognitive strategies
- Self-reflection and metacognition
- Goal setting and metacognition
- Teaching Methods and Techniques
- Direct instruction methods
- Indirect instruction methods
- Lesson Planning Strategies
- Lesson sequencing strategies
- Unit planning strategies
- Differentiated Instruction Strategies
- Differentiated instruction for English language learners
- Differentiated instruction for gifted students
- Standards and Benchmarks
- State science standards and benchmarks
- National science standards and benchmarks
- Curriculum Design
- Course design and alignment
- Backward design principles
- Curriculum mapping
- Instructional Materials
- Textbooks and digital resources
- Instructional software and apps
- Engaging Activities and Games
- Hands-on activities and experiments
- Cooperative learning games
- Learning Environment Design
- Classroom technology integration
- Classroom layout and design
- Instructional Strategies
- Collaborative learning strategies
- Problem-based learning strategies
- 9-12 Science Lesson Plans
- Life science lesson plans for 9-12 learners
- Earth science lesson plans for 9-12 learners
- Physical science lesson plans for 9-12 learners
- K-5 Science Lesson Plans
- Earth science lesson plans for K-5 learners
- Life science lesson plans for K-5 learners
- Physical science lesson plans for K-5 learners
- 6-8 Science Lesson Plans
- Earth science lesson plans for 6-8 learners
- Life science lesson plans for 6-8 learners
- Physical science lesson plans for 6-8 learners
- Science Teaching
- Inquiry-Based Learning: An Introduction to Teaching Strategies
Learn about inquiry-based learning, a teaching approach that encourages students to ask questions, find answers, and make meaningful connections. Discover key strategies for implementing inquiry-based learning in your classroom.
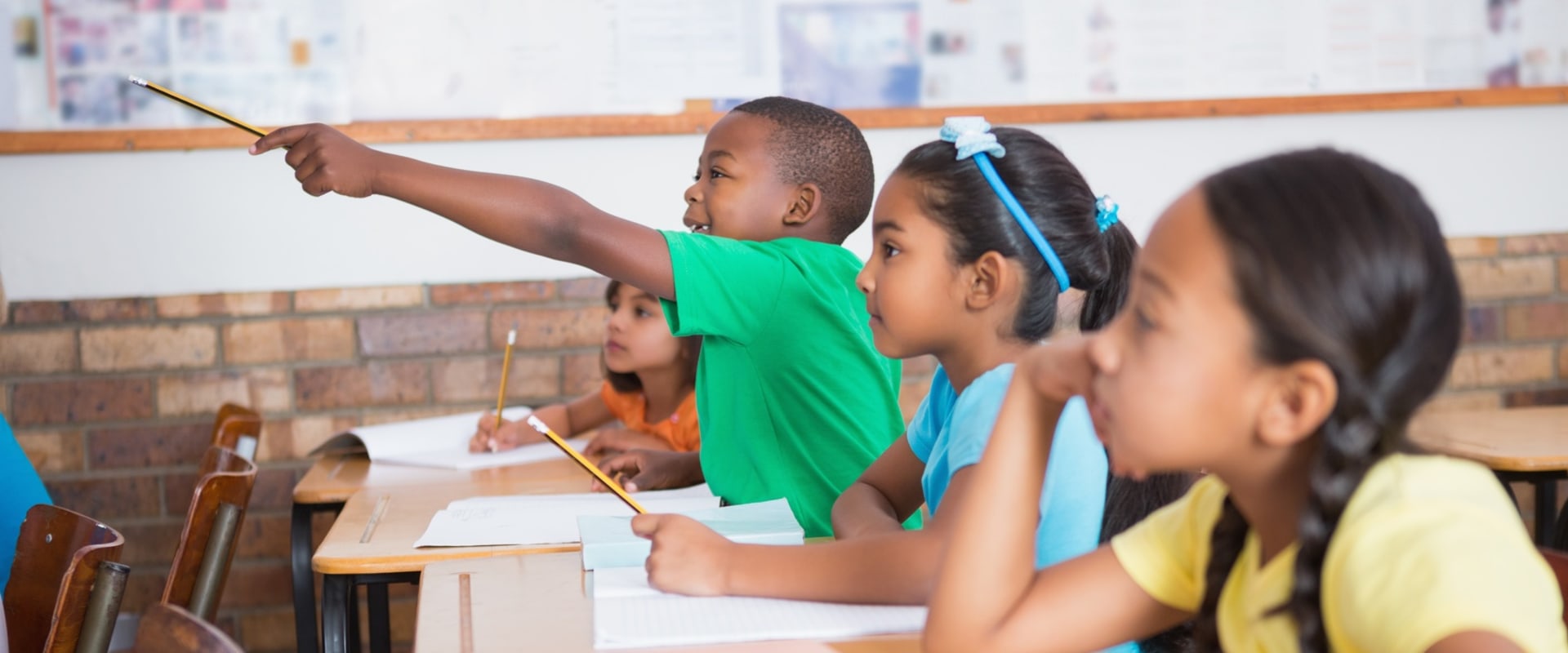
Inquiry-based learning requires teachers to shift the focus away from delivering content and instead emphasize the process of learning. To help students gain a deeper understanding of the world around them, teachers can use Profs online neuroscience tutors to provide guidance and support during the inquiry-based learning process. Teachers should create an environment where students feel comfortable asking questions, exploring ideas, and taking risks through inquiry-based methods. To ensure that students are engaged in their learning, teachers should incorporate opportunities for inquiry-based collaboration and discussion among students.
Moreover, teachers should design meaningful assignments that require students to think critically and make connections between concepts using inquiry-based approaches. To ensure that students are actively involved in their learning, teachers should also provide timely feedback on student progress through inquiry-based methods. This can include providing written or verbal feedback on assignments as well as offering guidance on how to improve work. Teachers can also encourage students to reflect on their own learning process by asking them to think about what worked well and what could be improved upon. Finally, teachers should also consider how they can assess student learning in an inquiry-based classroom. Rather than relying solely on traditional tests or quizzes, teachers should look for ways to assess student understanding through more creative methods such as projects or presentations.
Providing Meaningful Assignments
Additionally, assigning group projects or activities that allow students to collaborate on solving problems is an effective way to further engage students in the inquiry process. In order to create meaningful assignments, teachers should consider providing students with a range of materials to work with. For example, providing students with primary sources such as historical documents or scientific experiments can help them develop a more comprehensive understanding of the material. Additionally, providing students with a range of options for how they can present their research and solutions can help to engage them in the inquiry process.
Offering Timely Feedback
Using technology to provide real-time feedback, giving oral feedback, providing written feedback, using visual aids, creating an inquiry-based learning environment, encourage collaboration:, encourage risk-taking:, provide resources:, be patient:, assessing student learning, observation, performance-based assessments.
This means creating a classroom atmosphere that is conducive to exploration, experimentation, and critical thinking. One way to create an inquiry-based learning environment is to encourage collaboration among students. Group activities and projects can be a great way to foster a collaborative atmosphere in the classroom, as it allows students to work together to find answers to their questions. Additionally, providing students with the freedom to explore and ask questions without fear of being wrong can help create an environment that encourages inquiry.
It is also important for teachers to provide their students with the necessary resources for inquiry-based learning. This includes access to textbooks, reference materials, and other resources such as computers and technology. When students have access to these materials, they are able to research and discover answers on their own. Finally, it is important for teachers to provide guidance and support when necessary.
2.Dialogue:
3.online discussion forums:, 4.peer feedback:, observation:, class discussions:, tests and quizzes:.
By providing meaningful assignments, offering timely feedback, and assessing student understanding through creative methods, teachers can help create an environment where students feel comfortable asking questions and engaging in meaningful discussions. Inquiry-based learning can be used to help students develop critical thinking skills and make meaningful connections that will serve them in their academic and professional lives. Through inquiry-based learning, teachers can help students explore their own curiosities and develop their problem-solving abilities. By encouraging students to ask questions and search for answers, teachers can help create a classroom environment that fosters creativity and collaboration. With the right strategies in place, inquiry-based learning can be a powerful tool for engaging and motivating students.
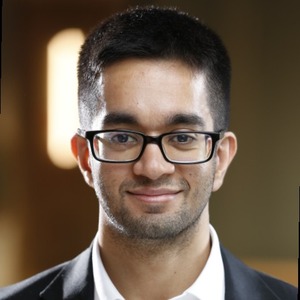
Shahid Lakha
Shahid Lakha is a seasoned educational consultant with a rich history in the independent education sector and EdTech. With a solid background in Physics, Shahid has cultivated a career that spans tutoring, consulting, and entrepreneurship. As an Educational Consultant at Spires Online Tutoring since October 2016, he has been instrumental in fostering educational excellence in the online tutoring space. Shahid is also the founder and director of Specialist Science Tutors, a tutoring agency based in West London, where he has successfully managed various facets of the business, including marketing, web design, and client relationships. His dedication to education is further evidenced by his role as a self-employed tutor, where he has been teaching Maths, Physics, and Engineering to students up to university level since September 2011. Shahid holds a Master of Science in Photon Science from the University of Manchester and a Bachelor of Science in Physics from the University of Bath.
New Articles
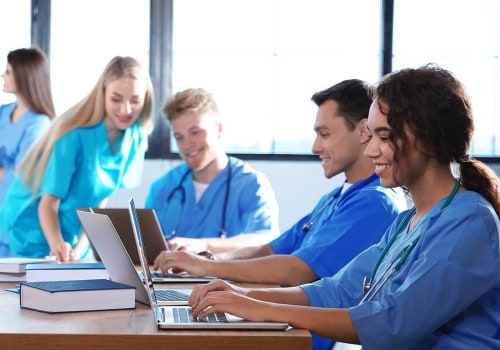
- Active Learning: A Comprehensive Overview
Learn what active learning is and how it can be used as an effective teaching strategy in the classroom.
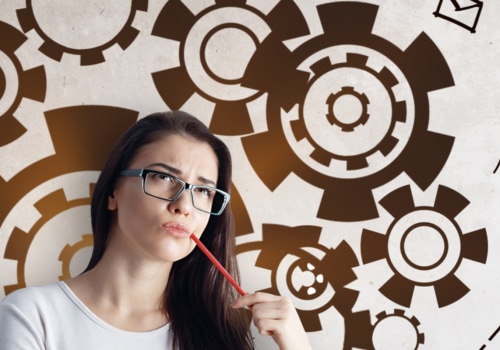
- An Overview of Metacognitive Strategies
Learn about metacognitive strategies for science learning and how to apply them in your studies.
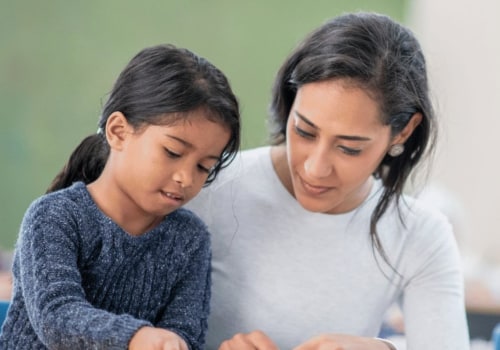
- Summative Assessment Techniques: An Overview
This article provides an overview of summative assessment techniques and how they can be used in the classroom.
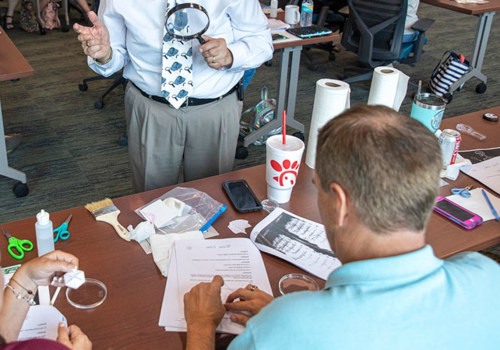
- Life Science Lesson Plans for 9-12 Learners
This article provides an overview of life science lesson plans for 9-12 learners, with clear headings, subheadings, and images to help break up the text.
Leave Reply
Your email address will not be published. Required fields are marked *
I agree that spam comments wont´t be published
- Behavior Management Techniques
- Behaviorism: A Comprehensive Overview
- Social Learning Theory Explained
- Formative Assessment Techniques
- Understanding Cognitivism: A Learning Theory
- Analysis Skills: Understanding Critical Thinking and Science Learning
- Creative Thinking Skills
- Constructivism: Exploring the Theory of Learning
- Problem-solving Skills: A Comprehensive Overview
- Classroom Rules - A Comprehensive Overview
Exploring Portfolio Assessment: An Introduction
- Differentiated Instruction: A Comprehensive Overview
Evaluation Skills: A Comprehensive Overview
- Classroom Routines: A Comprehensive Overview
- Effective Classroom Organization Strategies for Science Teaching
- Project-Based Learning: An In-Depth Look
- Performance-Based Assessment: A Comprehensive Overview
- State Science Standards and Benchmarks
- Course Design and Alignment
- The Advantages of Textbooks and Digital Resources
- Engaging Hands-on Activities and Experiments
- Backward Design Principles: Understanding Curriculum Design
- Integrating Technology into the Classroom
- Understanding Classroom Layout and Design
- Instructional Software and Apps: A Comprehensive Overview
- Understanding Curriculum Mapping
- Collaborative Learning Strategies
- Indirect Instruction Methods: A Comprehensive Overview
- Understanding National Science Standards and Benchmarks
- Exploring Problem-Based Learning Strategies
- Unit Planning Strategies
- Exploring Self-Reflection and Metacognition
- Exploring Goal Setting and Metacognition
- Earth Science Lesson Plans for K-5 Learners
Differentiated Instruction for English Language Learners
- Life Science Lesson Plans for K-5 Learners
- Earth Science Lesson Plans for 6-8 Learners
- Earth Science Lesson Plans for 9-12 Learners
- Life Science Lesson Plans for 6-8 Learners
- Physical Science Lesson Plans for 9-12 Learners
- Physical Science Lesson Plans for K-5 Learners
- Differentiated Instruction Strategies for Gifted Students
Recent Posts
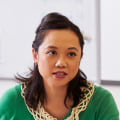
Which cookies do you want to accept?
- Case, scenario, problem, inquiry-based learning
- Teaching guidance
- Teaching practices
- Active learning
Facilitate students to apply disciplinary knowledge, critical thinking and problem-solving skills in safe, real-life contexts.
Case, scenario, problem and inquiry-based learning are active learning strategies suitable for a face-to-face, online or hybrid environment. These approaches require students to apply their disciplinary knowledge, critical thinking and problem-solving skills in a safe, real-world context.
Case-based learning (CBL) presents students with a case or dilemma situated in an authentic context, which they are required to solve. Students are provided with background, situation and supporting data. They can work individually or as a group. The course coordinator takes on a facilitator’s role to guide learning rather than dictate answers.
Scenario-based learning (SBL) uses interactive scenarios based on the principles of situated learning theory (Lave & Wenger, 1991). It works by simulating real-world practice, provide safe opportunities to engage in situations that may be otherwise difficult for students to experience in their studies.
Problem-based learning (PBL) supports learning through an enquiry-guided method for students to solve a real-life problem. Students use ‘triggers’ derived from the problem to define their own learning outcome/objectives. There is a specific, guided methodology for implementing PBL.
Inquiry-based learning (IBL) encourages students to explore material, ask questions, and share ideas in small groups with guided learning. It uses a constructivist approach with the goal for students to make meaning, guided by the Course Coordinators.
Best practice
Technology considerations, case studies, references and further reading, case-based learning (cbl).
A case study is generally based on real situations (names and facts often changed to ensure anonymity). Many case studies include supporting data and documentation and require students to answer an open-ended question or develop a solution(s). The facilitator has an active role in shaping questions that will guide students in their learning.
Most effective cases:
- are developed in line with defined learning objectives
- have an educational purpose
- are authentic and relevant
- draw on common/typical scenarios
- consider dilemmas to promote decision-making
- add supporting data where necessary, and
- have relatable characters, and some include the voice of characters (e.g. patients) to add drama and realism.
In facilitating case-based learning:
- Give students ample time to read and think about the case. You can provide the case before class.
- Introduce the case briefly and provide some guidelines for how to approach it.
- Create groups (ideally 3–6 students) and monitor them to ensure everyone is involved.
- Have groups present their solutions/reasoning.
- Ask questions for clarification and to move discussions to another level.
- Synthesise issues raised. Be sure to bring the various strands of the discussion back together at the end. Ask groups to summarise their findings and compare group responses. Help the whole class interpret and understand the implications of their solutions.
(Adapted from Case Studies , Eberly Center for Teaching Excellence & Educational Innovation, Carnegie Mellon University)
Scenario-based learning (SBL)
Scenarios put students in a simulated context to provide rich learning experiences.
When designing a scenario:
- Identify the learning outcomes . It is important to identify what you want students to achieve on completing the scenario and then work backwards from the learning outcomes to create the situation that will lead to this learning.
- Decide on your format . Is your scenario delivered in face-to-face or online environments? What media (photographs, audio, video) and other resources will you need? If you use an online scenario, will you provide other supporting activities, such as wikis, discussion forums, etc.?
- Choose a topic . Remember that non-routine tasks lend themselves to scenario-based learning. Consider using ‘critical incidents’ and challenging situations that have occurred in your subject area.
- Identify the trigger event or situation . This will be the starting point of your scenario. As you create the scenario, identify decision points and key areas for feedback and student reflection. Creating a storyboard is an effective way to do this.
- Peer review your scenario . Ask colleagues to work through the scenario to ensure that it flows in the way you expect and achieves the outcomes you intended.
Problem-based learning (PBL)
Problem-based learning can be used to engage in active learning that challenges higher-order thinking in collaboration with peers.
There are various ways to plan, design and implement PBL in your classroom. The following resources may suit your context:
- Wood (2007) identified a structure for incorporating PBL into the curriculum and emphasises that PBL will only be successful if the problems developed are of high quality.
- Ganareo and Lyons (2015) outline key steps to design, implement and assess PBL to help develop twenty-first-century skills such as teamwork, digital literacy and problem-solving.
- The ‘Seven Jump’ method (Gijselaers, 1995) used at Maastricht describes the key steps students go through to resolve a problem during PBL tutorial sessions.
Inquiry-based learning (IBL)
Inquiry-based learning (IBL) encourages students to explore a specific topic, ask questions, and share ideas.
Heick identified four phases of Inquiry-Based Learning :
- Interaction : dive into engaging, relevant, and credible media forms to identify a ‘need’ or opportunity for inquiry.
- Clarification : summarising, paraphrasing, and categorising learning with teacher or expert support.
- Questioning : asking questions to drive continued, self-directed inquiry.
- Design : designing an accessible, relevant, and curiosity-driven action or product to culminate and justify inquiry.
When planning case, scenario, problem and inquiry-based learning, you need to consider the context of the learners and select technologies that support the steps you have planned.
- Small group discussion in person or online (e.g. discussion boards , Zoom breakout rooms ).
- Identify relevant questions, (e.g. in person or through PadletUQ ).
- Research (e.g. journal articles, databases, search engines, Library Catalogue)
- Face-to-face or online brainstorming (e.g. discussion boards , PadletUQ, Zoom breakout rooms , or mind map).
- Spreadsheet software (e.g. Microsoft Excel, Google Sheets) for graphing and presenting data.
- Presentation software (e.g. Microsoft PowerPoint, Adobe Express , Prezi) for presenting investigation results.
- Collaborate (e.g. Zoom if presenting online, or Microsoft Teams ).
View centrally-supported active learning tools
View more case studies (UQ Assessment Ideas Factory)
4 Phases of Inquiry-based Learning , Teachthought
Active & Inquiry-based Learning , Victoria University Melbourne Australia
Azer, S. A. (2007). Twelve tips for creating trigger images for problem-based learning cases. Medical Teacher, 29 (2-3), 93-97. doi:10.1080/01421590701291444
Case-based Teaching and Problem-based Learning (University of Michigan, Centre for Research on Learning & Teaching)
Case Studies , Eberly Center for Teaching Excellence & Educational Innovation, Carnegie Mellon University
Clark, R., (2009). Accelerating expertise with scenario-based learning. Learning Blueprint . Merrifield, VA: American Society for Teaching and Development.
Davis, B. (1993). Tools for Teaching . San Francisco: Jossey-Bass.
Davis, C. & Wilcock, E. (2003). Teaching Materials Using Case Studies.
Enquiry-based learning (Griffith University)
Errington, E.P., (2003). Developing scenario-based learning: Practical insights for tertiary educators . Palmerston North, N.Z .: Dunmore Press. 9-20.
Ganareo, V., & Lyons, R. (2015). Problem-Based Learning: Six Steps to Design, Implement, and Assess .
Gijselaers, W. (1995). Perspectives on problem-based learning. In W. Gijselaers, D. Tempelaar, P. Keizer, J. Blommaert, E. Benard, & H. Kasper (Eds.), Educational Innovation in Economics and Business Administration (pp. 39-52). Netherlands: Springer.
Gossman, P., Stewart, T., Jaspers, M., & Chapman, B. (2007). Integrating web-delivered problem-based learning scenarios to the curriculum. Active Learning In Higher Education , 8(2), 139-153.
Journal of University Teaching and Learning Practice, 8 (1), 0-17. Retrieved from http://ro.uow.edu.au/cgi/viewcontent.cgi?article=1149&context=jutlp
Kindley, R. W. (2002). Scenario-based e-learning: a step beyond traditional e-learning. ASTD Magazine . Retrieved from http://www.astd.org/
Problem-Based Learning at Maastricht University
Retrieved from https://www.facultyfocus.com/articles/course-design-ideas/problem-based-learning-six-steps-to-design-implement-and-assess/
Ribeiro, L. R. C. (2011). The Pros and Cons of Problem-Based Learning from the Teacher's Standpoint.
Savery, John R. (2006) Overview of Problem-based Learning: Definitions and Distinctions, Interdisciplinary Journal of Problem-based Learning 1 (1)
Schwartz, P., Mennin, S., & Webb, G. (2001). Problem-Based Learning: Case Studies, Experience and Practice (Eds.). London, UK: Kogan Page Limited.
Using Case Studies to Teach , Centre for Excellence and Innovation in Teaching, Boston University
Weimer, M. (2009). Problem-Based Learning: Benefits and Risks . Retrieved from http://www.facultyfocus.com/articles/effective-teaching-strategies/problem-based-learning-benefits-and-risks/
Wood, D. F. (2003). Problem-based learning. BMJ, 326, 328-330. doi: 10.1136/bmj.326.7384.328
- Project-based learning
- Reflective learning
- Collaborative learning
- Experiential learning
- In-class active learning activities
Community of Practice
If you want to know more about how you could implement active learning in your courses, consider joining the Blended and Active Learning Innovation Community of Practice (BALI CoP) .
Resources
- Active learning tools
- UQ Assessment Ideas Factory
ITaLI offers personalised support services across various areas including case, scenario, problem, inquiry-based learning.
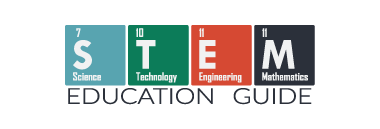
Transform Your Classroom with Inquiry-Based Strategies
Krystal DeVille
August 17, 2023
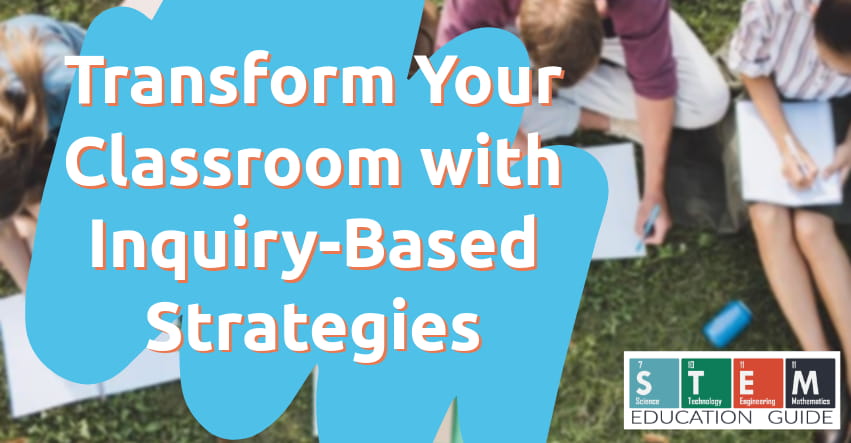
Inquiry-based learning builds on this fundamental drive to discover, improve, understand, and create a better world in the face of uncertainty.
Inquiry-based strategies provide teachers with powerful tools to facilitate interactive learning and collaborative problem-solving. Teachers can use inquiry-based instruction to guide students through a deeper understanding of core concepts and challenge them to think critically about their work.
This article will explore how inquiry-based strategies can transform classrooms into creative learning environments that support student growth.
Table of Contents
What is Inquiry-Based Learning
Humans have been practicing various forms of inquiry-based learning for centuries, including great thinkers like Einstein and Socrates. It happens when learners are curious and engaged in solving an authentic, meaningful problem. That’s the basic gist of it: asking intriguing questions and searching for useful answers.
Depending on the practitioner and context, inquiry-based learning can be more student-centered or teacher-led. This video from John Spencer suggests four levels of inquiry, which depend on the adult or teacher’s relative involvement, compared to the youth or students’ freedom.
We will delve more deeply into the process of inquiry-based learning and planning — including some of the specific steps that benefit from more or less teacher involvement — but in general, it’s helpful to remember that more student action equals more student engagement.
Sure, listening is a form of engagement, too, but are they really listening?
At its best, inquiry-based learning is an experiential practice that allows children to wrestle with real, relevant problems and to propose and enact their own creative solutions.
Benefits of Inquiry-Based Learning
The benefits of inquiry-based learning depend a bit on how it’s practiced. One of the clearest benefits is the built-in contextualization.
One year, I worked in a school with a shared annual goal for all teachers to practice purposing each lesson. This meant letting students know why a given lesson or topic was useful or relevant to their lives— why should they even learn this?
Sounds pretty straightforward, right?
Providing a solid reason or motivation to learn helps boost engagement and earn more student buy-in. Plus, it helps you (the teacher) frame the work, too.
It might guide your choice of applied learning tasks, inspire you to bring in a particular object to show, remind you of a story to tell, or it might even change the way you explain something.
Teachers can increase engagement even more by letting the students determine the purpose of a lesson (with varying levels of guidance as appropriate). In inquiry-based learning, this means allowing learners to ask their own questions and develop their own research focus.
Let’s look at how this works.
Examples of Inquiry-Based Learning
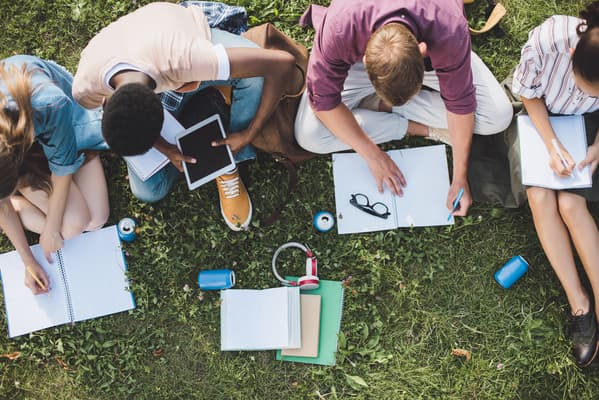
Words like inquiry, problem-solving, and research often remind us of STEM fields, which seem to be naturally inquiry-based. But just carrying out experiments or solving math problems is not necessarily inquiry-based on its own — it’s all about that context.
Inquiry-based learning is usually experiential (i.e. hands-on) and project-based. As a STEM educator, you might walk students through a robot-building kit, sharing the ins and outs of circuitry, measurement, and building. At the end of the day, they’ll be able to build a robot as well as anybody else with all the parts laid out.
But what if they don’t care about robots? What did they learn? How much will they remember?
In inquiry-based learning, there is more room for creativity, invention, and deeper understanding. What good is robotics? Why was this field developed? What tasks would be easier completed with robotic support? Where have you seen robotics used in the world? What materials are involved? Are they the best materials? Why or why not.
The questions could be infinite!
Make sure you don’t ask all of them; just ask a few and let the students ask even more. This is one of those times where it’s best if you don’t have all of the answers, so give yourself a break. You’ll want to track them down together, in a voyage of pure discovery.
The science fair is a classic example of inquiry-based learning, where students come up with their own projects, their own process, and their own rationale.
Last year, high school student Dasia Taylor was inspired to enter her state-wide science fair with an amazing idea. She had learned about color-changing sutures that could detect infection and wound-healing status, but they were accessible only to the wealthy.
Driven by a well-researched need for better access and equity, particularly among low-income communities and developing countries, Dasia invented a version of the color-changing sutures that would be more affordable and could be more widely produced. Read about her focus on racial equity and her personal drive to solve this big problem.
Dasia and the science fair provide an awesome example of inquiry-based learning addressing a real-world problem, and genuine curiosity and practice paving the way to success. If you can find ways to harness that science fair mentality more often, including for other topics, your learners might surprise you with their ingenuity.
This example from Youthlearn.org walks you through the process of an inquiry-based lesson on a social studies topic. It allows students to practice using valuable resources, like nonfiction texts and computers, when a purely hands-on approach may not always be available or appropriate.
Gifted students take different approaches and require new methods to help them learn best. Join us in our article, Teaching Strategies & Tips for Gifted Students .
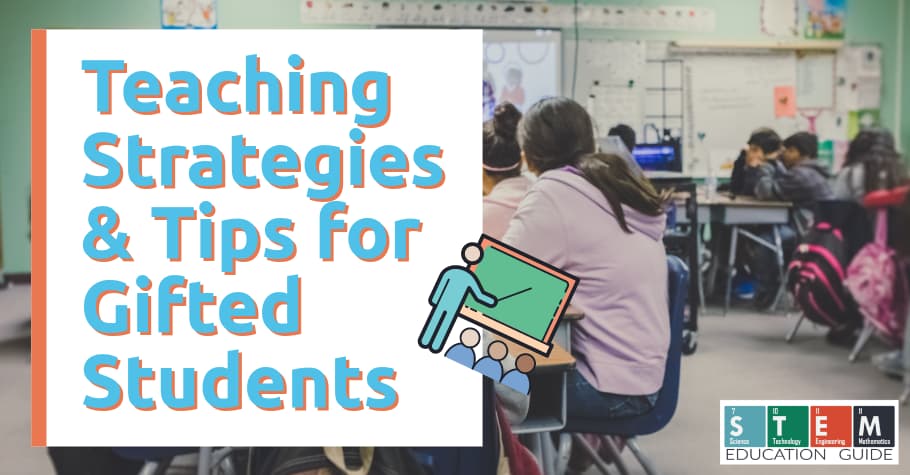
Challenges of Inquiry-Based Learning
A more student-centered, open-ended inquiry process can be hard for many educators to embrace.
As the responsible adult in the room, it can be scary to let go of the reins. We also know that certain learners need more targeted guidance and support, and finding the right mix of teacher direction and student ownership can be tricky.
Inquiry-based learning also requires a hefty number of supplies. Whether books, tech resources, science equipment, building supplies, or even study participants, students are going to need a lot of stuff. Planning and preparing is essential. And getting a big teacher bag.
Additionally, inquiry-based lessons can become surprising, frustrating, or unpredictable with diverse groups of students who all have such different backgrounds and knowledge, you can’t always guess what direction the learning will take. This makes it tough to plan.
That brings us to my favorite strategy for implementing this style of learning with your kids.
Pedagogy encompasses both the what and the why: what teaching strategies are you employing throughout a lesson, and why did you choose those? Check out our article all about Pedagogy Principles to Use in Your Classroom .
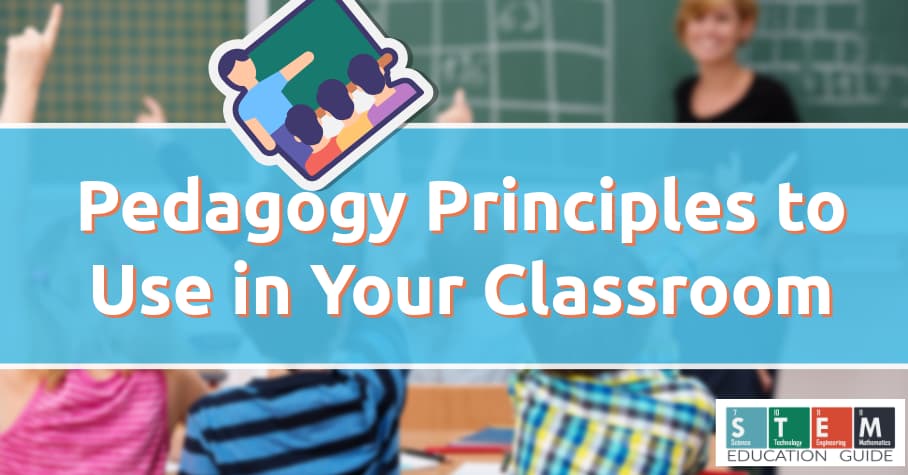
Strategies for Implementing Inquiry-Based Learning
You can find an array of processes and formulas for inquiry-based learning from various authors, teachers, and theorists. I like the 5 E’s laid out by Dr. Sam Northern in the American Association of School Librarians (AASL) journal publication Knowledge Quest . The 5 E’s are:
In this model, instruction begins with the students (not the teacher). Learners are immediately engaged with the material, either something hands-on that they can interact with or even a compelling video or text.
During the engagement phase, the teacher might offer a few prompts but is ultimately an observer, assessing students’ existing knowledge and understanding.
Learners continue to explore the subject at hand, asking their own questions, forming hypotheses, talking to each other, and trying things out. They are inspired by peers and by the task or objects in front of them.
As learners eventually begin to explain and elaborate together, the teacher may take a more active role in guiding the conversation, providing additional information, highlighting key points, and making important whiteboard notes.
This model was designed for STEM learning, but it can be applied across fields. As with project-based learning, inquiry-based strategies provide a great jumping-off point for cross-curricular thematic units .
Wrapping Up Inquiry-Based Strategies
Inquiry-based learning creates an environment in which students’ limits are not defined by the lesson, but by the depths of their own curiosity and enterprise. In this way, it becomes possible to reach beyond the curriculum, using existing material as a jumping-off point toward more.
Teachers serve as observers, supporters, and guides, but the learners lead. Bring your own set of questions as an emergency tool and a backup, but go into your inquiry-based lesson without too many specific expectations. Who knows what you and your learners may discover!
Are you looking for a pick-me-up? Please take a look at our article all about Inspirational Quotes for Teachers .
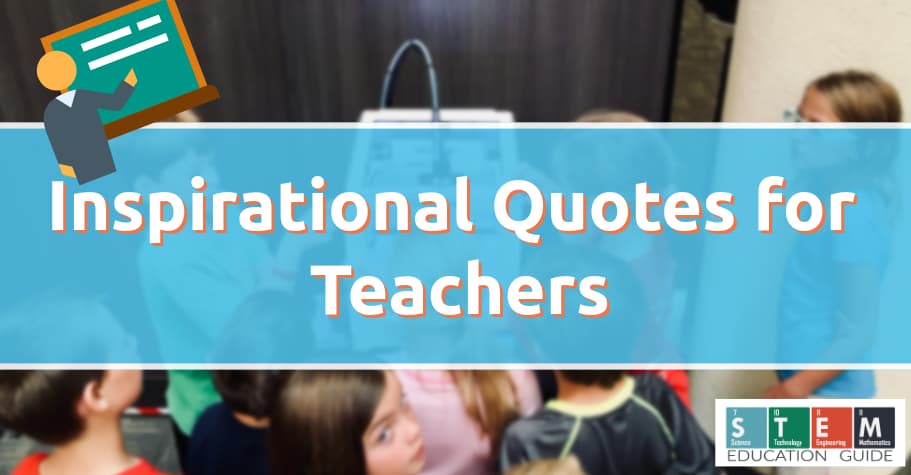
Leave a Comment Cancel reply
Save my name, email, and website in this browser for the next time I comment.
most recent
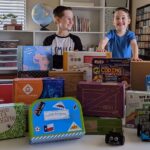
Activities and Games , Toy Gift Guides
Best stem subscription boxes for kids: hands-on reviews.

10 Honest Insights: How Do You Make Your Kids Eat Vegetables They Don’t Like?

10 Absolute Worst Parts About Having a Child

Essential Tips for Building Your Child’s Emotional Intelligence
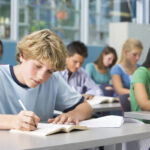
What is STEM? What You Need to Know
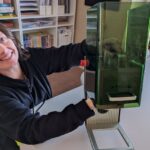
Laser Engraving , Activities and Games , Art Projects , Technology
Laser engraving: steam education and creative expression.

xTool F1 Laser Engraver Review: Performance and Precision
STEM Education Guide
[email protected] STEM Education Guide 9125 SVL BOX Victorville, CA 92395
Your Compass for STEM Discovery
© 2024 STEM Education Guide
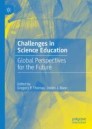
Challenges in Science Education pp 39–58 Cite as
Teaching Science That Is Inquiry-Based: Practices and Principles
- Robyn M. Gillies 3
- First Online: 09 February 2023
440 Accesses
Evidence has emerged in recent years regarding the importance of teaching science through an inquiry-based approach where students are encouraged to be actively involved in investigations that challenge their curiosity, encourage them to ask questions, explore potential solutions, use evidence to help explain different phenomena, and predict outcomes under different conditions. The inquiry process is complex and multifaceted as it involves students reconciling their current understandings of a problem with both the evidence obtained from an inquiry while also being able to demonstrate their understandings in ways that are logical, well-reasoned, and viewed as justifiable. Drawing on current research, this chapter proposes three curriculum-based interventions in cooperative learning, scientific literacy, and scientific discourses that have the potential to promote student understanding during inquiry learning.
- Inquiry-based science
- Scientific literacy
- Cooperating to learn
- Scientific discourse
This is a preview of subscription content, log in via an institution .
Buying options
- Available as PDF
- Read on any device
- Instant download
- Own it forever
- Available as EPUB and PDF
- Compact, lightweight edition
- Dispatched in 3 to 5 business days
- Free shipping worldwide - see info
- Durable hardcover edition
Tax calculation will be finalised at checkout
Purchases are for personal use only
Alexander, R. (2008). Culture, dialogue and learning: Notes on an emerging pedagogy. In N. Mercer & S. Hodgkinson (Eds.), Exploring talk in school (pp. 91–114). Sage.
Google Scholar
Areepattamannil, S., Cairns, D., & Dickson, M. (2020). Teacher-directed versus inquiry-based science instruction: Investigating links to adolescent students’ science dispositions across 66 countries. Journal of Science Teacher Education, 31 , 675–704. https://doi.org/10.1080/1046560X.2020.1753309
Article Google Scholar
Bathgate, M., Crowell, A., Schunn, C., Cannady, M., & Dorph, R. (2015). The learning benefits of being willing and able to engage in scientific argumentation. International Journal of Science Education, 37 , 1590–1612. https://psycnet.apa.org/doi/10.1080/09500693.2015.1045958
Bybee, R. (2015). The BSCS 5 E Instructional Model: Creating teachable moments (p. 126). National Science Teachers’ Association Press.
Bybee, R. (2019). Using the BSCS 5E Instructional Model to introduce STEM Disciplines. Science and Children, 56 (6), 8–12.
Bybee, R., & Van Scotter, P. (2007). Reinventing the science curriculum. Educational Leadership, 64 (4), 43–47.
Chinn, C., Duncan, R., Dianovsky, M., & Rhinehart, R. (2013). Promoting conceptual change through inquiry. In S. Vosniadou (Ed.), International handbook of research in conceptual change (2nd ed., pp. 339–559). Routledge.
Duschl, R., & Grandy, R. (2008). Reconsidering the character and role of inquiry in school science: Framing the debates. In R. Duschl & R. Grandy (Eds.), Teaching scientific inquiry: Recommendations for research and implementation (pp. 1–37). Sense.
Chapter Google Scholar
Felton, M., Garcia-Mila, M., & Gilabert, S. (2009). Deliberation versus dispute: The impact of argumentative discourse on learning and reasoning in the science classroom. Informal Logic, 29 (417), 446. https://doi.org/10.22329/il.v29i4.2907
Felton, M., Garcia-Mila, M., Villarroel, C., & Gilabert, S. (2015). Arguing collaboratively: Argumentative discourse types and their potential for knowledge building. British Journal of Educational Psychology, 85 , 372–386. https://psycnet.apa.org/doi/10.1111/bjep.12078
Firman, M., Ertikanto, C., & Abdurrahman, A. (2019). Description of meta-analysis of inquiry-based learning of science in improving students’ inquiry skills. International Conference on Mathematics and Science Education , 1–6. Journal of Physics Conference Series. 1157 022018.
Ford, M. J., & Forman, E. A. (2015). Uncertainty and scientific progress in classroom dialogue. In L. B. Resnick, C. S. C. Asterhan, & S. N. Clarke (Eds.), Socializing intelligence through academic talk and dialogue (pp. 143–156). AERA.
Giamellaro, M. (2014). Primary contextualization of science through immersion in content-rich settings. International Journal of Science Education, 36 , 2848–2871. https://doi.org/10.1080/09500693.2014.937787
Gillies, R. M. (2007). Cooperative learning: Integrating theory and practice . Sage. https://doi.org/10.4135/9781483329598
Book Google Scholar
Gillies, R. M. (2016). Enhancing classroom-based talk: Blending practice, research and theory . Routledge.
Gillies, R. M. (2020). Inquiry-based science education . CRC Press. https://doi.org/10.1201/9780429299179
Gillies, R. M., & Nichols, K. (2015). How to support primary teachers’ implementation of inquiry: Teachers’ reflections on teaching cooperative inquiry-based science. Research in Science Education, 45 , 171–191. https://doi.org/10.1007/s11165-014-9418-x
Gillies, R. M., Nichols, K., Burgh, G., & Haynes, M. (2012). The effects of two strategic and meta-cognitive questioning approaches on children’s explanatory behaviour, problem-solving, and learning during cooperative, inquiry-based science. International Journal of Educational Research, 53 , 93–106. https://doi.org/10.1016/j.ijer.2012.02.003
Gillies, R. M., Nichols, K., Burgh, G., & Haynes, M. (2014). Primary students scientific reasoning and discourse during cooperative inquiry-based science activities. International Journal of Educational Research, 63 , 127–140. https://doi.org/10.1016/j.ijer.2013.01.001
Howe, C., & Abedin, M. (2013). Classroom dialogue: A systematic review across four decades of research. Cambridge Journal of Education, 43 , 325–356. https://doi.org/10.1080/0305764X.2013.786024
Huff, K., & Bybee, R. (2013). The practice of critical discourse in science classrooms. Science Scope, 36 (9), 30–34.
Johnson, D., & Johnson, R. (2002). Learning together and alone: Overview and meta-analysis. Asia Pacific Journal of Education, 22 , 95–105. https://doi.org/10.1080/0218879020220110
Johnson, D., & Johnson, F. (2009). Joining together: Group theory and group skills (10th ed.). Allyn and Bacon.
Johnson, D., Johnson, R., Roseth, C., & Shin, T. (2014). The relationship between motivation and achievement in interdependent situations. Journal of Applied Social Psychology, 44 , 622–633. https://doi.org/10.1111/jasp.12280
Kang, J., & Keinonen, T. (2018). The effect of student-centered approaches on students’ interest and achievement in science: Relevant topic-based, open and guided inquiry-based, and discussion-based approaches. Research in Science Education, 48 , 865–885. https://doi.org/10.1007/s11165-016-9590-2
King, A. (1997). Ask to think-tel why: A model of transactive peer tutoring for scaffolding higher level complex learning. Educational Psychologist, 32 , 221–235. https://doi.org/10.1207/s15326985ep3204_3
Krajcik, J., & Sutherland, L. (2010). Supporting students in developing literacy in science. Science, 328 , 456–459. https://www.science.org/doi/10.1126/science.1182593
Lazonder, A., & Harmsen, R. (2016). Meta-analysis of inquiry-based learning: Effects of guidance. Review of Educational Research, 86 , 681–718. https://doi.org/10.3102/0034654315627366
Liou, P. (2020). Students’ attitudes towards science and science achievement: An analysis of the differential effects of science instructional practices. Journal of Research in Science Teaching, 58 , 310–331. https://doi.org/10.1002/tea.21643
Liu, O., Lee, H., & Linn, M. (2010). An investigation of teacher impact on student inquiry science performance using a hierarchical linear model. Journal of Research in Science Teaching, 47 , 807–819. https://doi.org/10.1002/tea.20372
Murphy, C., Smith, G., & Broderick, N. (2019). A starting point: Provide children opportunities to engage with scientific inquiry and nature of science. Research in Science Education, 51 , 1759–1793. https://doi.org/10.1007/s11165-019-9825-0
National Research Council. (1996). National Science Education Standards . National Academy Press.
National Science Teachers Association. (2004). NSTA position statement: Scientific Inquiry . Retrieved from http://www.nsta.org/about/positions/inquiry.aspx/
Rennie, L. (2005). Science awareness and scientific literacy. Teaching Science, 51 (1), 10–14. http://hdl.handle.net/20.500.11937/31481
Resnick, L., Michaels, S., & O’Connor, C. (2010). How (well structured) talk builds the mind. In D. Pressis & R. Sternberg (Eds.), Innovations in educational psychology: Perspectives on learning, teaching and human development (pp. 163–194). Springer.
Salchegger, S., Wallner-Paschon, C., & Bertsch, C. (2021). Explaining Waldorf Students’ high motivation but moderate achievement in science: Is inquiry-based science education the key? Large Scale Assessments in Education, 9 , 14. https://doi.org/10.1186/s40536-021-00107-3
Soysal, Y. (2021). Argument-based inquiry, teachers’ talk moves, and students’ critical thinking in the classroom. Science & Education, 30 , 33–65. https://doi.org/10.1007/s11191-020-00163-1
Tang, K., & Moje, E. (2010). Relating multimodal representations to the literacies of science. Research in Science Education, 40 , 81–85. http://hdl.handle.net/20.500.11937/27263
Treagust, D., Qureshi, S., Vishnumolakala, V., Ojeil, J., Mocerino, M., & Southam, D. (2020). Process-orientated guided learning inquiry (POGIL) as a culturally relevant pedagogy (CRP) in Qatar: A perspective from Grade 10 chemistry classes. Research in Science Education, 50 , 813–831. https://doi.org/10.1007/s11165-018-9712-0
Tseng, C., Tuan, H., & Chin, C. (2013). How to help teachers develop inquiry teaching: Perspectives from experienced science teachers. Research in Science Education, 43 , 809–825. https://doi.org/10.1007/s11165-012-9292-3
Download references
Author information
Authors and affiliations.
The University of Queensland, St Lucia, QLD, Australia
Robyn M. Gillies
You can also search for this author in PubMed Google Scholar
Corresponding author
Correspondence to Robyn M. Gillies .
Editor information
Editors and affiliations.
The University of Alberta, Edmonton, AB, Canada
Gregory P. Thomas
James Cook University, Townsville, QLD, Australia
Helen J. Boon
Rights and permissions
Reprints and permissions
Copyright information
© 2023 The Author(s), under exclusive license to Springer Nature Switzerland AG
About this chapter
Cite this chapter.
Gillies, R.M. (2023). Teaching Science That Is Inquiry-Based: Practices and Principles. In: Thomas, G.P., Boon, H.J. (eds) Challenges in Science Education. Palgrave Macmillan, Cham. https://doi.org/10.1007/978-3-031-18092-7_3
Download citation
DOI : https://doi.org/10.1007/978-3-031-18092-7_3
Published : 09 February 2023
Publisher Name : Palgrave Macmillan, Cham
Print ISBN : 978-3-031-18091-0
Online ISBN : 978-3-031-18092-7
eBook Packages : Education Education (R0)
Share this chapter
Anyone you share the following link with will be able to read this content:
Sorry, a shareable link is not currently available for this article.
Provided by the Springer Nature SharedIt content-sharing initiative
- Publish with us
Policies and ethics
- Find a journal
- Track your research
- e-Learning Glossary
What is Inquiry-based Learning: Benefits, Key Elements and Challenges
Discover the transformative power of Inquiry-Based Learning (IBL) in our comprehensive guide. Learn how IBL shifts the focus to student curiosity, fostering engagement, critical thinking, and real-world problem-solving skills for a dynamic classroom experience.
Inquiry-Based Learning (IBL) is a pedagogical approach that shifts from traditional rote memorization to a student-centered learning process.
Central to IBL is the idea that learning is driven by student inquiries, problems, or scenarios, rather than by direct instruction. In this model, learners actively participate in their education, engaging in exploration, questioning, and discovery. IBL emphasizes critical thinking, problem-solving, and the active application of knowledge in real-world contexts.
It fosters a dynamic classroom environment where students take ownership of their learning, collaborating with peers and engaging in hands-on activities. This approach not only enhances students' cognitive skills but also prepares them for real-life challenges by promoting adaptability, creativity, and lifelong learning.
Key Characteristics of Inquiry-based Learning
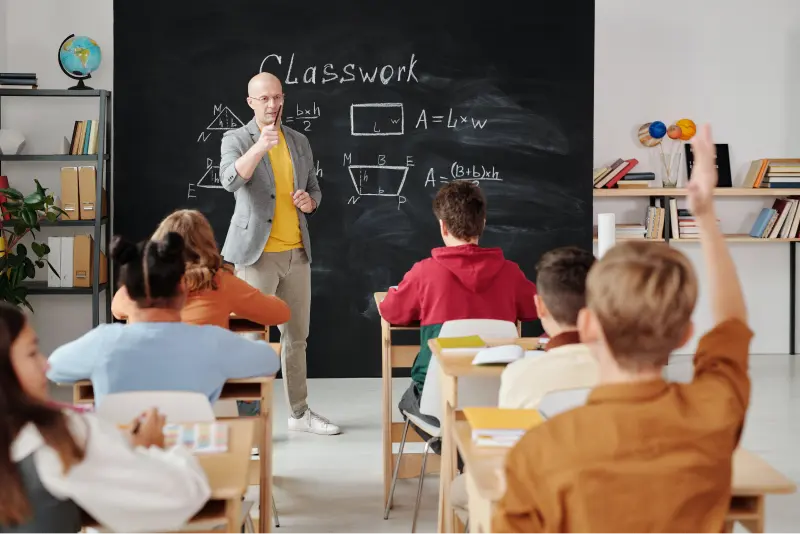
Inquiry-Based Learning is defined by several core features that facilitate a dynamic and interactive learning environment.
Understanding these characteristics is essential for educators aiming to implement IBL in their classrooms effectively.
Student-Centered Approach
At the heart of IBL is the shift from teacher-centered instruction to student-centered learning. This paradigm shift places students at the forefront of their learning journey, empowering them to take ownership of their educational experiences. Teachers serve as guides or facilitators, providing support and resources rather than direct instruction. This approach encourages students to explore their interests and questions, fostering a deeper connection to the material.
Active Learning
IBL promotes active engagement with the subject matter . Students are involved in hands-on activities, experiments, and discussions that require critical thinking and problem-solving skills. This engagement goes beyond passive listening, encouraging learners to apply concepts in real-world contexts, which enhances retention and understanding.
Collaborative Environment
Collaboration is a key element of IBL, with students often working in groups to explore questions or solve problems. This collaborative learning environment fosters communication, teamwork, and the exchange of diverse perspectives, aligning with Vygotsky's emphasis on social interaction in cognitive development.
Focus on the Process
Unlike traditional learning, which often emphasizes the final product or the correct answer, IBL focuses on the learning process itself. Students are encouraged to explore various pathways, reflect on their thinking, and understand the rationale behind their conclusions. This focus on process over product cultivates a growth mindset and resilience, as students learn to see challenges and mistakes as opportunities for learning.
Development of Critical Thinking and Inquiry Skills
IBL explicitly aims to develop skills such as questioning, investigating, and synthesizing information. Students learn to ask meaningful questions, seek out resources, and critically evaluate information. These inquiry skills are invaluable in an information-rich age, preparing students to navigate complex and often ambiguous real-world problems.
Integration of Interdisciplinary Knowledge
IBL often involves integrating knowledge and skills from various disciplines, reflecting the interconnected nature of real-world issues. This interdisciplinary approach helps students see the relevance of what they are learning and how it applies beyond the classroom walls.
The Four Phases of Inquiry-Based Learning
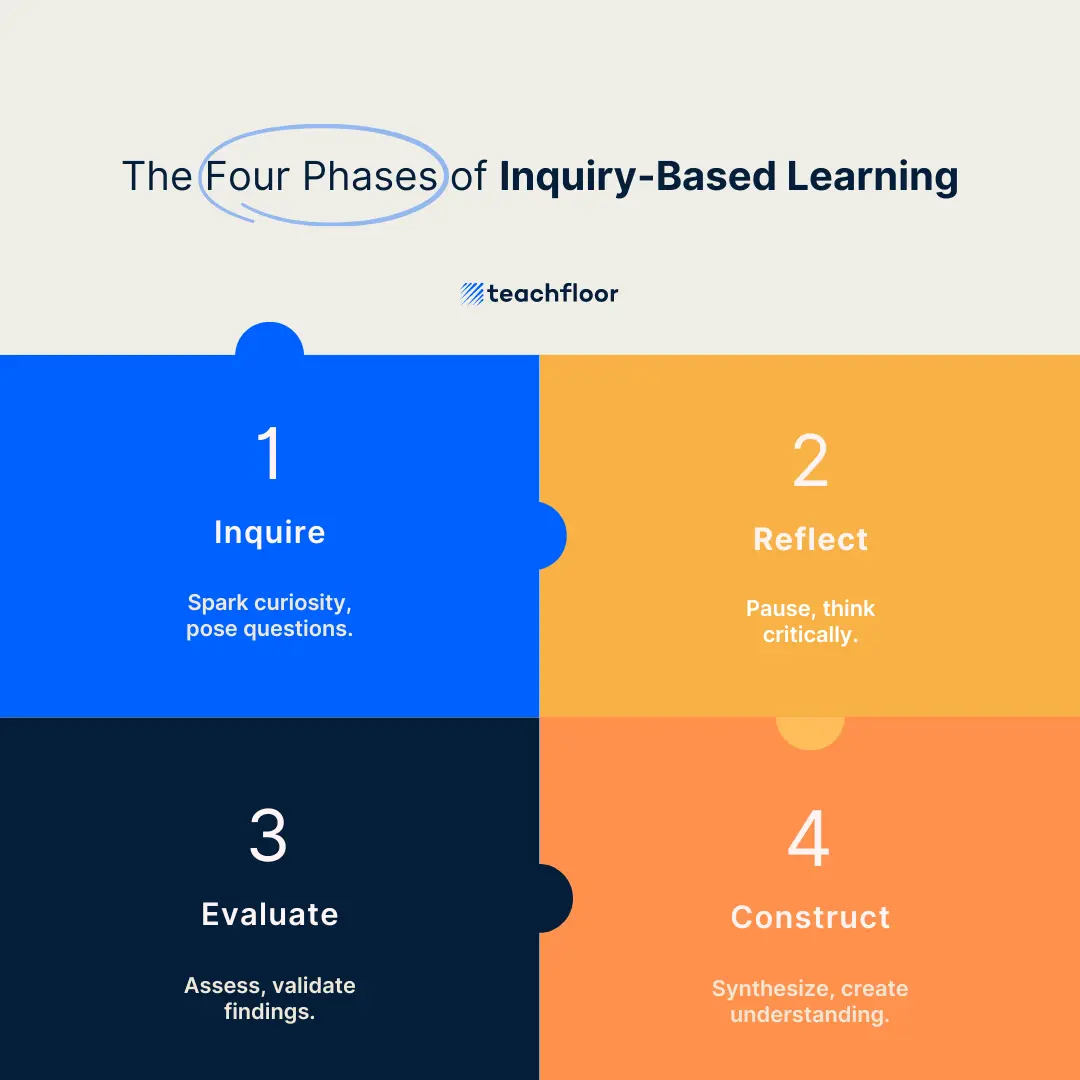
To deepen our understanding of Inquiry-Based Learning (IBL) and its practical application, it's essential to recognize the structured approach that underpins this educational strategy.
Central to the effectiveness of IBL is its division into distinct phases, each serving a specific purpose in guiding learners through the complex process of inquiry.
These phases create a scaffolded framework that supports students in navigating their learning journey, from initial curiosity to deep exploration and reflection
This initial phase is where curiosity is sparked and the direction of learning is set. It involves the identification of a question or problem that is both relevant to the curriculum and engaging to the students.
This stage is characterized by open-ended questions that prompt students to investigate deeply, leveraging prior knowledge and identifying areas where further information is needed.
Techniques such as brainstorming, preliminary research, and hypothesis formulation are key in this phase, setting the stage for a guided yet exploratory learning journey.
Reflection is integral to the learning process, providing students with the opportunity to pause and consider what they have learned, how they have learned it, and what it means in the broader context of their studies and personal experiences.
This phase encourages metacognitive skills, asking students to think about their own thinking and learning processes. Reflection can be facilitated through various methods, including journaling, discussion forums, or peer feedback sessions, allowing students to consolidate their understanding and prepare for deeper evaluation.
Evaluation involves a critical assessment of both the learning process and the outcomes achieved. In this phase, students are engaged in analyzing the information gathered, assessing the validity and reliability of their sources, and determining the relevance of their findings to the original question or problem.
This critical appraisal extends to self-evaluation, where students assess their research strategies, collaboration effectiveness, and problem-solving approaches. The evaluation phase is crucial for developing critical thinking and analytical skills, as it requires students to apply criteria-based judgment to their work and the work of their peers.
The final phase of the IBL process is where students synthesize their findings and insights to construct new knowledge, solutions, or understandings. This construction can take many forms, from written reports and presentations to creative projects or practical solutions.
The key is that the output demonstrates a deep understanding of the subject matter , informed by the inquiry, reflection, and evaluation phases. This phase not only culminates the learning process but also demonstrates the application of knowledge in a tangible or conceptual form, showcasing students' ability to think critically and creatively.
Benefits of Inquiry-Based Learning
Enhanced critical thinking and problem-solving skills.
One of the most significant benefits of IBL is its capacity to enhance critical thinking and problem-solving skills. By engaging students in the process of questioning, exploring, and synthesizing information, IBL encourages a deeper level of cognitive processing. Students learn to approach problems from multiple angles, evaluate evidence, and develop reasoned arguments, equipping them with the ability to tackle complex and unfamiliar situations effectively.
Increased Engagement and Motivation
IBL's student-centered approach fosters a learning environment where students feel more connected and engaged with the material. By allowing learners to follow their interests and questions, IBL taps into intrinsic motivation, making learning more meaningful and enjoyable. This engagement is further amplified through hands-on activities and collaborative projects, which add relevance and context to academic concepts.
Improved Communication and Collaboration Skills
The collaborative nature of IBL activities helps students develop essential communication and teamwork skills. As students work together to explore questions and solve problems, they learn to articulate their ideas, listen to others, and integrate diverse perspectives. These social skills are crucial for success in both academic and professional settings, promoting a culture of respect and cooperation.
Fosters Independence and Self-Directed Learning
IBL encourages students to take ownership of their learning process, fostering independence and self-directed learning skills. By navigating their inquiries and managing their projects, students build confidence in their ability to learn and find information. This autonomy prepares them for lifelong learning, enabling them to adapt to new challenges and continuously acquire new knowledge.
Promotes Adaptability and Flexibility
The open-ended nature of IBL challenges students to adapt to changing conditions and embrace ambiguity. As students navigate the inquiry process, they learn to be flexible in their thinking and approach, skills that are increasingly valuable in a rapidly changing world. This adaptability is crucial for innovation and creativity, as it encourages learners to see challenges as opportunities for growth.
Supports Differentiated Learning
IBL naturally accommodates diverse learning styles and paces, offering differentiated pathways for students to engage with content. Teachers can tailor activities to meet individual needs, providing support where necessary while challenging students to stretch their abilities. This personalized approach helps ensure that all students can find success and feel valued within the learning community.
Challenges and Criticisms of Inquiry-based Learning
Resource and time intensive.
One of the primary challenges of implementing IBL is that it can be significantly more resource and time-intensive than traditional teaching methods. Planning and facilitating inquiry-based activities require a considerable amount of preparation, including the development of suitable projects, the sourcing of materials, and the creation of assessment criteria that accurately measure learning outcomes. Additionally, the nature of IBL means that class periods may not follow a predictable schedule, necessitating a flexible approach to curriculum planning and time management.
Requires High Levels of Teacher Expertise
Effective IBL facilitation demands a high level of pedagogical expertise from educators. Teachers must be skilled in guiding students through the inquiry process, providing just enough support to keep them engaged without taking over the process. This balance can be challenging to achieve, especially for educators accustomed to more traditional, direct instructional roles. Moreover, teachers need to be proficient in a range of assessment strategies to evaluate student learning in IBL settings accurately.
Potential for Reduced Coverage of Curriculum
Critics of IBL often express concern that the approach may lead to reduced coverage of the curriculum. Because IBL activities can take more time than traditional lessons, there's a risk that students may not be exposed to the full breadth of content required by standardized curricula. This concern is particularly relevant in subjects where a vast amount of knowledge needs to be covered within a limited time frame.
Assessment Challenges
Assessing student learning in IBL environments poses unique challenges. Traditional tests and quizzes may not effectively capture the depth of understanding or the range of skills developed through inquiry-based activities. Educators must therefore employ a variety of assessment methods, such as portfolios, presentations, and self-assessments , to get a comprehensive view of student achievement. Developing and grading these alternative assessments can be time-consuming and subjective.
Equity Concerns
Finally, there are equity concerns associated with IBL. Students from diverse backgrounds may have different levels of access to resources, support, and prior knowledge, potentially affecting their ability to engage fully with IBL activities. Without careful planning and support, there's a risk that IBL could exacerbate existing educational inequalities rather than mitigate them.
Implementing IBL in the Classroom
Start small.
For educators new to IBL, starting small can help ease the transition. Begin by integrating inquiry-based activities into existing lesson plans or dedicating a portion of class time to student-directed projects. This approach allows both teachers and students to become comfortable with the IBL process without overwhelming them.
Create a Supportive Classroom Environment
A supportive and safe classroom environment is crucial for the success of IBL. Encourage curiosity and value all questions, fostering a culture where students feel comfortable exploring ideas and taking intellectual risks. Establishing norms for collaboration and respectful discourse can also enhance the effectiveness of group work.
Use Open-Ended Questions
Open-ended questions are the backbone of IBL, stimulating critical thinking and exploration. Design questions that cannot be answered with a simple "yes" or "no," and that require students to apply, analyze, and synthesize information. These questions should be aligned with learning objectives and designed to provoke curiosity and deeper inquiry.
Facilitate, Don't Dictate
In IBL, the teacher's role shifts from the primary source of knowledge to a facilitator of learning . Provide guidance and resources to help students navigate their inquiries, but resist the urge to provide immediate answers to questions. Encourage students to find solutions independently or collaboratively, fostering independence and resilience.
Incorporate a Variety of Resources
IBL thrives on the use of diverse resources, including digital tools, library materials, expert interviews, and field trips. Encourage students to look beyond textbooks, leveraging technology and community resources to gather information and insights related to their inquiries.
Scaffold the Inquiry Process
While the ultimate goal of IBL is for students to conduct independent inquiries, initially, they may need significant support. Scaffold the process by breaking it down into manageable steps, providing tools and strategies for each stage. As students become more skilled in navigating the inquiry process, gradually reduce the level of scaffolding.
Assess Process and Product
Assessment in IBL should focus on both the process and the product of learning. Use formative assessments to provide feedback during the inquiry process, and summative assessments to evaluate the final outcomes. Consider employing a variety of assessment methods, including portfolios, presentations, reflective journals, and peer evaluations, to capture the full extent of student learning.
Reflect and Revise
Reflection is a key component of IBL for both students and teachers. Encourage students to reflect on their learning process, challenges faced, and knowledge gained. Teachers should also reflect on the effectiveness of IBL activities, seeking student feedback and making adjustments as necessary to improve future inquiries.
Conclusion and Future of IBL
Inquiry-Based Learning offers a transformative approach to education, preparing students with the skills needed for the modern world. Despite its challenges, the future of IBL is promising, with ongoing developments in educational technology and research supporting its effectiveness. Embracing IBL represents a commitment to an inclusive, adaptive, and student-centered educational philosophy.
Create engaging and interactive courses at scale. Start today.
.png)
Related learning terms
Discover the power of Explicit Knowledge and its role in your organizational growth. Unveil the assets of knowledge management today.
Learn about the benefits, steps to take, and ways to find opportunities to stay current in your field and advance your career.
Compliance training teaches employees about laws, regulations, and company policies to ensure ethical behavior, protect customer information and safety, and avoid legal consequences.
Employee onboarding is the process by which we help new hires become familiar with our organization and start working productively.
We empower online academies to launch engaging learning experiences and improve learning outcomes through our social learning platform.
- White Label LMS
- LMS for Nonprofits
- Integrations
- Comparisons
- eLearning Glossary
- Request a demo
- Cohort-based learning
- Collaborative learning
- Corporate training
- Capability academy
- Group coaching
- Course creators platform
- Community-based learning
- Free course pricing calculator
- Free course revenues calculator
- Privacy Policy
- System Status

The 5 Phases of Inquiry-Based Learning
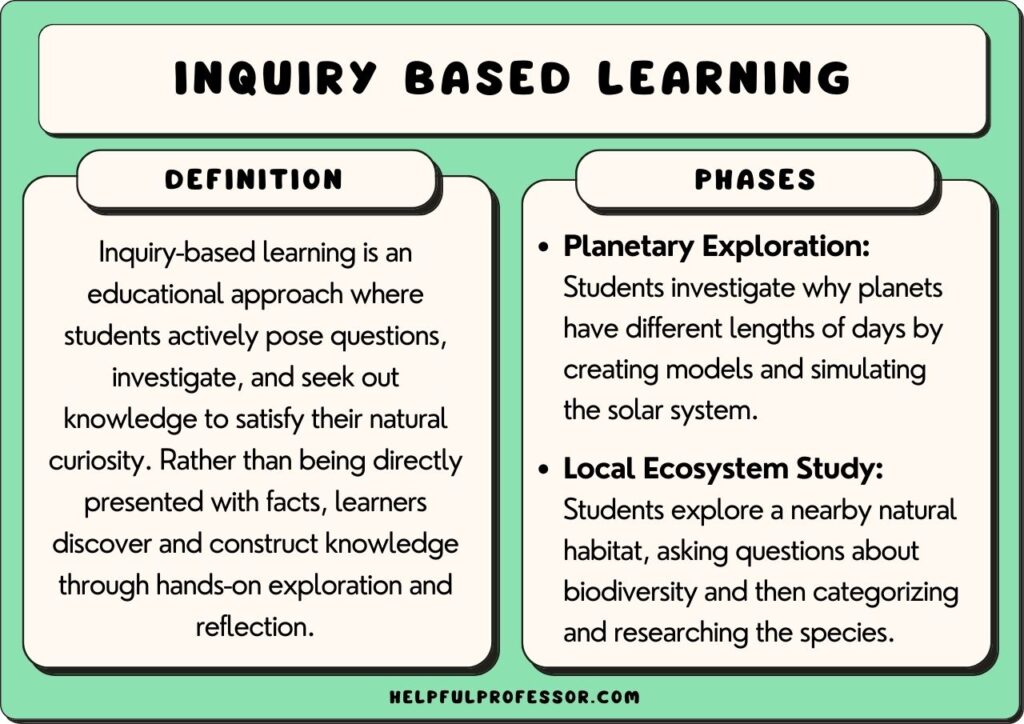
Inquiry based learning places an emphasis on finding answers through discovery. It is contrasted to educational approaches that privilege repetition and memorization.
An inquiry-based approach will ask students to investigate concepts using research and analysis. Its greatest benefit is that it requires the use of higher-order thinking skills such as problem solving to reach conclusions.
There are five phases to an inquiry based learning approach. The 5 phases are:
- Orientation – discovering the problem.
- Conceptualization – finding a research question.
- Investigation – collecting evidence and information.
- Conclusion – analyzing the evidence and making up your mind.
- Discussion – exploring the implications of your new knowledge.
Use these five phases in lesson plans and college papers to demonstrate your depth of knowledge of how inquiry-based learning works.
1. Orientation
In the orientation phase, teachers present the students with a topic. The teacher will provide the foundations that are required to understand the topic. The teacher may also define a problem that needs to be solved.
Teachers start an inquiry based learning session by presenting a topic in an engaging or inspiring way.
In this stage, the teacher is the ‘provocateur’. Their job is to build engagement, excitement and curiosity.
An orientation may be a video, presentation from a guest speaker, or a book. Sometimes, I’ll present my students with a cartoon, article from a local newspaper, or provocative YouTube video.
The key here is to simultaneously motivate the students to learn and give the foundations for follow-up inquiry.
Grade 3 teacher Joanne has a curriculum outcome about “arachnids” that she needs to achieve. Joanne presents her students with a range of photos of mites, ticks, spiders and scorpions. She asks the students: what do all these creatures have in common? Answers could include: they all have eight legs, they’re all insects (she will need to correct this misconception during the inquiry phase), and they are all probably poisonous. The class has a discussion about how they feel about arachnids: they’re gross, interesting, and maybe a little scary.
Go Deeper: 18 Examples of Inquiry-Based Learning
2. Conceptualization
In the conceptualization stage, students come up with a plan for how they might learn more about their topic. Teachers might ask students to come up with a research question and develop an action plan for investigating the question.
During the conceptualization stage, the teacher works on releasing some control over the lesson’s direction to the students. The teacher uses the students’ questions and comments from Phase 1 to stimulate a round of question-posing discussion. The teacher might ask the students to present their own questions. Or, if a stringent curriculum outcome needs to be met, the teacher may present a question of her own.
Central to this phase is the development of a question or problem. Without a question, there would be no subsequent inquiry.
Once an inquiry question is in place (either posed by the students or teacher), the teacher should require the students to come up with an action plan to address the question. One common way to do this is to get students to break-up into groups and come up with an action plan: How will we answer this question? What resources will we need? What are the steps that we need to take?
Grade 3 teacher Joanne asks her students what questions they have about arachnids. Examples of questions might be: Do they all have eight legs? Are they all poisonous? Which arachnids are found in our local area? How many eyes do they have? As the students present their questions, Joanne writes them on the whiteboard at the front of the class.
Joanne then asks her students to break up into groups of 6 and come up with a plan-of-action for answering these questions. Group 1 decides they want to create a poster answering the questions presented. They request iPads for internet research. Group 2 decides they want to create a blog about arachnids. They request an expert from the local zoo comes to show them real-life arachnids in the classroom.
3. Investigation
In the investigation phase, students will actively explore the topic through research, experimentation, exploration, observation and data collection. They should also record their data for follow-up reflection.
The investigation phases should be a student-centered phase in which the students actively seek out answers. Students use the procedures they put together in Phase 2 to conduct their inquiry. The role of the teacher is to facilitate this exploration, gather the required resources, and facilitate group discussion.
Some subject-specific examples may be:
- In chemistry class, this phase often involves conducting controlled experiments.
- In history class, it may involve going to a local historical site and examining landmarks or buildings of interest.
- In physics class, it may involve engineering an experiment to observe gravity.
Upon suggestion from her students, Joanne invites a local zoologist to bring in samples of arachnids for students to investigate. The zoologist places them in glass cages in a row on a table. The students bring their questions like “Do all arachnids have 8 legs?” and “How many eyes do they have?” and see if they can answer them through observation. For smaller arachnids, students get the chance to look at them through the classroom’s microscopes. Following observation, further questions are asked of the expert, such as questions about arachnids from the local area.
4. Conclusion
During the conclusion phase, students should look at the data they collected and see what new answers they have. Students should look at the research question they devised in Phase 2 and see if they can now answer it.
The conclusion phase is an opportunity to reflect on the data collected and analyze it. Students should get together and compare their findings to the research question. Have they answered the questions they set before starting the inquiry? How can they present this information in new ways? What new questions do they have?
This phase may end with presenting a new hypothesis, statement of belief, or a model for explaining a phenomenon.
With older students, you could use phrases like: ‘data organization’, ‘synthesizing’, ‘generating a hypothesis’, and ‘data interpretation’.
After the expert leaves, the students get together in groups and compare notes. Together, they check to make sure there are no discrepancies in their findings (and discuss those discrepancies to see if solutions can be found). Then, they create posters presenting their findings.
5. Discussion
In the discussion phase, students should explore the implications of their new knowledge. Questions should include: What does this mean? How does this impact my beliefs and understanding? How can I use this knowledge in the future?
Discussions at the end of an inquiry should have students thinking forward. The teacher facilitates this discussion and asks prying questions that stimulate higher-order thinking .
Students should be asked how their thinking, beliefs or conceptions about the topic have changed. A good question might be: “How does this new knowledge change your feelings about this topic?” or “What is something that surprised you during this investigation?”
Students should also talk about the implications of their new knowledge for the world beyond the classroom. If it’s a study of gravity, consider getting students to talk about how this knowledge is important for building stable bridges. If it’s a study of precipitation, you could talk about how your new knowledge is valuable for farmers who want to drought-proof their business.
Finally, ensure students leave with more questions. There are always new questions that emerge when we push our horizons of knowledge. Have students leave the class inspired to learn more.
Joanne gets her class together after they have presented their posters to discuss what they now know about arachnids. She presents forward-thinking questions like: Have your opinions of arachnids changed now? Have you seen any arachnids around the local area since we started this investigation? Do you think you can now tell the difference between an arachnid and an insect when looking at them now?
Definitions
Here are some great, high-quality definitions of Inquiry Based Learning from scholarly sources. Use these in your essay!
- “Inquiry-based learning is an approach to teaching and learning that places students’ questions, ideas and observations at the center of the learning experience” (Ontario Ministry of Education, 2013, p. 2).
- “Inquiry-based learning is an educational strategy in which students follow methods and practices similar to those of professional scientists in order to construct knowledge. It can be defined as a process of discovering new causal relations, with the learner formulating hypotheses and testing them by conducting experiments and/or making observations” (Pedaste et al., 2015, p. 47).
Theoretical Foundations
Inquiry based learning is based on the constructivist learning theory .
Constructivists believe that we learn through actively ‘mulling over’ information in our minds. We use our logic and reason to come to conclusions about topics.
This is in contrast to theories like behaviorism which believe we should be told information from a teacher rather than learning it ourselves through our experiences.
Central to a constructivist approach is the idea of learning through ‘doing’ in a student-centered environment (as opposed to passive learning in a teacher-centered environment).
Final Thoughts
Inquiry learning is one of the most popular approaches to education in the 21st Century . It promotes student-centered learning , problem-solving and creative thinking . It’s a strategy that not only teaches content but also thinking skills.
Unfortunately many of my college students write essays about inquiry based learning in really vague terms.
Often, students don’t write about any clear, actionable ways to apply the idea in classrooms. Instead, we need clear and actionable steps to ensure inquiry occur in the classroom. That’s why I encourage my college students to employ the five phases of inquiry when applying IBL in the classroom.
Beyrow, M., Godau, M., Heidmann, F., Langer, C., Wettach, R., & Mieg, H. (2019). Inquiry-Based Learning in Design. Inquiry-Based Learning – Undergraduate Research (pp. 239-247). https://doi.org/10.1007/978-3-030-14223-0_22
Ernst, Dana & Hodge, Angie & Yoshinobu, Stan. (2017). What Is Inquiry-Based Learning? Notices of the American Mathematical Society, 64 . 570-574. https://doi.org/10.1090/noti1536
Ontario Department of Education. (2013). Inquiry-Based Learning . Retrieved from: http://www.edu.gov.on.ca/eng/literacynumeracy/inspire/research/CBS_InquiryBased.pdf
Pedaste, M., Mäeots, M., Siiman, L. A., De Jong, T., Van Riesen, S. A., Kamp, E. T., … & Tsourlidaki, E. (2015). Phases of inquiry-based learning: Definitions and the inquiry cycle. Educational research review , 14 , 47-61. ( access here )
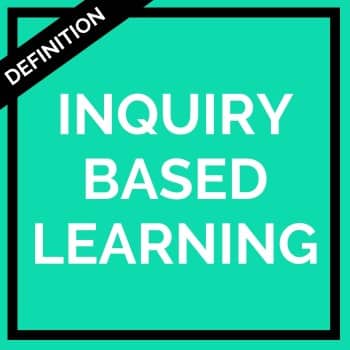
Chris Drew (PhD)
Dr. Chris Drew is the founder of the Helpful Professor. He holds a PhD in education and has published over 20 articles in scholarly journals. He is the former editor of the Journal of Learning Development in Higher Education. [Image Descriptor: Photo of Chris]
- Chris Drew (PhD) https://helpfulprofessor.com/author/chris-drew-phd/ 5 Top Tips for Succeeding at University
- Chris Drew (PhD) https://helpfulprofessor.com/author/chris-drew-phd/ 50 Durable Goods Examples
- Chris Drew (PhD) https://helpfulprofessor.com/author/chris-drew-phd/ 100 Consumer Goods Examples
- Chris Drew (PhD) https://helpfulprofessor.com/author/chris-drew-phd/ 30 Globalization Pros and Cons
Leave a Comment Cancel Reply
Your email address will not be published. Required fields are marked *

An official website of the United States government
The .gov means it’s official. Federal government websites often end in .gov or .mil. Before sharing sensitive information, make sure you’re on a federal government site.
The site is secure. The https:// ensures that you are connecting to the official website and that any information you provide is encrypted and transmitted securely.
- Publications
- Account settings
Preview improvements coming to the PMC website in October 2024. Learn More or Try it out now .
- Advanced Search
- Journal List
- Springer Nature - PMC COVID-19 Collection

STEM Problem Solving: Inquiry, Concepts, and Reasoning
Aik-ling tan.
Natural Sciences and Science Education, meriSTEM@NIE, National Institute of Education, Nanyang Technological University, Singapore, Singapore
Yann Shiou Ong
Yong sim ng, jared hong jie tan, associated data.
The datasets used and analysed during the current study are available from the corresponding author on reasonable request.
Balancing disciplinary knowledge and practical reasoning in problem solving is needed for meaningful learning. In STEM problem solving, science subject matter with associated practices often appears distant to learners due to its abstract nature. Consequently, learners experience difficulties making meaningful connections between science and their daily experiences. Applying Dewey’s idea of practical and science inquiry and Bereiter’s idea of referent-centred and problem-centred knowledge, we examine how integrated STEM problem solving offers opportunities for learners to shuttle between practical and science inquiry and the kinds of knowledge that result from each form of inquiry. We hypothesize that connecting science inquiry with practical inquiry narrows the gap between science and everyday experiences to overcome isolation and fragmentation of science learning. In this study, we examine classroom talk as students engage in problem solving to increase crop yield. Qualitative content analysis of the utterances of six classes of 113 eighth graders and their teachers were conducted for 3 hours of video recordings. Analysis showed an almost equal amount of science and practical inquiry talk. Teachers and students applied their everyday experiences to generate solutions. Science talk was at the basic level of facts and was used to explain reasons for specific design considerations. There was little evidence of higher-level scientific conceptual knowledge being applied. Our observations suggest opportunities for more intentional connections of science to practical problem solving, if we intend to apply higher-order scientific knowledge in problem solving. Deliberate application and reference to scientific knowledge could improve the quality of solutions generated.
Introduction
As we enter to second quarter of the twenty-first century, it is timely to take stock of both the changes and demands that continue to weigh on our education system. A recent report by World Economic Forum highlighted the need to continuously re-position and re-invent education to meet the challenges presented by the disruptions brought upon by the fourth industrial revolution (World Economic Forum, 2020 ). There is increasing pressure for education to equip children with the necessary, relevant, and meaningful knowledge, skills, and attitudes to create a “more inclusive, cohesive and productive world” (World Economic Forum, 2020 , p. 4). Further, the shift in emphasis towards twenty-first century competencies over mere acquisition of disciplinary content knowledge is more urgent since we are preparing students for “jobs that do not yet exist, technology that has not yet been invented, and problems that has yet exist” (OECD, 2018 , p. 2). Tan ( 2020 ) concurred with the urgent need to extend the focus of education, particularly in science education, such that learners can learn to think differently about possibilities in this world. Amidst this rhetoric for change, the questions that remained to be answered include how can science education transform itself to be more relevant; what is the role that science education play in integrated STEM learning; how can scientific knowledge, skills and epistemic practices of science be infused in integrated STEM learning; what kinds of STEM problems should we expose students to for them to learn disciplinary knowledge and skills; and what is the relationship between learning disciplinary content knowledge and problem solving skills?
In seeking to understand the extent of science learning that took place within integrated STEM learning, we dissected the STEM problems that were presented to students and examined in detail the sense making processes that students utilized when they worked on the problems. We adopted Dewey’s ( 1938 ) theoretical idea of scientific and practical/common-sense inquiry and Bereiter’s ideas of referent-centred and problem-centred knowledge building process to interpret teacher-students’ interactions during problem solving. There are two primary reasons for choosing these two theoretical frameworks. Firstly, Dewey’s ideas about the relationship between science inquiry and every day practical problem-solving is important in helping us understand the role of science subject matter knowledge and science inquiry in solving practical real-world problems that are commonly used in STEM learning. Secondly, Bereiter’s ideas of referent-centred and problem-centred knowledge augment our understanding of the types of knowledge that students can learn when they engage in solving practical real-world problems.
Taken together, Dewey’s and Bereiter’s ideas enable us to better understand the types of problems used in STEM learning and their corresponding knowledge that is privileged during the problem-solving process. As such, the two theoretical lenses offered an alternative and convincing way to understand the actual types of knowledge that are used within the context of integrated STEM and help to move our understanding of STEM learning beyond current focus on examining how engineering can be used as an integrative mechanism (Bryan et al., 2016 ) or applying the argument of the strengths of trans-, multi-, or inter-disciplinary activities (Bybee, 2013 ; Park et al., 2020 ) or mapping problems by the content and context as pure STEM problems, STEM-related problems or non-STEM problems (Pleasants, 2020 ). Further, existing research (for example, Gale et al., 2000 ) around STEM education focussed largely on description of students’ learning experiences with insufficient attention given to the connections between disciplinary conceptual knowledge and inquiry processes that students use to arrive at solutions to problems. Clarity in the role of disciplinary knowledge and the related inquiry will allow for more intentional design of STEM problems for students to learn higher-order knowledge. Applying Dewey’s idea of practical and scientific inquiry and Bereiter’s ideas of referent-centred and problem-centred knowledge, we analysed six lessons where students engaged with integrated STEM problem solving to propose answers to the following research questions: What is the extent of practical and scientific inquiry in integrated STEM problem solving? and What conceptual knowledge and problem-solving skills are learnt through practical and science inquiry during integrated STEM problem solving?
Inquiry in Problem Solving
Inquiry, according to Dewey ( 1938 ), involves the direct control of unknown situations to change them into a coherent and unified one. Inquiry usually encompasses two interrelated activities—(1) thinking about ideas related to conceptual subject-matter and (2) engaging in activities involving our senses or using specific observational techniques. The National Science Education Standards released by the National Research Council in the US in 1996 defined inquiry as “…a multifaceted activity that involves making observations; posing questions; examining books and other sources of information to see what is already known; planning investigations; reviewing what is already known in light of experimental evidence; using tools to gather, analyze, and interpret data; proposing answers, explanations, and predictions; and communicating the results. Inquiry requires identification of assumptions, use of critical and logical thinking, and consideration of alternative explanations” (p. 23). Planning investigation; collecting empirical evidence; using tools to gather, analyse and interpret data; and reasoning are common processes shared in the field of science and engineering and hence are highly relevant to apply to integrated STEM education.
In STEM education, establishing the connection between general inquiry and its application helps to link disciplinary understanding to epistemic knowledge. For instance, methods of science inquiry are popular in STEM education due to the familiarity that teachers have with scientific methods. Science inquiry, a specific form of inquiry, has appeared in many science curriculum (e.g. NRC, 2000 ) since Dewey proposed in 1910 that learning of science should be perceived as both subject-matter and a method of learning science (Dewey, 1910a , 1910b ). Science inquiry which involved ways of doing science should also encompass the ways in which students learn the scientific knowledge and investigative methods that enable scientific knowledge to be constructed. Asking scientifically orientated questions, collecting empirical evidence, crafting explanations, proposing models and reasoning based on available evidence are affordances of scientific inquiry. As such, science should be pursued as a way of knowing rather than merely acquisition of scientific knowledge.
Building on these affordances of science inquiry, Duschl and Bybee ( 2014 ) advocated the 5D model that focused on the practice of planning and carrying out investigations in science and engineering, representing two of the four disciplines in STEM. The 5D model includes science inquiry aspects such as (1) deciding on what and how to measure, observe and sample; (2) developing and selecting appropriate tools to measure and collect data; (3) recording the results and observations in a systematic manner; (4) creating ways to represent the data and patterns that are observed; and (5) determining the validity and the representativeness of the data collected. The focus on planning and carrying out investigations in the 5D model is used to help teachers bridge the gap between the practices of building and refining models and explanation in science and engineering. Indeed, a common approach to incorporating science inquiry in integrated STEM curriculum involves student planning and carrying out scientific investigations and making sense of the data collected to inform engineering design solution (Cunningham & Lachapelle, 2016 ; Roehrig et al., 2021 ). Duschl and Bybee ( 2014 ) argued that it is needful to design experiences for learners to appreciate that struggles are part of problem solving in science and engineering. They argued that “when the struggles of doing science is eliminated or simplified, learners get the wrong perceptions of what is involved when obtaining scientific knowledge and evidence” (Duschl & Bybee, 2014 , p. 2). While we concur with Duschl and Bybee about the need for struggles, in STEM learning, these struggles must be purposeful and grade appropriate so that students will also be able to experience success amidst failure.
The peculiar nature of science inquiry was scrutinized by Dewey ( 1938 ) when he cross-examined the relationship between science inquiry and other forms of inquiry, particularly common-sense inquiry. He positioned science inquiry along a continuum with general or common-sense inquiry that he termed as “logic”. Dewey argued that common-sense inquiry serves a practical purpose and exhibits features of science inquiry such as asking questions and a reliance on evidence although the focus of common-sense inquiry tends to be different. Common-sense inquiry deals with issues or problems that are in the immediate environment where people live, whereas the objects of science inquiry are more likely to be distant (e.g. spintronics) from familiar experiences in people’s daily lives. While we acknowledge the fundamental differences (such as novel discovery compared with re-discovering science, ‘messy’ science compared with ‘sanitised’ science) between school science and science that is practiced by scientists, the subject of interest in science (understanding the world around us) remains the same.
The unfamiliarity between the functionality and purpose of science inquiry to improve the daily lives of learners does little to motivate learners to learn science (Aikenhead, 2006 ; Lee & Luykx, 2006 ) since learners may not appreciate the connections of science inquiry in their day-to-day needs and wants. Bereiter ( 1992 ) has also distinguished knowledge into two forms—referent-centred and problem-centred. Referent-centred knowledge refers to subject-matter that is organised around topics such as that in textbooks. Problem-centred knowledge is knowledge that is organised around problems, whether they are transient problems, practical problems or problems of explanations. Bereiter argued that referent-centred knowledge that is commonly taught in schools is limited in their applications and meaningfulness to the lives of students. This lack of familiarity and affinity to referent-centred knowledge is likened to the science subject-matter knowledge that was mentioned by Dewey. Rather, it is problem-centred knowledge that would be useful when students encounter problems. Learning problem-centred knowledge will allow learners to readily harness the relevant knowledge base that is useful to understand and solve specific problems. This suggests a need to help learners make the meaningful connections between science and their daily lives.
Further, Dewey opined that while the contexts in which scientific knowledge arise could be different from our daily common-sense world, careful consideration of scientific activities and applying the resultant knowledge to daily situations for use and enjoyment is possible. Similarly, in arguing for problem-centred knowledge, Bereiter ( 1992 ) questioned the value of inert knowledge that plays no role in helping us understand or deal with the world around us. Referent-centred knowledge has a higher tendency to be inert due to the way that the knowledge is organised and the way that the knowledge is encountered by learners. For instance, learning about the equation and conditions for photosynthesis is not going to help learners appreciate how plants are adapted for photosynthesis and how these adaptations can allow plants to survive changes in climate and for farmers to grow plants better by creating the best growing conditions. Rather, students could be exposed to problems of explanations where they are asked to unravel the possible reasons for low crop yield and suggest possible ways to overcome the problem. Hence, we argue here that the value of the referent knowledge is that they form the basis and foundation for the students to be able to discuss or suggest ways to overcome real life problems. Referent-centred knowledge serves as part of the relevant knowledge base that can be harnessed to solve specific problems or as foundational knowledge students need to progress to learn higher-order conceptual knowledge that typically forms the foundations or pillars within a discipline. This notion of referent-centred knowledge serving as foundational knowledge that can be and should be activated for application in problem-solving situation is shown by Delahunty et al. ( 2020 ). They found that students show high reliance on memory when they are conceptualising convergent problem-solving tasks.
While Bereiter argues for problem-centred knowledge, he cautioned that engagement should be with problems of explanation rather than transient or practical problems. He opined that if learners only engage in transient or practical problem alone, they will only learn basic-category types of knowledge and fail to understand higher-order conceptual knowledge. For example, for photosynthesis, basic-level types of knowledge included facts about the conditions required for photosynthesis, listing the products formed from the process of photosynthesis and knowing that green leaves reflect green light. These basic-level knowledges should intentionally help learners learn higher-level conceptual knowledge that include learners being able to draw on the conditions for photosynthesis when they encounter that a plant is not growing well or is exhibiting discoloration of leaves.
Transient problems disappear once a solution becomes available and there is a high likelihood that we will not remember the problem after that. Practical problems, according to Bereiter are “stuck-door” problems that could be solved with or without basic-level knowledge and often have solutions that lacks precise definition. There are usually a handful of practical strategies, such as pulling or pushing the door harder, kicking the door, etc. that will work for the problems. All these solutions lack a well-defined approach related to general scientific principles that are reproducible. Problems of explanations are the most desirable types of problems for learners since these are problems that persist and recur such that they can become organising points for knowledge. Problems of explanations consist of the conceptual representations of (1) a text base that serves to represent the text content and (2) a situation model that shows the portion of the world in which the text is relevant. The idea of text base to represent text content in solving problems of explanations is like the idea of domain knowledge and structural knowledge (refers to knowledge of how concepts within a domain are connected) proposed by Jonassen ( 2000 ). He argued that both types of knowledges are required to solve a range of problems from well-structured problems to ill-structured problems with a simulated context, to simple ill-structured problems and to complex ill-structured problems.
Jonassen indicated that complex ill-structured problems are typically design problems and are likely to be the most useful forms of problems for learners to be engaged in inquiry. Complex ill-structured design problems are the “wicked” problems that Buchanan ( 1992 ) discussed. Buchanan’s idea is that design aims to incorporate knowledge from different fields of specialised inquiry to become whole. Complex or wicked problems are akin to the work of scientists who navigate multiple factors and evidence to offer models that are typically oversimplified, but they apply them to propose possible first approximation explanations or solutions and iteratively relax constraints or assumptions to refine the model. The connections between the subject matter of science and the design process to engineer a solution are delicate. While it is important to ensure that practical concerns and questions are taken into consideration in designing solutions (particularly a material artefact) to a practical problem, the challenge here lies in ensuring that creativity in design is encouraged even if students initially lack or neglect the scientific conceptual understanding to explain/justify their design. In his articulation of wicked problems and the role of design thinking, Buchanan ( 1992 ) highlighted the need to pay attention to category and placement. Categories “have fixed meanings that are accepted within the framework of a theory or a philosophy and serve as the basis for analyzing what already exist” (Buchanan, 1992 , p. 12). Placements, on the other hand, “have boundaries to shape and constrain meaning, but are not rigidly fixed and determinate” (p. 12).
The difference in the ideas presented by Dewey and Bereiter lies in the problem design. For Dewey, scientific knowledge could be learnt from inquiring into practical problems that learners are familiar with. After all, Dewey viewed “modern science as continuous with, and to some degree an outgrowth and refinement of, practical or ‘common-sense’ inquiry” (Brown, 2012 ). For Bereiter, he acknowledged the importance of familiar experiences, but instead of using them as starting points for learning science, he argued that practical problems are limiting in helping learners acquire higher-order knowledge. Instead, he advocated for learners to organize their knowledge around problems that are complex, persistent and extended and requiring explanations to better understand the problems. Learners are to have a sense of the kinds of problems to which the specific concept is relevant before they can be said to have grasp the concept in a functionally useful way.
To connect between problem solving, scientific knowledge and everyday experiences, we need to examine ways to re-negotiate the disciplinary boundaries (such as epistemic understanding, object of inquiry, degree of precision) of science and make relevant connections to common-sense inquiry and to the problem at hand. Integrated STEM appears to be one way in which the disciplinary boundaries of science can be re-negotiated to include practices from the fields of technology, engineering and mathematics. In integrated STEM learning, inquiry is seen more holistically as a fluid process in which the outcomes are not absolute but are tentative. The fluidity of the inquiry process is reflected in the non-deterministic inquiry approach. This means that students can use science inquiry, engineering design, design process or any other inquiry approaches that fit to arrive at the solution. This hybridity of inquiry between science, common-sense and problems allows for some familiar aspects of the science inquiry process to be applied to understand and generate solutions to familiar everyday problems. In attempting to infuse elements of common-sense inquiry with science inquiry in problem-solving, logic plays an important role to help learners make connections. Hypothetically, we argue that with increasing exposure to less familiar ways of thinking such as those associated with science inquiry, students’ familiarity with scientific reasoning increases, and hence such ways of thinking gradually become part of their common-sense, which students could employ to solve future relevant problems. The theoretical ideas related to complexities of problems, the different forms of inquiry afforded by different problems and the arguments for engaging in problem solving motivated us to examine empirically how learners engage with ill-structured problems to generate problem-centred knowledge. Of particular interest to us is how learners and teachers weave between practical and scientific reasoning as they inquire to integrate the components in the original problem into a unified whole.
The integrated STEM activity in our study was planned using the S-T-E-M quartet instructional framework (Tan et al., 2019 ). The S-T-E-M quartet instructional framework positions complex, persistent and extended problems at its core and focusses on the vertical disciplinary knowledge and understanding of the horizontal connections between the disciplines that could be gained by learners through solving the problem (Tan et al., 2019 ). Figure 1 depicts the disciplinary aspects of the problem that was presented to the students. The activity has science and engineering as the two lead disciplines. It spanned three 1-h lessons and required students to both learn and apply relevant scientific conceptual knowledge to solve a complex, real-world problem through processes that resemble the engineering design process (Wheeler et al., 2019 ).

Connections across disciplines in integrate STEM activity
In the first session (1 h), students were introduced to the problem and its context. The problem pertains to the issue of limited farmland in a land scarce country that imports 90% of food (Singapore Food Agency [SFA], 2020 ). The students were required to devise a solution by applying knowledge of the conditions required for photosynthesis and plant growth to design and build a vertical farming system to help farmers increase crop yield with limited farmland. This context was motivated by the government’s effort to generate interests and knowledge in farming to achieve the 30 by 30 goal—supplying 30% of country’s nutritional needs by 2030. The scenario was a fictitious one where they were asked to produce 120 tonnes of Kailan (a type of leafy vegetable) with two hectares of land instead of the usual six hectares over a specific period. In addition to the abovementioned constraints, the teacher also discussed relevant success criteria for evaluating the solution with the students. Students then researched about existing urban farming approaches. They were given reading materials pertaining to urban farming to help them understand the affordances and constraints of existing solutions. In the second session (6 h), students engaged in ideation to generate potential solutions. They then designed, built and tested their solution and had opportunities to iteratively refine their solution. Students were given a list of materials (e.g. mounting board, straws, ice-cream stick, glue, etc.) that they could use to design their solutions. In the final session (1 h), students presented their solution and reflected on how well their solution met the success criteria. The prior scientific conceptual knowledge that students require to make sense of the problem include knowledge related to plant nutrition, namely, conditions for photosynthesis, nutritional requirements of Kailin and growth cycle of Kailin. The problem resembles a real-world problem that requires students to engage in some level of explanation of their design solution.
A total of 113 eighth graders (62 boys and 51 girls), 14-year-olds, from six classes and their teachers participated in the study. The students and their teachers were recruited as part of a larger study that examined the learning experiences of students when they work on integrated STEM activities that either begin with a problem, a solution or are focused on the content. Invitations were sent to schools across the country and interested schools opted in for the study. For the study reported here, all students and teachers were from six classes within a school. The teachers had all undergone 3 h of professional development with one of the authors on ways of implementing the integrated STEM activity used in this study. During the professional development session, the teachers learnt about the rationale of the activity, familiarize themselves with the materials and clarified the intentions and goals of the activity. The students were mostly grouped in groups of three, although a handful of students chose to work independently. The group size of students was not critical for the analysis of talk in this study as the analytic focus was on the kinds of knowledge applied rather than collaborative or group think. We assumed that the types of inquiry adopted by teachers and students were largely dependent on the nature of problem. Eighth graders were chosen for this study since lower secondary science offered at this grade level is thematic and integrated across biology, chemistry and physics. Furthermore, the topic of photosynthesis is taught under the theme of Interactions at eighth grade (CPDD, 2021 ). This thematic and integrated nature of science at eighth grade offered an ideal context and platform for integrated STEM activities to be trialled.
The final lessons in a series of three lessons in each of the six classes was analysed and reported in this study. Lessons where students worked on their solutions were not analysed because the recordings had poor audibility due to masking and physical distancing requirements as per COVID-19 regulations. At the start of the first lesson, the instructions given by the teacher were:
You are going to present your models. Remember the scenario that you were given at the beginning that you were tasked to solve using your model. …. In your presentation, you have to present your prototype and its features, what is so good about your prototype, how it addresses the problem and how it saves costs and space. So, this is what you can talk about during your presentation. ….. pay attention to the presentation and write down questions you like to ask the groups after the presentation… you can also critique their model, you can evaluate, critique and ask questions…. Some examples of questions you can ask the groups are? Do you think your prototype can achieve optimal plant growth? You can also ask questions specific to their models.
Data collection
Parental consent was sought a month before the start of data collection. The informed consent adhered to confidentiality and ethics guidelines as described by the Institutional Review Board. The data collection took place over a period of one month with weekly video recording. Two video cameras, one at the front and one at the back of the science laboratory were set up. The front camera captured the students seated at the front while the back video camera recorded the teacher as well as the groups of students at the back of the laboratory. The video recordings were synchronized so that the events captured from each camera can be interpreted from different angles. After transcription of the raw video files, the identities of students were substituted with pseudonyms.
Data analysis
The video recordings were analysed using the qualitative content analysis approach. Qualitative content analysis allows for patterns or themes and meanings to emerge from the process of systematic classification (Hsieh & Shannon, 2005 ). Qualitative content analysis is an appropriate analytic method for this study as it allows us to systematically identify episodes of practical inquiry and science inquiry to map them to the purposes and outcomes of these episodes as each lesson unfolds.
In total, six h of video recordings where students presented their ideas while the teachers served as facilitator and mentor were analysed. The video recordings were transcribed, and the transcripts were analysed using the NVivo software. Our unit of analysis is a single turn of talk (one utterance). We have chosen to use utterances as proxy indicators of reasoning practices based on the assumption that an utterance relates to both grammar and context. An utterance is a speech act that reveals both meaning and intentions of the speaker within specific contexts (Li, 2008 ).
Our research analytical lens is also interpretative in nature and the validity of our interpretation is through inter-rater discussion and agreement. Each utterance at the speaker level in transcripts was examined and coded either as relevant to practical reasoning or scientific reasoning based on the content. The utterances could be a comment by the teacher, a question by a student or a response by another student. Deductive coding is deployed with the two codes, practical reasoning and scientific reasoning derived from the theoretical ideas of Dewey and Bereiter as described earlier. Practical reasoning refers to utterances that reflect commonsensical knowledge or application of everyday understanding. Scientific reasoning refers to utterances that consist of scientifically oriented questions, scientific terms, or the use of empirical evidence to explain. Examples of each type of reasoning are highlighted in the following section. Each coded utterance is then reviewed for detailed description of the events that took place that led to that specific utterance. The description of the context leading to the utterance is considered an episode. The episodes and codes were discussed and agreed upon by two of the authors. Two coders simultaneously watched the videos to identify and code the episodes. The coders interpreted the content of each utterance, examine the context where the utterance was made and deduced the purpose of the utterance. Once each coder has established the sense-making aspect of the utterance in relation to the context, a code of either practical reasoning or scientific reasoning is assigned. Once that was completed, the two coders compared their coding for similarities and differences. They discussed the differences until an agreement was reached. Through this process, an agreement of 85% was reached between the coders. Where disagreement persisted, codes of the more experienced coder were adopted.
Results and Discussion
The specific STEM lessons analysed were taken from the lessons whereby students presented the model of their solutions to the class for peer evaluation. Every group of students stood in front of the class and placed their model on the bench as they presented. There was also a board where they could sketch or write their explanations should they want to. The instructions given by the teacher to the students were to explain their models and state reasons for their design.
Prevalence of Reasoning
The 6h of videos consists of 1422 turns of talk. Three hundred four turns of talk (21%) were identified as talk related to reasoning, either practical reasoning or scientific reasoning. Practical reasoning made up 62% of the reasoning turns while 38% were scientific reasoning (Fig. (Fig.2 2 ).

Frequency of different types of reasoning
The two types of reasoning differ in the justifications that are used to substantiate the claims or decisions made. Table Table1 1 describes the differences between the two categories of reasoning.
Types of reasoning used in the integrated STEM activity
Applications of Scientific Reasoning
Instances of engagement with scientific reasoning (for instance, using scientific concepts to justify, raising scientifically oriented questions, or providing scientific explanations) revolved around the conditions for photosynthesis and the concept of energy conversion when students were presenting their ideas or when they were questioned by their peers. For example, in explaining the reason for including fish in their plant system, one group of students made connection to cyclical energy transfer: “…so as the roots of the plants submerged in the water, faeces from the fish will be used as fertilizers so that the plant can grow”. The students considered how organic matter that is still trapped within waste materials can be released and taken up by plants to enhance the growth. The application of scientific reasoning made their design one that is innovative and sustainable as evaluated by the teacher. Some students attempted more ecofriendly designs by considering energy efficiencies through incorporating water turbines in their farming systems. They applied the concept of different forms of energy and energy conversion when their peers inquired about their design. The same scientific concepts were explained at different levels of details by different students. At one level, the students explained in a purely descriptive manner of what happens to the different entities in their prototypes, with implied changes to the forms of energy─ “…spins then generates electricity. So right, when the water falls down, then it will spin. The water will fall on the fan blade thing, then it will spin and then it generates electricity. So, it saves electricity, and also saves water”. At another level, students defended their design through an explanation of energy conversion─ “…because when the water flows right, it will convert gravitational potential energy so, when it reaches the bottom, there is not really much gravitational potential energy”. While these instances of applying scientific reasoning indicated that students have knowledge about the scientific phenomena and can apply them to assist in the problem-solving process, we are not able to establish if students understood the science behind how the dynamo works to generate electricity. Students in eighth grade only need to know how a generator works at a descriptive level and the specialized understanding how a dynamo works is beyond the intended learning outcomes at this grade level.
The application of scientific concepts for justification may not always be accurate. For instance, the naïve conception that students have about plants only respiring at night and not in the day surfaced when one group of students tried to justify the growth rates of Kailan─ “…I mean, they cannot be making food 24/7 and growing 24/7. They have nighttime for a reason. They need to respire”. These students do not appreciate that plants respire in the day as well, and hence respiration occurs 24/7. This naïve conception that plants only respire at night is one that is common among learners of biology (e.g. Svandova, 2014 ) since students learn that plant gives off oxygen in the day and takes in oxygen at night. The hasty conclusion to that observation is that plants carry out photosynthesis in the day and respire at night. The relative rates of photosynthesis and respiration were not considered by many students.
Besides naïve conceptions, engagement with scientific ideas to solve a practical problem offers opportunities for unusual and alternative ideas about science to surface. For instance, another group of students explained that they lined up their plants so that “they can take turns to absorb sunlight for photosynthesis”. These students appear to be explaining that the sun will move and depending on the position of the sun, some plants may be under shade, and hence rates of photosynthesis are dependent on the position of the sun. However, this idea could also be interpreted as (1) the students failed to appreciate that sunlight is everywhere, and (2) plants, unlike animals, particularly humans, do not have the concept of turn-taking. These diverse ideas held by students surfaced when students were given opportunities to apply their knowledge of photosynthesis to solve a problem.
Applications of Practical Reasoning
Teachers and students used more practical reasoning during an integrated STEM activity requiring both science and engineering practices as seen from 62% occurrence of practical reasoning compared with 38% for scientific reasoning. The intention of the activity to integrate students’ scientific knowledge related to plant nutrition to engineering practice of building a model of vertical farming system could be the reason for the prevalence of practical reasoning. The practical reasoning used related to structural design considerations of the farming system such as how water, light and harvesting can be carried out in the most efficient manner. Students defended the strengths of designs using logic based on their everyday experiences. In the excerpt below (transcribed verbatim), we see students applied their everyday experiences when something is “thinner” (likely to mean narrower), logically it would save space. Further, to reach a higher level, you use a machine to climb up.
Excerpt 1. “Thinner, more space” Because it is more thinner, so like in terms of space, it’s very convenient. So right, because there is – because it rotates right, so there is this button where you can stop it. Then I also installed steps, so that – because there are certain places you can’t reach even if you stop the – if you stop the machine, so when you stop it and you climb up, and then you see the condition of the plants, even though it costs a lot of labour, there is a need to have an experienced person who can grow plants. Then also, when like – when water reach the plants, cos the plants I want to use is soil-based, so as the water reach the soil, the soil will xxx, so like the water will be used, and then we got like – and then there’s like this filter that will filter like the dirt.
In the examples of practical reasoning, we were not able to identify instances where students and teachers engaged with discussion around trade-off and optimisation. Understanding constraints, trade-offs and optimisations are important ideas in informed design matrix for engineering as suggested by Crismond and Adams ( 2012 ). For instance, utterances such as “everything will be reused”, “we will be saving space”, “it looks very flimsy” or “so that it can contains [sic] the plants” were used. These utterances were made both by students while justifying their own prototypes and also by peers who challenged the design of others. Longer responses involving practical reasoning were made based on common-sense, everyday logic─ “…the product does not require much manpower, so other than one or two supervisors like I said just now, to harvest the Kailan, hence, not too many people need to be used, need to be hired to help supervise the equipment and to supervise the growth”. We infer that the higher instances of utterances related to practical reasoning could be due to the presence of more concrete artefacts that is shown, and the students and teachers were more focused on questioning the structure at hand. This inference was made as instructions given by the teacher at the start of students’ presentation focus largely on the model rather than the scientific concepts or reasoning behind the model.
Intersection Between Scientific and Practical Reasoning
Comparing science subject matter knowledge and problem-solving to the idea of categories and placement (Buchanan, 1992 ), subject matter is analogous to categories where meanings are fixed with well-established epistemic practices and norms. The problem-solving process and design of solutions are likened to placements where boundaries are less rigid, hence opening opportunities for students’ personal experiences and ideas to be presented. Placements allow students to apply their knowledge from daily experiences and common-sense logic to justify decisions. Common-sense knowledge and logic are more accessible, and hence we observe higher frequency of usage. Comparatively, while science subject matter (categories) is also used, it is observed less frequently. This could possibly be due either to less familiarity with the subject matter or lack of appropriate opportunity to apply in practical problem solving. The challenge for teachers during implementation of a STEM problem-solving activity, therefore, lies in the balance of the application of scientific and practical reasoning to deepen understanding of disciplinary knowledge in the context of solving a problem in a meaningful manner.
Our observations suggest that engaging students with practical inquiry tasks with some engineering demands such as the design of modern farm systems offers opportunities for them to convert their personal lived experiences into feasible concrete ideas that they can share in a public space for critique. The peer critique following the sharing of their practical ideas allows for both practical and scientific questions to be asked and for students to defend their ideas. For instance, after one group of students presented their prototype that has silvered surfaces, a student asked a question: “what is the function of the silver panels?”, to which his peers replied : “Makes the light bounce. Bounce the sunlight away and then to other parts of the tray.” This question indicated that students applied their knowledge that shiny silvered surfaces reflect light, and they used this knowledge to disperse the light to other trays where the crops were growing. An example of a practical question asked was “what is the purpose of the ladder?”, to which the students replied: “To take the plants – to refill the plants, the workers must climb up”. While the process of presentation and peer critique mimic peer review in the science inquiry process, the conceptual knowledge of science may not always be evident as students paid more attention to the design constraints such as lighting, watering, and space that was set in the activity. Given the context of growing plants, engagement with the science behind nutritional requirements of plants, the process of photosynthesis, and the adaptations of plants could be more deliberately explored.
The goal of our work lies in applying the theoretical ideas of Dewey and Bereiter to better understand reasoning practices in integrate STEM problem solving. We argue that this is a worthy pursue to better understand the roles of scientific reasoning in practical problem solving. One of the goals of integrated STEM education in schools is to enculture students into the practices of science, engineering and mathematics that include disciplinary conceptual knowledge, epistemic practices, and social norms (Kelly & Licona, 2018 ). In the integrated form, the boundaries and approaches to STEM learning are more diverse compared with monodisciplinary ways of problem solving. For instance, in integrated STEM problem solving, besides scientific investigations and explanations, students are also required to understand constraints, design optimal solutions within specific parameters and even to construct prototypes. For students to learn the ways of speaking, doing and being as they participate in integrated STEM problem solving in schools in a meaningful manner, students could benefit from these experiences.
With reference to the first research question of What is the extent of practical and scientific reasoning in integrated STEM problem solving, our analysis suggests that there are fewer instances of scientific reasoning compared with practical reasoning. Considering the intention of integrated STEM learning and adopting Bereiter’s idea that students should learn higher-order conceptual knowledge through engagement with problem solving, we argue for a need for scientific reasoning to be featured more strongly in integrated STEM lessons so that students can gain higher order scientific conceptual knowledge. While the lessons observed were strong in design and building, what was missing in generating solutions was the engagement in investigations, where learners collected or are presented with data and make decisions about the data to allow them to assess how viable the solutions are. Integrated STEM problems can be designed so that science inquiry can be infused, such as carrying out investigations to figure out relationships between variables. Duschl and Bybee ( 2014 ) have argued for the need to engage students in problematising science inquiry and making choices about what works and what does not.
With reference to the second research question , What is achieved through practical and scientific reasoning during integrated STEM problem solving? , our analyses suggest that utterance for practical reasoning are typically used to justify the physical design of the prototype. These utterances rely largely on what is observable and are associated with basic-level knowledge and experiences. The higher frequency of utterances related to practical reasoning and the nature of the utterances suggests that engagement with practical reasoning is more accessible since they relate more to students’ lived experiences and common-sense. Bereiter ( 1992 ) has urged educators to engage learners in learning that is beyond basic-level knowledge since accumulation of basic-level knowledge does not lead to higher-level conceptual learning. Students should be encouraged to use scientific knowledge also to justify their prototype design and to apply scientific evidence and logic to support their ideas. Engagement with scientific reasoning is preferred as conceptual knowledge, epistemic practices and social norms of science are more widely recognised compared with practical reasoning that are likely to be more varied since they rely on personal experiences and common-sense. This leads us to assert that both context and content are important in integrated STEM learning. Understanding the context or the solution without understanding the scientific principles that makes it work makes the learning less meaningful since we “…cannot strip learning of its context, nor study it in a ‘neutral’ context. It is always situated, always relayed to some ongoing enterprise”. (Bruner, 2004 , p. 20).
To further this discussion on how integrated STEM learning experiences harness the ideas of practical and scientific reasoning to move learners from basic-level knowledge to higher-order conceptual knowledge, we propose the need for further studies that involve working with teachers to identify and create relevant problems-of-explanations that focuses on feasible, worthy inquiry ideas such as those related to specific aspects of transportation, alternative energy sources and clean water that have impact on the local community. The design of these problems can incorporate opportunities for systematic scientific investigations and scaffolded such that there are opportunities to engage in epistemic practices of the constitute disciplines of STEM. Researchers could then examine the impact of problems-of-explanations on students’ learning of higher order scientific concepts. During the problem-solving process, more attention can be given to elicit students’ initial and unfolding ideas (practical) and use them as a basis to start the science inquiry process. Researchers can examine how to encourage discussions that focus on making meaning of scientific phenomena that are embedded within specific problems. This will help students to appreciate how data can be used as evidence to support scientific explanations as well as justifications for the solutions to problems. With evidence, learners can be guided to work on reasoning the phenomena with explanatory models. These aspects should move engagement in integrated STEM problem solving from being purely practice to one that is explanatory.
Limitations
There are four key limitations of our study. Firstly, the degree of generalisation of our observations is limited. This study sets out to illustrate what how Dewey and Bereiter’s ideas can be used as lens to examine knowledge used in problem-solving. As such, the findings that we report here is limited in its ability to generalise across different contexts and problems. Secondly, the lessons that were analysed came from teacher-frontal teaching and group presentation of solution and excluded students’ group discussions. We acknowledge that there could potentially be talk that could involve practical and scientific reasonings within group work. There are two practical consideration for choosing to analyse the first and presentation segments of the suite of lesson. Firstly, these two lessons involved participation from everyone in class and we wanted to survey the use of practical and scientific reasoning by the students as a class. Secondly, methodologically, clarity of utterances is important for accurate analysis and as students were wearing face masks during the data collection, their utterances during group discussions lack the clarity for accurate transcription and analysis. Thirdly, insights from this study were gleaned from a small sample of six classes of students. Further work could involve more classes of students although that could require more resources devoted to analysis of the videos. Finally, the number of students varied across groups and this could potentially affect the reasoning practices during discussions.
Acknowledgements
The authors would like to acknowledge the contributions of the other members of the research team who gave their comment and feedback in the conceptualization stage.
Authors’ Contribution
The first author conceptualized, researched, read, analysed and wrote the article.
The second author worked on compiling the essential features and the variations tables.
The third and fourth authors worked with the first author on the ideas and refinements of the idea.
This study is funded by Office of Education Research grant OER 24/19 TAL.
Data Availability
Declarations.
The authors declare that they have no competing interests.
Publisher's Note
Springer Nature remains neutral with regard to jurisdictional claims in published maps and institutional affiliations.
Contributor Information
Aik-Ling Tan, Email: [email protected] .
Yann Shiou Ong, Email: [email protected] .
Yong Sim Ng, Email: [email protected] .
Jared Hong Jie Tan, Email: moc.liamg@derajeijgnohnat .
- Aikenhead GS. Science education for everyday life: Evidence-based practice. Teachers College Press; 2006. [ Google Scholar ]
- Bereiter C. Referent-centred and problem-centred knowledge: Elements of an educational epistemology. Interchange. 1992; 23 (4):337–361. doi: 10.1007/BF01447280. [ CrossRef ] [ Google Scholar ]
- Breiner JM, Johnson CC, Harkness SS, Koehler CM. What is STEM? A discussion about conceptions of STEM in education and partnership. School Science and Mathematics. 2012; 112 (1):3–11. doi: 10.1111/j.194908594.2011.00109.x. [ CrossRef ] [ Google Scholar ]
- Brown, M. J. (2012). John Dewey’s logic of science. HOPS: The Journal of the International Society for the History of Philosophy of Science, 2 (2), 258–306.
- Bruner, J. (2004). The psychology of learning: A short history (pp.13–20). Winter: Daedalus.
- Bryan LA, Moore TJ, Johnson CC, Roehrig GH. Integrated STEM education. In: Johnson CC, Peters-Burton EE, Moore TJ, editors. STEM road map: A framework for integrated STEM education. Routledge; 2016. pp. 23–37. [ Google Scholar ]
- Buchanan R. Wicked problems in design thinking. Design Issues. 1992; 8 (2):5–21. doi: 10.2307/1511637. [ CrossRef ] [ Google Scholar ]
- Bybee RW. The case for STEM education: Challenges and opportunities. NSTA Press; 2013. [ Google Scholar ]
- Crismond DP, Adams RS. The informed design teaching and learning matrix. Journal of Engineering Education. 2012; 101 (4):738–797. doi: 10.1002/j.2168-9830.2012.tb01127.x. [ CrossRef ] [ Google Scholar ]
- Cunningham, C. M., & Lachapelle, P. (2016). Experiences to engage all students. Educational Designer , 3(9), 1–26. https://www.educationaldesigner.org/ed/volume3/issue9/article31/
- Curriculum Planning and Development Division [CPDD] (2021). 2021 Lower secondary science express/ normal (academic) teaching and learning syllabus . Singapore: Ministry of Education.
- Delahunty T, Seery N, Lynch R. Exploring problem conceptualization and performance in STEM problem solving contexts. Instructional Science. 2020; 48 :395–425. doi: 10.1007/s11251-020-09515-4. [ CrossRef ] [ Google Scholar ]
- Dewey J. Logic: The theory of inquiry. Henry Holt and Company Inc; 1938. [ Google Scholar ]
- Dewey J. Science as subject-matter and as method. Science. 1910; 31 (787):121–127. doi: 10.1126/science.31.787.121. [ PubMed ] [ CrossRef ] [ Google Scholar ]
- Dewey J. How we think. D.C. Heath & Co Publishers; 1910. [ Google Scholar ]
- Duschl, R. A., & Bybee, R. W. (2014). Planning and carrying out investigations: an entry to learning and to teacher professional development around NGSS science and engineering practices. International Journal of STEM Education, 1 (12). DOI: 10.1186/s40594-014-0012-6.
- Gale, J., Alemder, M., Lingle, J., & Newton, S (2000). Exploring critical components of an integrated STEM curriculum: An application of the innovation implementation framework. International Journal of STEM Education, 7(5), 10.1186/s40594-020-0204-1.
- Hsieh H-F, Shannon SE. Three approaches to qualitative content analysis. Qualitative Health Research. 2005; 15 (9):1277–1288. doi: 10.1177/1049732305276687. [ PubMed ] [ CrossRef ] [ Google Scholar ]
- Jonassen DH. Toward a design theory of problem solving. ETR&D. 2000; 48 (4):63–85. doi: 10.1007/BF02300500. [ CrossRef ] [ Google Scholar ]
- Kelly, G., & Licona, P. (2018). Epistemic practices and science education. In M. R. Matthews (Ed.), History, philosophy and science teaching: New perspectives (pp. 139–165). Cham, Switzerland: Springer. 10.1007/978-3-319-62616-1.
- Lee O, Luykx A. Science education and student diversity: Synthesis and research agenda. Cambridge University Press; 2006. [ Google Scholar ]
- Li D. The pragmatic construction of word meaning in utterances. Journal of Chinese Language and Computing. 2008; 18 (3):121–137. [ Google Scholar ]
- National Research Council . The National Science Education standards. National Academy Press; 1996. [ Google Scholar ]
- National Research Council (2000). Inquiry and the national science education standards: A guide for teaching and learning. Washington, DC: The National Academies Press. 10.17226/9596.
- OECD (2018). The future of education and skills: Education 2030. Downloaded on October 3, 2020 from https://www.oecd.org/education/2030/E2030%20Position%20Paper%20(05.04.2018).pdf
- Park, W., Wu, J.-Y., & Erduran, S. (2020) The nature of STEM disciplines in science education standards documents from the USA, Korea and Taiwan: Focusing on disciplinary aims, values and practices. Science & Education, 29 , 899–927.
- Pleasants J. Inquiring into the nature of STEM problems: Implications for pre-college education. Science & Education. 2020; 29 :831–855. doi: 10.1007/s11191-020-00135-5. [ CrossRef ] [ Google Scholar ]
- Roehrig, G. H., Dare, E. A., Ring-Whalen, E., & Wieselmann, J. R. (2021). Understanding coherence and integration in integrated STEM curriculum. International Journal of STEM Education, 8(2), 10.1186/s40594-020-00259-8
- SFA (2020). The food we eat . Downloaded on May 5, 2021 from https://www.sfa.gov.sg/food-farming/singapore-food-supply/the-food-we-eat
- Svandova K. Secondary school students’ misconceptions about photosynthesis and plant respiration: Preliminary results. Eurasia Journal of Mathematics, Science, & Technology Education. 2014; 10 (1):59–67. doi: 10.12973/eurasia.2014.1018a. [ CrossRef ] [ Google Scholar ]
- Tan M. Context matters in science education. Cultural Studies of Science Education. 2020 doi: 10.1007/s11422-020-09971-x. [ CrossRef ] [ Google Scholar ]
- Tan, A.-L., Teo, T. W., Choy, B. H., & Ong, Y. S. (2019). The S-T-E-M Quartet. Innovation and Education , 1 (1), 3. 10.1186/s42862-019-0005-x
- Wheeler LB, Navy SL, Maeng JL, Whitworth BA. Development and validation of the Classroom Observation Protocol for Engineering Design (COPED) Journal of Research in Science Teaching. 2019; 56 (9):1285–1305. doi: 10.1002/tea.21557. [ CrossRef ] [ Google Scholar ]
- World Economic Forum (2020). Schools of the future: Defining new models of education for the fourth industrial revolution. Retrieved on Jan 18, 2020 from https://www.weforum.org/reports/schools-of-the-future-defining-new-models-of-education-for-the-fourth-industrial-revolution/
- Math for Kids
- Parenting Resources
- ELA for Kids
- Teaching Resources
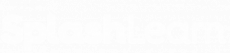
10 Best Math Intervention Strategies for Struggling Students
How to Teach Division to Kids in 11 Easy Steps
How to Teach Place Value in 9 Easy Steps
8 Math Division Tricks: Making Division Fun & Accessible
10 Best Strategies for Solving Math Word Problems
When Do Kids Start Preschool: Age & Readiness Skills
Kindergarten Readiness Checklist: A Guide for Parents
How to Choose Best School For Your Kid: 12 Best Tips
Why Kids Get Bored at School: 10 Tips to Keep Them Interested
11 Best Writing Apps for Kids
6 Effective Ways to Improve Writing Skills
40 Four Letter Words That Start With A
What Are the Stages of Spelling Development: Ultimate Guide
48 Rhyming Words for Kindergarten Kids
How to Teach Vowels to Kids: A Step-by-Step Guide
What is Teachers Professional Development: Strategies & More
11 Best Ways to Create a Positive Learning Environment for Kids
How to Encourage Creativity in the Classroom – 9 Best Tips
25 Best Websites for Teachers
10 Best Lesson Planning Apps for Teachers
What Is “Inquiry-Based Learning”?: Types, Benefits, Examples
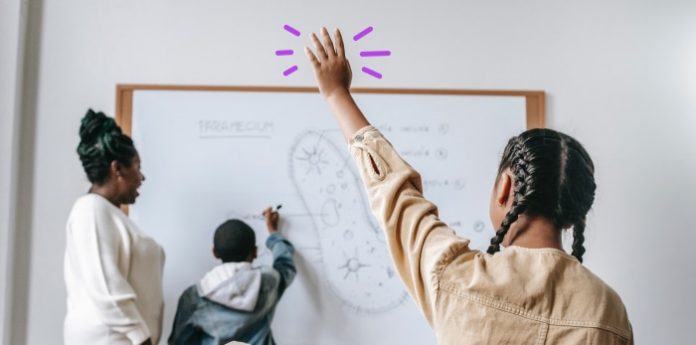
What Is Inquiry-Based Learning?
The 4 types of inquiry-based learning, 7 benefits of inquiry-based learning, 5 inquiry-based learning examples, 5 strategies and tips for implementing inquiry-based learning, 4 models to use in the classroom, let’s wrap, frequently asked questions (faqs).
Are you looking for a teaching strategy that will engage your students in the learning process? Do you want them to be able to ask questions and investigate real-world problems? If so, you should consider using inquiry-based learning in your classroom.
Inquiry-based learning is a teaching method that encourages students to ask questions and investigate real-world problems. This type of learning has many benefits and can be used in various subject areas.
This blog will discuss the benefits of inquiry-based learning and provide some strategies, tips, and models that you can use in your classroom. But first, let’s take a closer look at what inquiry-based learning is.
- What is inquiry-based learning
- Types of inquiry-based learning
- Benefits of inquiry-based learning
- Inquiry-based learning examples
- Strategies for implementing inquiry-based learning in the classroom
- Four models to use in the classroom

Inquiry-based learning is a student-centered teaching method that encourages students to ask questions and investigate real-world problems. In this type of learning environment, students are actively engaged in the learning process and are given the opportunity to explore their natural curiosities.
This type of learning is often hands-on and allows students to connect what they learn in the classroom and the real world. Inquiry-based learning has been shown to improve critical thinking skills, problem-solving skills, and creativity.
SplashLearn: Most Comprehensive Learning Program for PreK-5

SplashLearn inspires lifelong curiosity with its game-based PreK-5 learning program loved by over 40 million children. With over 4,000 fun games and activities, it’s the perfect balance of learning and play for your little one.
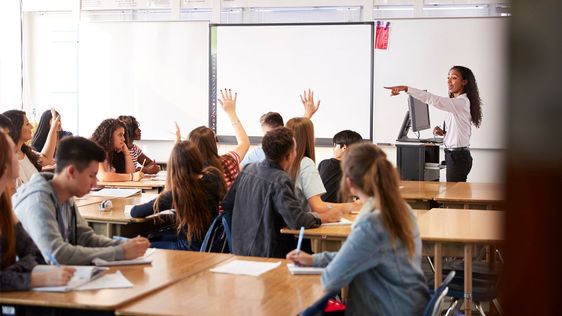
There are four types of inquiry-based learning:
1. The Structured Inquiry Approach
The structured inquiry approach is a sequential process that helps students learn how to ask questions and investigate real-world problems. This type of inquiry-based learning is often used in science classes, where students are given a problem to investigate and are taught how to use the scientific process to find a solution.
2. The Open-Ended Inquiry Approach
The open-ended inquiry approach is a more free-form approach to inquiry-based learning. In this type of learning environment, students are given the freedom to explore their interests and ask questions about the topic they are studying. This type of inquiry-based learning is often used in humanities classes, where students are asked to explore a topic in-depth and debate different viewpoints.
3. The Problem-Based Inquiry Approach
A problem-based inquiry approach is a problem-solving approach to inquiry-based learning. In this type of approach, students are given a real-world problem to solve. This type of inquiry-based learning is often used in mathematics and engineering classes, where students are asked to apply what they have learned to solve a real-world problem.
4. The Guided Inquiry Approach
The guided inquiry approach is a teacher-led approach to inquiry-based learning. In this type of approach, the teacher guides the students through the inquiry process and helps them to ask questions and find solutions to real-world problems. This type of inquiry-based learning is often used in elementary and middle school classrooms.
Now that we have a better understanding of the different types of inquiry-based learning, let’s take a look at the benefits.

With so many benefits, it is no wonder that inquiry-based learning has become a popular teaching method . Some of the benefits of inquiry-based learning include:
1. Encourages critical thinking
Inquiry-based learning encourages students to think critically about the information they are presented with. They are asked to question the information and develop their own solutions. This type of learning helps students develop problem-solving skills and critical-thinking skills.
2. Improves problem-solving skills
Inquiry-based learning helps students develop problem-solving skills. When they are given the opportunity to explore real-world problems, they are forced to think outside the box and come up with their own solutions. This is an important skill that will help them in their future careers.
3. Encourages creativity
This concept of learning encourages creativity. When students are given the opportunity to explore a problem independently, they often come up with creative solutions. This is due to the fact that any particular way of thinking does not restrict them.
4. Improves communication skills
It also helps students improve their communication skills. When working on a problem, they often have to explain their thoughts and ideas to others. This helps them learn how to communicate effectively with others.
5. Connects learning to the real world
Inquiry-based learning helps connect learning to the real world. When students are allowed to explore problems that exist in the real world, they can see how what they are learning in the classroom is relevant. This also helps them develop a better understanding of the material.
6. Helps students understand complex topics
Inquiry-based learning can also help students understand complex topics. When they are allowed to explore these topics in a hands-on environment, they can learn about them more meaningfully.
7. Encourages engaged learning
Finally, this type of learning encourages engaged learning. When students are actively involved in the learning process, they are more likely to retain the information. This is due to the fact that they are invested in what they are doing.

Now that we have looked at the benefits of inquiry-based learning, let’s take a look at some examples.
1. Science Experiments
One way to incorporate inquiry-based learning into your classroom is to allow students to conduct experiments. This will encourage them to ask questions and think critically about the results.
2. Field Trips
Another way to encourage inquiry-based learning is to take students on field trips. This will allow them to explore real-world problems and see how what they are learning in the classroom is relevant.
3. Classroom Debates
Classroom debates are another great way to encourage this type of learning. When students debate a topic, they are forced to think critically about both sides of the argument.
4. Projects
Projects are another great way to encourage inquiry-based learning. When students are given the opportunity to work on a project that is related to the topic they are studying, they will be more likely to learn and remember the information.
5. Group Work
When students work in groups, they are able to share their ideas and thoughts with others. This helps them to understand the material better.

Now that we have looked at the benefits of inquiry-based learning and some examples, let’s look at some inquiry-based strategies and tips that you can use in your classroom.
1. Start with a Question
The best way to start an inquiry-based lesson is by asking a question. This will get students thinking about the topic and will encourage them to ask their own questions.
2. Allow for Exploration
Once you have asked a question, allow students to explore the topic on their own. This will help them to understand the material better.
3. Encourage Discussion
Encourage students to discuss their ideas with each other. This will help them to develop a better understanding of the material.
4. Provide Resources
Be sure to provide students with resources that they can use to explore the topic. This will help them develop a better understanding. Teachers can also give access to online learning platforms like SplashLearn , which further help enhance the knowledge of the concepts.
5. Summarize What Was Learned
At the end of the lesson, be sure to summarize what was learned. This will help students to remember the information.
You can use different models to encourage inquiry-based learning in your classroom. The important thing is that you allow students to be actively involved in the learning process. Let’s have a look at a few models that you can use.
Now that we have looked at the benefits of inquiry-based learning and some strategies for implementing it in your classroom , let’s take a look at four models you can use.
1. The Question Model
The question model is one of the most basic models for inquiry-based learning. It involves asking students questions about the topic you are teaching. This will encourage them to think critically about the material.
2. The Problem-Based Learning Model
The problem-based learning model is another excellent option for inquiry-based learning. This model involves giving students a problem to solve. They will need to think critically about the problem and find a solution.
3. The Project-Based Learning Model
Project-based learning is a great way for students to explore a topic in depth. This model involves giving students a project to work on that is related to the topic you are teaching.
4. The Inquiry Cycle Model
With the inquiry cycle model, students are given the opportunity to ask questions, investigate a topic, and then share their findings. This model allows students to explore a topic in-depth and share their discoveries with others.
Inquiry-based learning is a teaching method that encourages students to ask questions and explore their answers. This type of learning has many benefits, both for students and teachers. In this article, we’ve looked at some of the critical benefits of inquiry-based learning as well as strategies you can use to get started in your own classroom. We hope you’re inspired to give it a try!
What is the importance of inquiry-based learning?
Inquiry-based learning is important because it allows students to explore and ask questions about the world around them. This type of learning helps students develop critical thinking and problem-solving skills.
What is the definition of inquiry-based learning?
Inquiry-based learning is a type of active learning that encourages students to ask questions, conduct research, and explore new ideas. This approach to learning helps students develop critical thinking, problem-solving, and research skills.
What are the roles of students in inquiry-based learning?
In inquiry-based learning, students take on the role of researcher. They are encouraged to ask questions and explore new ideas. Students also have the opportunity to share their findings with their classmates and learn from each other.
How do you plan an inquiry-based lesson?
Inquiry-based lessons are typically designed around a central question or problem. From there, teachers can provide resources and scaffolding to help students investigate the topic. It is important to leave room for student exploration and allow them to ask their own questions.
What are the five guiding questions of inquiry?
The 5 guiding questions of inquiry are:

Do inquiry-based and project-based learning have to be the same thing?
No, inquiry-based and project-based learning are two different approaches. Inquiry-based learning is focused on student-driven research and exploration. Project-based learning is focused on students working together to complete a real-world project. However, both approaches can include elements of inquiry and problem-solving.
12 Study Habits of Students for Optimal Academic Performance
What is Cooperative Learning: Benefits, How to Use & More
10 Best Techniques to Use Distributed Practice at Your School
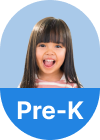
Most Popular
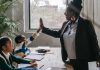
15 Best Report Card Comments Samples
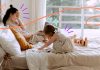
101 Best Riddles for Kids (With Explanation)

40 Best Good Vibes Quotes to Brighten Your Day
Recent posts.
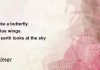
11 Best Easter Poems for Kids in 2024
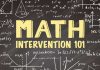
15 Best Reading Fluency Activities for Early Learners
Math & ela | prek to grade 5, kids see fun., you see real learning outcomes..
Watch your kids fall in love with math & reading through our scientifically designed curriculum.
Parents, try for free Teachers, use for free
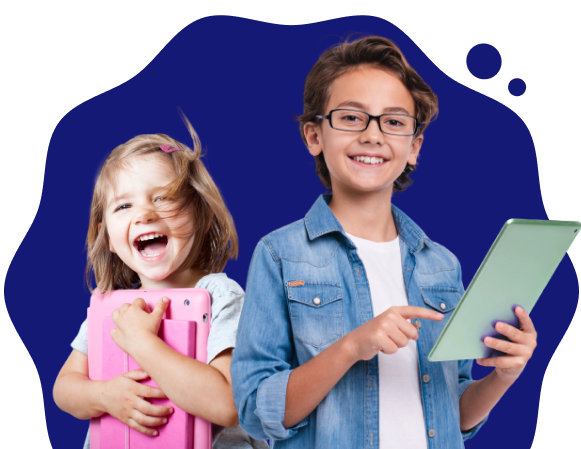
About SplashLearn
Enter the Splashverse! Inspire lifelong curiosity with this game-based PreK-5 learning experience loved by over 40 million children. SplashLearn is the perfect balance of learning and game-play that your little one needs to build math and reading confidence.
- Games for Kids
- Worksheets for Kids
- Math Worksheets
- ELA Worksheets
- Math Vocabulary
- Number Games
- Addition Games
- Subtraction Games
- Multiplication Games
- Division Games
- Addition Worksheets
- Subtraction Worksheets
- Multiplication Worksheets
- Division Worksheets
- Times Tables Worksheets
- Reading Games
- Writing Games
- Phonics Games
- Sight Words Games
- Letter Tracing Games
- Reading Worksheets
- Writing Worksheets
- Phonics Worksheets
- Sight Words Worksheets
- Letter Tracing Worksheets
- Prime Number
- Order of Operations
- Long multiplication
- Place value
- Parallelogram
- SplashLearn Success Stories
- SplashLearn Apps
- [email protected]
© Copyright - SplashLearn
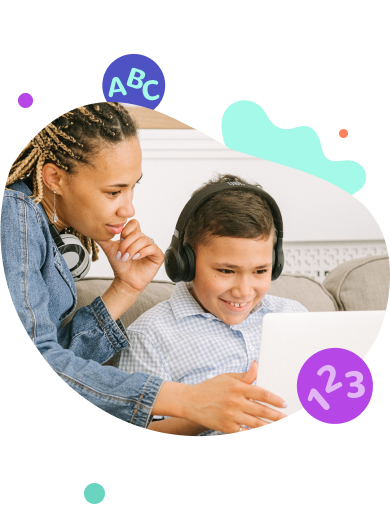
Make study-time fun with 14,000+ games & activities, 450+ lesson plans, and more—free forever.
Parents, Try for Free Teachers, Use for Free

- MISSION / VISION
- DIVERSITY STATEMENT
- CAREER OPPORTUNITIES
- Kide Science
- STEMscopes Science
- Collaborate Science
- STEMscopes Math
- Math Nation
- STEMscopes Coding
- Mastery Coding
- DIVE-in Engineering
- STEMscopes Streaming
- Tuva Data Literacy
- NATIONAL INSTITUTE FOR STEM EDUCATION
- STEMSCOPES PROFESSIONAL LEARNING
- RESEARCH & EFFICACY STUDIES
- STEM EDUCATION WEBINARS
- LEARNING EQUITY
- DISTANCE LEARNING
- PRODUCT UPDATES
- LMS INTEGRATIONS
- STEMSCOPES BLOG
- FREE RESOURCES
- TESTIMONIALS
What is Inquiry-based Learning?
ALI Staff | Published August 31, 2023 | Updated December 14, 2023
Sparking student engagement, no matter the subject matter, often means meeting students where they’re already at.
It’s about tapping into their natural curiosity while motivating them to do the work necessary to learn the lesson.
This can be accomplished through projects, group work, games, and inquiry-based instruction.
Each of these strategies shares the idea of hands-on learning in some way, but inquiry education takes a unique approach, giving students an increased level of autonomy to help drive engagement.
What is inquiry in education?
Whether it receives a label or not, a significant goal of education is to drive students to ask questions and seek the truth to answer them.
Students need to feel confident and motivated to want to know more. Creating an environment in your own classroom where student inquiry is comfortable isn’t always easy.
Time constraints may make it hard to address all questions or spend time teaching students how to find answers themselves.
One way to meet this need without burdening your curriculum is to incorporate inquiry-based learning.
What is inquiry-based learning?
Inquiry education is a student-centered approach to learning that encourages students to search for truth as it relates to real-world problems.
They’re given autonomy to ask their own questions and/or develop their own methods for finding answers.
This strategy actively engages students in the learning process while allowing them to explore their natural curiosities. The use of real-world issues also ensures they’re making relevant connections to the world around them.
4 Ways to Use Inquiry in Education
Because inquiry learning has such a high-level definition, how you decide to use it in your classroom can vary. Thankfully, there are four different approaches that make it easier to incorporate inquiry-based learning into your curriculum.
1. Structured/Confirmation inquiry
This sequential approach to questioning and researching makes it ideal for science classes. Any questions that require a process or specific methodology to get to the solution will benefit from this inquiry-based learning method.
2. Open-ended Inquiry
For subjects that can allow students to think in a more creative way to answer questions, the open-ended approach works best. This is helpful in reading, history, the arts — all humanities classes — where students may use a variety of sources and strategies to gather information, formulate answers, and possibly find more questions to ask.
3. Problem-based inquiry
Ideal for problems that address real-world issues in areas like math and engineering, this approach to inquiry-based learning makes it easy for students to use what they’ve already learned to find answers to questions that require strong problem-solving skills.
4. Guided Inquiry
For younger students, who may need more time to be ready to manage inquiry-based learning, this approach is teacher-led. While student inquiry is still occurring in this approach, the teacher helps them ask questions and find solutions, primarily to a real-world problem they can identify with.
Benefits of inquiry-based learning
The biggest benefit of adopting inquiry in the classroom is the power it gives students to direct their own learning.
It triggers curiosity because it allows students to look at a topic or question from their own perspective.
It also provides them with autonomy (to some degree) to search for an answer. When it’s so easy for students to feel like they have no control over what they’re learning, this is quite a bonus.
Other benefits of inquiry-based learning include:
- Improved critical thinking skills
- Enhanced problem-solving skills
- Better communication skills
- More opportunities to think creatively
- Practice presenting thoughts and ideas to others in an accessible way
- Stronger connections to the real world and how what they’re learning is relevant
Inquiry-based learning allows students to find their own solutions, even if it means thinking outside the box.
They get to decide where to go for answers, how they'll explore solving a problem, and in what way they’ll share their findings.
It’s a little more freedom than they might find otherwise, yet it’s all within the confines of learning the material.
Where and how to implement inquiry learning
Inquiry-based learning is always hands-on in some way.
Whether that’s through a specific activity, with steps to accomplish, or simply observing the world around them to draw conclusions, there are many ways to implement inquiry in the classroom, including:
- Field trips
- Classroom debate
Regardless of the activity that’s framing your inquiry-based instruction, to get those inquiring minds active, start with a question.
This is either something big-picture that comes from you or something students develop on their own.
No matter where the question comes from, it’s then up to the students to find an answer.
As they do this, they’ll naturally access a variety of resources. Make sure to provide a few starting sources for help, and encourage students to bounce ideas off each other if they’re able to collaborate.
By presenting what they’ve learned and then reflecting on their work, students are actively participating in the learning process.
This not only helps them achieve the goal of the assignment but makes it easier to retain this new information to use again.
The lasting effect of inquiry in education
Asking questions about and finding answers to real-world issues are two abilities students need to develop as they learn.
Often, the focus of skill development is on problem-solving, communication, GRIT, and so many other essential soft skills, but inquiry-based learning covers them all.
It allows students to flex these all-important qualities while learning to ask good questions and seek solid answers.
This learning strategy completes the package of abilities students should take with them out into the real world, becoming stronger professionals no matter their career choice.
Share this post!
Related articles.
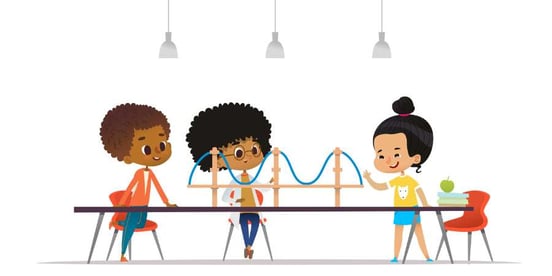
Engineering in Elementary School
Integrating engineering concepts into elementary education is a crucial topic in this day and age.
It's about...
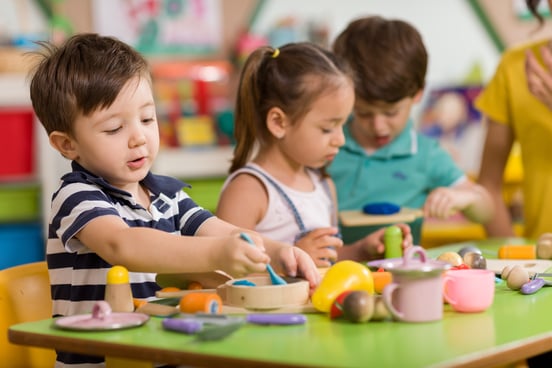
Top 7 Benefits of Play-Based Learning In Early Childhood Education
The idea of a play-based curriculum may sound counterintuitive.
“Play” suggests giving children free rein to explore...
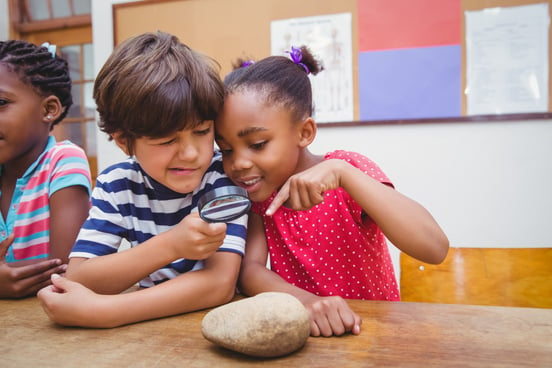
Exploring the Engineering Design Process for Kids
Introducing the Engineering Design Process for kids can sound like an intimidating concept.
As with any other framework...
STAY INFORMED ON THE LATEST IN STEM. SUBSCRIBE TODAY!
Which stem subjects are of interest to you.
STEMscopes Tech Specifications STEMscopes Security Information & Compliance Privacy Policy Terms and Conditions
© 2024 Accelerate Learning
- Prodigy Math
- Prodigy English
From our blog
- Is a Premium Membership Worth It?
- Promote a Growth Mindset
- Help Your Child Who's Struggling with Math
- Parent's Guide to Prodigy
- Assessments
- Math Curriculum Coverage
- English Curriculum Coverage
- Game Portal
What Is Inquiry-Based Learning: 7 Benefits & Strategies You Need to Know
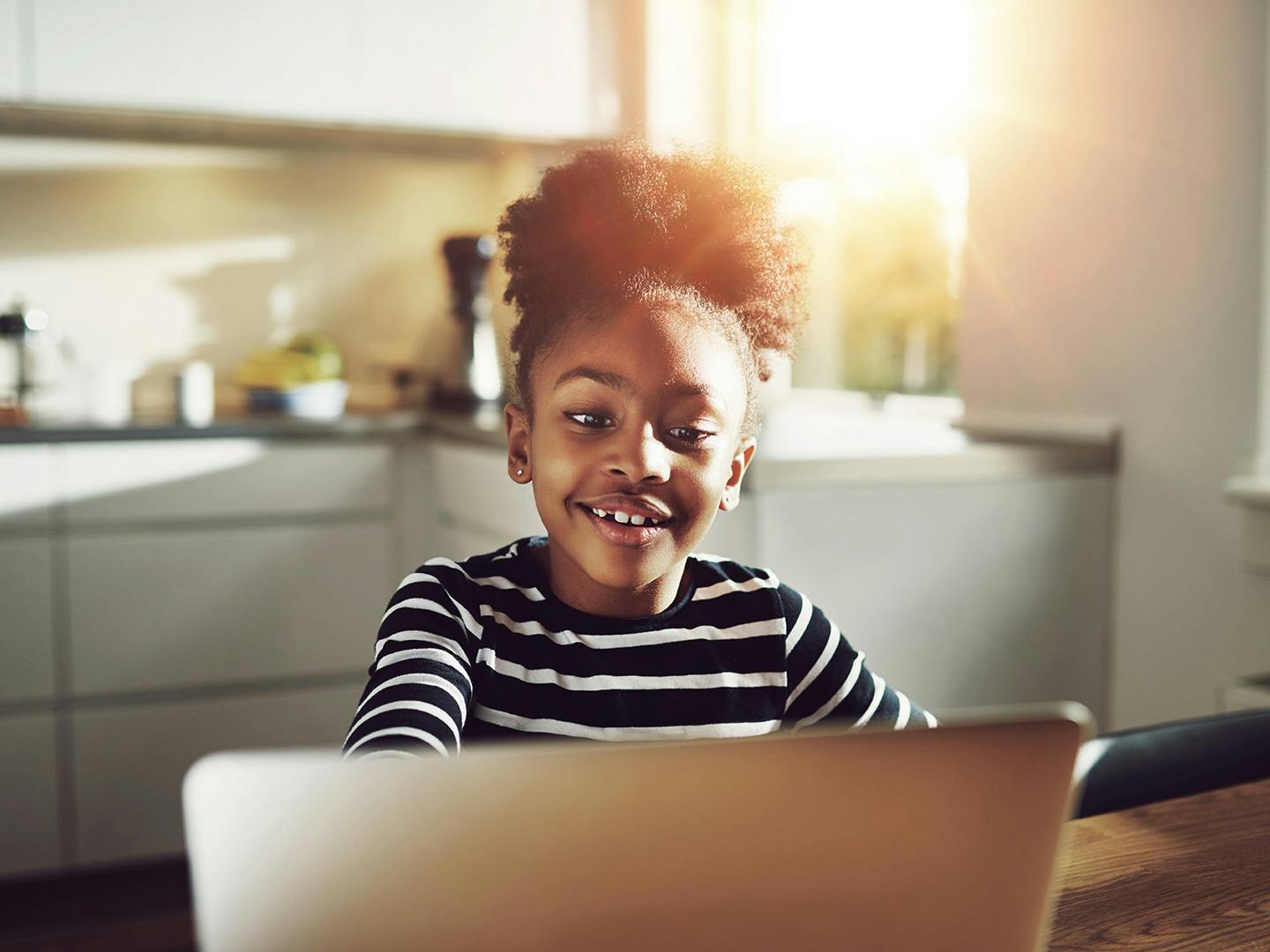
Written by Marcus Guido
Help inspire your students to love learning with Prodigy's online learning platform.
- Teaching Strategies
What Is Inquiry-Based Learning?
- Benefits of inquiry-based learning
- Strategies for implementing activities
Developed in the 1960s, many teachers see inquiry-based learning as a new pedagogy -- meaning they have questions about how to use it and if it’s worthwhile.
Like problem-based learning , proponents state that letting students investigate solutions to open questions has a range of advantages. But the pedagogy must be shaped by research-backed approaches to reap these advantages.
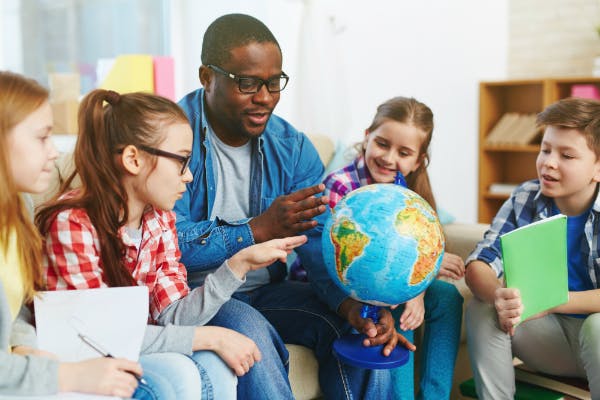
If you’re familiar with the definition of inquiry-based learning, feel free to skip this section .
For the many educators who aren’t, it is a learning and teaching method that prioritizes student questions, ideas and analyses. To highlight the pedagogy's nuances, it is important to define inquiry-based learning from both a learner and teacher perspective.
From a student point-of-view, inquiry-based learning focuses on investigating an open question or problem. They must use evidence-based reasoning and creative problem-solving to reach a conclusion, which they must defend or present.
From a teacher point-of-view, inquiry-based teaching focuses on moving students beyond general curiosity into the realms of critical thinking and understanding. You must encourage students to ask questions and support them through the investigation process, understanding when to begin and how to structure an inquiry activity.
Using methods such as guided research, document analysis and question-and-answer sessions, you can run inquiry activities in the form of:
- Case studies
- Group projects
- Research projects
- Field work, especially for science lessons
- Unique exercises tailored to your students
Whichever kind of activity you use, it should allow students to develop unique strategies for solving open questions.
The 4 Types of Inquiry-Based Learning
There are different kinds of inquiry-based learning, which become decreasingly structured and suit different classrooms:
- Confirmation Inquiry -- You give students a question, its answer and the method of reaching this answer. Their goal is to build investigation and critical-thinking skills, learning how the specific method works.
- Structured Inquiry -- You give students an open question and an investigation method. They must use the method to craft an evidence-backed conclusion.
- Guided Inquiry -- You give students an open question. Typically in groups, they design investigation methods to reach a conclusion.
- Open Inquiry -- You give students time and support. They pose original questions that they investigate through their own methods, and eventually present their results to discuss and expand.
Regardless of the type, inquiry-based learning aims to develop students’ abilities to analyze, synthesize and evaluate information -- indications of high-level thinking according to Bloom’s Taxonomy .
7 Benefits of Inquiry-Based Learning
As well as building skills to help students reach a high level of thinking, inquiry-based learning can deliver other benefits to students and teachers.
1. Reinforces Curriculum Content
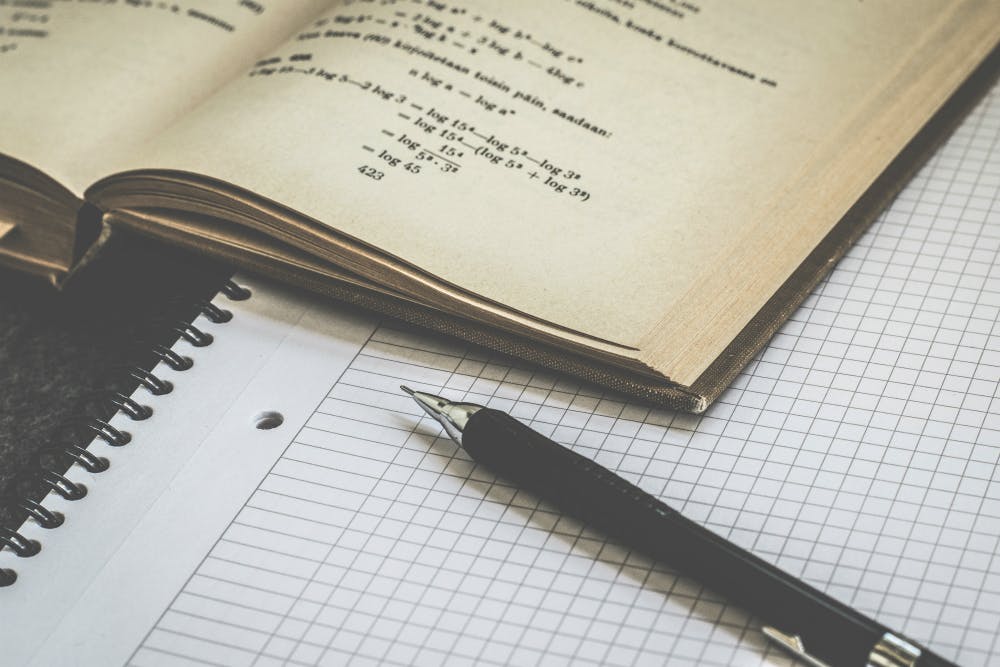
Whereas some see inquiry-based learning as a departure from the curriculum, you can use it to reinforce relevant content and improve understanding of core concepts .
This is due to curiosity’s effect on the brain. When a concept sparks curiosity, there is increased activity in the hippocampus -- the region of the brain responsible for memory creation.
When students show more curiosity than normal regarding a specific topic, satiate it by using their questions to introduce an inquiry activity in the coming days.
In doing so, they should effectively retain essential information gleaned during the exercise, according to a study from the Association for Psychological Science .
2. “Warms Up” the Brain for Learning
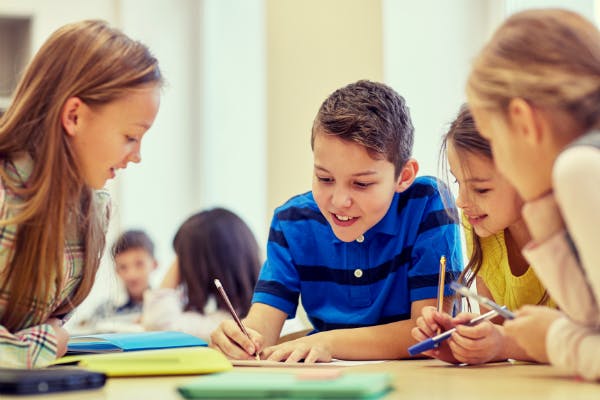
Running a brief inquiry activity to start class can help students absorb information throughout the day, according to the same study.
Specifically, it states that curiosity prepares the brain for learning -- allowing students to become more proficient at understanding and remembering skills and concepts.
An easy way to inspire curiosity is by launching an inquiry activity as a surprise. Related to a recent topic students found especially interesting, begin a lesson by playing a video or sharing a primary source document. Then, give students an open question to answer either individually or as a group.
This will help start class in a curiosity-sparking, intellectually-stimulating way.
3. Promotes a Deeper Understanding of Content
By delving into a concept through inquiry, students should see it as more than a simple rule, idea or formula.
Many of them will understand:
- How the idea was developed
- Why the rule or formula works
- When they can properly apply the rule, idea or formula
This is because the process of asking open questions, solving them through original strategies, empowers students to take ownership of their learning . Barring hiccups, they should be able to build understanding of a concept through their own methods and thinking styles. The same principle applies to experiential learning , which puts students at the center of the learning experience.
They won’t have to follow a process they can’t grasp, possibly arriving at a seemingly-unjustified conclusion.
4. Helps Make Learning Rewarding
Inquiry can help students see the intrinsic rewards of learning, says an oft-cited article from the Harvard Educational Review .
The author states that many kids learn in an attempt to earn “the rewards of parental or teacher approval or the avoidance of failure.” As a result, they may not appreciate the inherent benefits of learning.
He hypothesized that inquiry-based learning instills a different mindset . It shows students how fulfilling the act of discovery is, and that theorizing a new strategy or original conclusion is a reward. Because of this, they grow to enjoy the learning process itself -- not parent or teacher approval. This means that student appreciation for learning can improve with a simple inquiry exercise.
5. Builds Initiative and Self-Direction
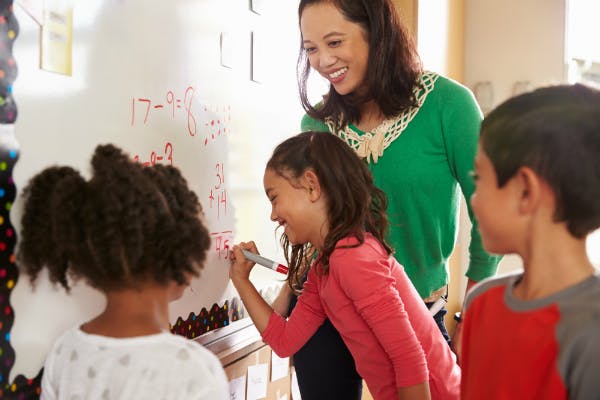
Students can improve certain transferable skills through inquiry-based learning, many of which relate to initiative and self-direction. This is evident when examining the steps of the inquiry process. Students learn how to ask questions, investigate, discuss, collaborate, cooperate and reach their own conclusions. Although they can separately build these skills through other activities, self-guided inquiry and analysis synthesizes this development. Such skills will not only prove useful as students reach higher grades but enter post-secondary school and beyond.
6. Works in Almost Any Classroom
Inquiry-based learning can also benefit teachers, as you can repurpose activities for almost any classroom . Even regardless of grade and individual skill levels. This is because you can:
- Adapt the pace and content to suit the needs of students
- Appeal to students who struggle to grasp content through traditional lessons
- Deliver exercises that greatly differ, using distinct content and investigation methods
- Use an inquiry exercise as either a “minds-on” activity, review, full lesson or standalone project
- Reinforce and expand upon any relevant concept, as long as students have shown curiosity towards it
In these ways, you’ll have the flexibility to provide inquiry exercises to the majority of your classes year after year.
7. Offers Differentiated Instruction
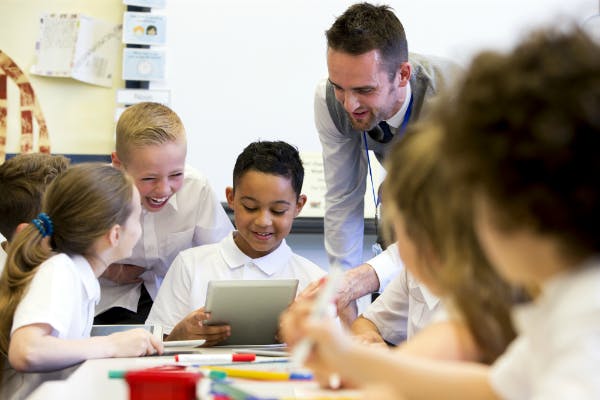
Running an inquiry-based learning activity will give you a chance to use differentiated instruction strategies , appealing to the diverse learning styles of your students. Students can work by themselves, or as part of a small or large group. Inquiry itself typically involves methods such as discussion and guided research. You can also provide content in form of text, audio, video and virtual or physical manipulatives such as building blocks. Delivering a range of content and ways to process it, inquiry activities can allow you to meet your students’ distinct learning needs and preferences. Prodigy Math Game is an adaptive learning platform that helps students practice math skills at their own pace. Sign up for a free teacher account today to help:
- Deliver differentiated assessments and test preps
- Inform your instruction and drive student achievement
- Pinpoint students' working grade levels and their levels on key strands
7 Inquiry-Based Learning Strategies and Activities for Teachers
Like any teaching method, there are strategies to help you successfully run an inquiry activity. These strategies will also allow you and your students to enjoy the full extent of inquiry-based learning’s benefits.
1. Keep Guiding Principles in Mind
To run an inquiry activity, there are broad principles you should follow:
- Learners are at the centre of the inquiry process. You, along with the resources and technology you provide are there to support them.
- Inquiry activities themselves should concentrate on building information-processing and critical thinking abilities.
- You should monitor how students develop these skills as they build conceptual understanding of the topic in question.
- As well as facilitating the exercise, try to learn more about your students’ learning habits and inquiry-based learning in general.
Keeping these principles in mind should keep you and your students focused on the overarching purposes of inquiry-based learning.
2. Demonstrate How to Participate
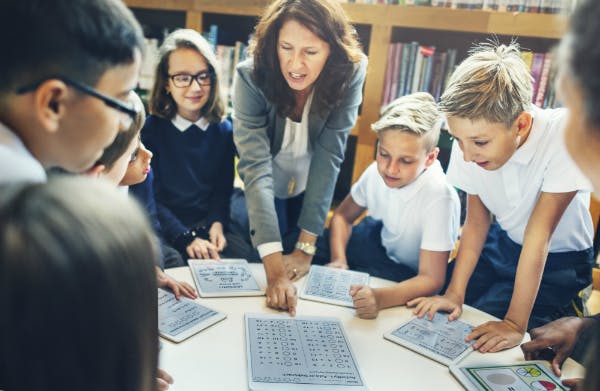
Because students may not be familiar with inquiry-based learning, consider demonstrating how to participate in an inquiry activity. Specifically, they must learn how to:
- Contribute ideas
- Develop those ideas
- Question themselves and group members in a constructive manner
- Investigate, to the fullest extent possible, their ideas and hypotheses
Launching a mock-exercise for the class to tackle as a group, actively participate to give students a first-hand look at how to complete these steps. For example, after presenting an open question, facilitate and contribute to a brainstorming session. This will exemplify pitching and developing ideas. Demonstrating how to participate in this way should prepare students for future exercises.
3. Surprise Students
To spark curiosity and enjoy its aforementioned benefits, run a surprise inquiry activity. With little to no context, start class by:
- Playing a video
- Handing out a mathematical formula or list of math word problems
- Distributing a primary source document
The content piece must relate to a topic that interests students, effectively engaging them. After they’ve examined the content, split them into small groups and give them an open question to answer. For example, you may ask them to determine applications for the mathematical formula or word problems. As research about curiosity indicates, their findings and conclusions should stick with them beyond the activity.
4. Use Inquiry when Traditional Methods Won’t Work
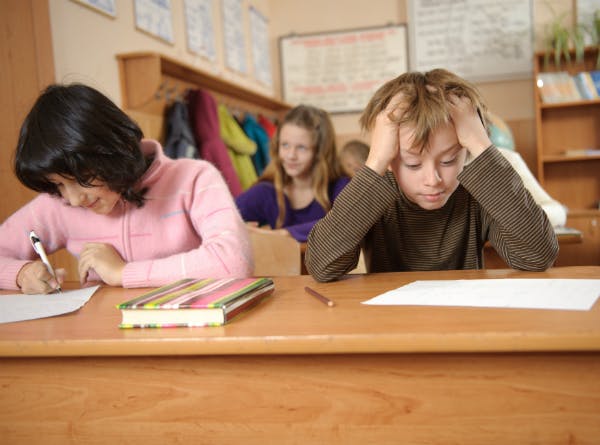
Structured or guided inquiry activities can lend themselves to topics that students typically struggle to grasp, allowing them to process content in different ways. Investigating a question you present, they should be able to use their own techniques to analyze information that may normally be too challenging otherwise. As a result, they’ll likely form conclusions that make sense to them. You can then discuss these conclusions and fill knowledge gaps to ensure everyone is on the same page. Furthermore, monitoring students throughout the activity can teach you about their learning styles, informing how to approach other difficult lessons. If you are writing report card comments , you may use the opportunity to observe student behavior.
5. Understand When Inquiry Won’t Work
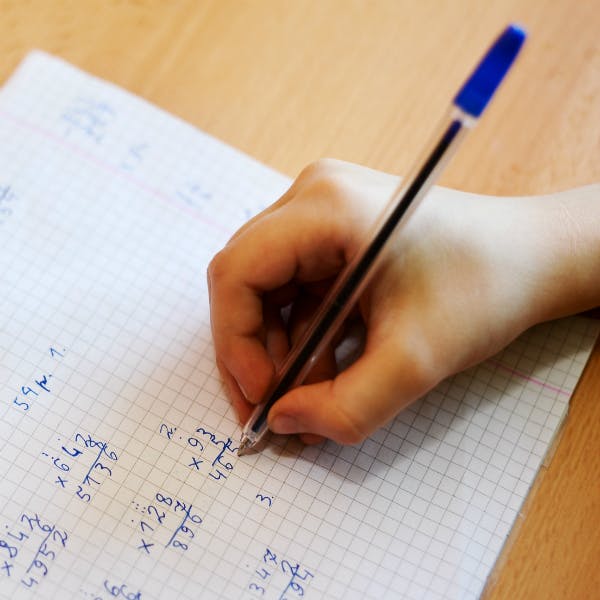
Inquiry-based learning delivers its share of benefits, but you must recognize which lessons don’t call for inquiry. Take this scenario as an example: You want to run a guided inquiry activity for math class, which (a) introduces negative integers and (b) requires students to determine the concept's application in real-life scenarios. Asking students to read an introductory text about negative integers will likely drain time and cause confusion. On the other hand, a brief overview will allow them to spend more time on the latter part of the activity, which focuses on analysis and discovery. As this example shows, there are cases when a simple explanation will suffice over an elongated activity.
6. Don’t Wait for the Perfect Question
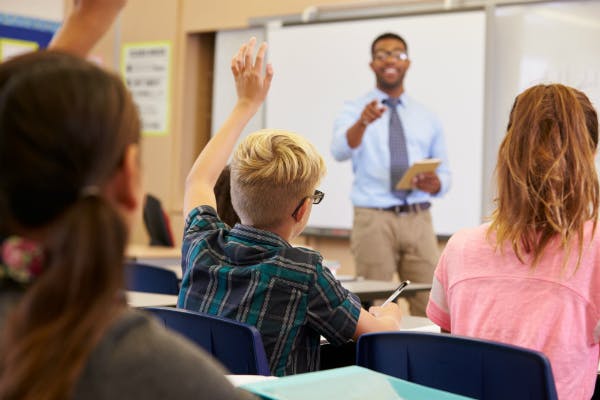
A student can ask a question that stimulates classmates’ curiosity, signaling you to prepare or launch an inquiry activity. But this is rarely the case. And you shouldn’t wait for it. Rather, you can initiate an inquiry activity when you feel it is appropriate. But it must use a guiding question that:
- Reflects a core curriculum concept
- Has engaged students from past or other classes
- Interests students, as indicated in previous lessons and discussions
The question’s source, whether from you or your students, is secondary.
7. Run a Check-In Afterwards
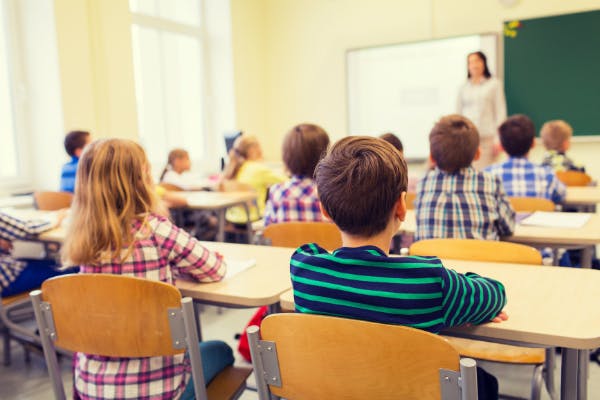
Allotting time for class-wide reflection lets students discuss challenges and discoveries, filling knowledge gaps and supplementing findings. This prepares them for future lessons and inquiry activities. They’ll learn about an array of ideas to consider throughout their study of the specific topic, and strategies to try during the next exercise. It can be especially helpful for learners who struggle in small groups, giving them a different way to process the activity’s outcomes.
Wrapping Up this Guide to Inquiry-Based Learning
Armed with a thorough understanding of inquiry-based learning and the strategies you need to run activities, your students should see benefits. These not only include an improved understanding of curriculum concepts and the development of transferable skills, but -- according to research and proponents -- a greater appreciation for learning’s inherent rewards. This, in itself, should create a more engaged classroom. Try Prodigy Math Game — the adaptive, curriculum-aligned math platform that helps students love learning math teachers can use Prodigy to:
- Align in-game adventures with classroom lessons
- Collect insights into student progress and learning gaps
- Send differentiated content and Assessments in just a few clicks
- Our Mission
Bringing Inquiry-Based Learning Into Your Class
A four-step approach to using a powerful model that increases student agency in learning.
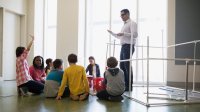
Adopting an inquiry-based learning (IBL) approach in my classroom has been the most meaningful change I have made in my teaching. The benefit of increased student agency in learning, the authentic connections we make to the world around us, and the 21st-century skills IBL nurtures are great reasons to explore how inquiry can enhance what you are doing in your classroom.
But with great opportunity come challenges all educators should consider before diving into inquiry. Perhaps one of the greatest mistakes the inquiry teacher can make is to give too much agency over learning to learners too soon. Many teachers get so inspired by the Free Inquiry process I share at conferences, as well as the demonstrations of learning students produce, that they have their students dive right into Free Inquiry when they make this powerful change in their teaching. In my experience, without scaffolding students will not feel as confident or supported through their inquiry journey.
The Types of Student Inquiry
The Types of Student Inquiry are a scaffolded approach to inquiry in the classroom, one that gradually increases student agency over learning while providing learners with the necessary skills, knowledge, and understanding to be successful in their inquiry.
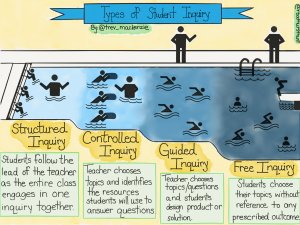
In the shallow end of the Types of Student Inquiry pool, Structured Inquiry gives the teacher control of the essential question, the starting point—for example, “What defines a culture?” or “What is the importance of the scientific method?” These questions are not answered in a single lesson and do not have a single answer, and, in fact, our understanding of an essential question may change over time as we research it. In Structured Inquiry, the teacher also controls specific learning activities, the resources students will use to create understanding, and the summative assessment learners will complete to demonstrate their understanding.
In Controlled Inquiry, the teacher provides several essential questions. Learners unpack several resources predetermined by the teacher to provide valuable context and rich meaning relative to the essential questions. All learners typically demonstrate their understanding using the same summative assessment. In Guided Inquiry, the teacher further empowers student agency by providing several essential questions, having the students select the resources they will use to research their answers, and allowing them to choose how they will demonstrate understanding. Student agency over learning comes through this selection of resources and the summative assessment.
And finally, in the deep end of the inquiry pool, Free Inquiry allows learners, with the support of the teacher, to construct their own essential question, research a wide array of resources, customize their learning activities, and design their own summative assessment to demonstrate their learning.
How Are the Types of Student Inquiry Helpful?
Inquiry is most successful when strongly scaffolded. The Types of Student Inquiry act as a scope and sequence to support learners in their journey toward Free Inquiry. In my classroom, we begin in a Structured Inquiry model, transition to a Controlled Inquiry unit, move on to Guided Inquiry, and if all goes well, conclude in Free Inquiry. These four types of inquiry make up our time together in the course.
This structure allows us to successfully address the curriculum and the “must know” content and skills of each discipline, grade level, and course. In the Structured, Controlled, and Guided units, I plan to achieve specific learning objectives and unpack particular resources in order to best prepare my learners for whatever summative assessment they will see at the end of our time together. Whether it’s a provincial, state, or governing body exam or the SAT, I ensure that this material is learned during the types of student inquiry I have more control over.
Students should feel connected to their learning, certain about how to plan their inquiry, and comfortable with its responsibility. The Types of Student Inquiry structure our coursework and learning in a gradual release of control model, one where students learn essential inquiry skills throughout the year rather than being thrown into the deep end of the inquiry pool right away.
Each of my students has a copy of the swimming pool illustration above, and it hangs on our classroom wall. It illustrates the gradual increase of student agency our inquiry journey will provide, the changing skill set the inquiry learner will gain, and the ever-transforming role of the teacher. It also allows me to speak to the questions, concerns, or anxieties students may encounter in their inquiry. The swimming pool analogy illustrates that we won’t go anywhere in the pool until the learner is prepared to do so and that I’m always there to provide support, whether it be in the pool or facilitating from the side.
How to Move Forward in Adopting Inquiry
First, look through the lens of your learners when considering how you want to shape your classroom. Keep them at the heart of your decisions. Think of them as you plan what inquiry will look like in your classroom, imagine how they will respond to the gradual increase in agency, and question how you can best meet their needs.
Second, think big and start small. Take some time to imagine the inquiry classroom you want in a few years and begin to plan to make this dream a reality. Start with a unit you love or that you’ve seen resonate with your learners. Revamp it to begin with an essential question and frame it in one of the Types of Student Inquiry. At the end, reflect with your learners on how it went.
And last, try to become comfortable with the mess of uncertainty. Even with the scaffolding of the Types of Student Inquiry and the many structures I have in place to support increasing student agency, inquiry doesn’t offer a clear destination. What essential questions learners ask, what resources they will unpack, and what new understandings they create are all initially unknown when we begin our inquiry journey. Be prepared for this and for your learners to need support in adopting inquiry as their own.
Inquiry and Problem Solving
Inquiry and problem solving refer to an array of learner-centered processes that facilitate deep engagement with a question or problem and strategies to develop subsequent solutions and explanations.
With any approach to inquiry and problem-solving, students follow a series of phases or specific discipline-based practices to take them through an intentional process. These phases or practices are not necessarily completed in a linear or lock-step manner, but provide some structure for students to address a question, issue, problem, or need.
Common elements of inquiry and problem-solving include:
Asking questions or defining problems
Exploring solutions or explanations
Analyzing or testing solutions or explanations
Communicating or taking action on solutions or explanations
The elements are most effective when applied in an iterative cycle, so that students have the opportunity to revise their solutions and explanations based on utility, analysis, and feedback. Here's how different sets of discipline-based practices and inquiry models can align to the four common elements of inquiry.
Inquiry Elements
Scientific and Engineering Practices
Standards for Mathematical Practice
Inquiry Arc
Design Thinking
Asking questions and defining problems
Make sense of problems and persevere in solving them
Developing questions and planning inquiries
Empathize with users
Define the problem
Planning and carrying out investigations
Ideate potential solutions
Prototype solutions
Developing and using models
Analyzing and interpreting data
Using mathematics and computational thinking
Constructing explanations and designing solutions
Model with mathematics
Reason abstractly and quantitatively
Use appropriate tools strategically
Attend to precision
Look for and make sense of structure
Look for and express regularity in repeated reasoning
Applying disciplinary concepts and tools
Evaluating sources and using evidence
Test solutions
Engaging in argument from evidence
Obtaining, evaluating and communicating information
Construct viable arguments and critique the reasoning of others
Communicating conclusions and taking informed action
Implementation of solution
Inquiry and problem-solving opportunities encourage students to engage with relevant concepts and skills within authentic, real-world contexts. Research suggests that combining real-world application with sustained participation in inquiry and problem solving experiences can lead to increased student engagement and support deeper learning of concepts and skills. Additionally, opportunities for inquiry and problem solving can help students develop:
- Professional skills - e.g. communication, professionalism, collaboration, and empathy;
- Innovative mindsets - e.g. creative, ‘out of the box’ thinking; and
- Metacognition - e.g. self-regulation and self-monitoring of their learning progress
Developing these skills prepares students for college, career, and community success, and help students meet the HIDOE general learner outcomes . To further examine the benefits of inquiry-based learning, see the following resources:
Center for Inspired Teaching
Review of Research on Inquiry-Based Learning
Inquiry-Based Teaching in World Languages
Inquiry-Based Models
Students can engage in deep learning of concepts and skills through a variety of inquiry-based models, including: instructional approaches, instructional sequences, and/or design processes.
- Instructional approaches - models of inquiry that are designated by essential features more so than one consensus sequence of events
- Instructional sequences - models of inquiry that have a specific sequence of events based on learning theory or practices of a discipline
- Design processes - models of design based on an iterative process of prototyping and testing to develop a final product
Examples of inquiry-based models, their essential features and ideas for getting started with them are presented below:
Instructional Approaches
- Project-Based Learning: Essential Features | Getting Started
- Problem-Based Learning: Essential Features | Getting Started
- Place-Based Learning: Essential Features | Getting Started
Instructional Sequences
- 5E Instructional Model: Essential Features | Getting Started
- Inquiry Design Model: Essential Features | Getting Started
- Gather - Reason - Communicate: Essential Features | Getting Started
Design Processes
- Engineering Design Processes: Essential Features | Getting Started
- Design Thinking: Essential Features | Getting Started
Considerations for Implementing Inquiry-Based Learning
Whatever process or model is chosen to engage students in inquiry and problem-solving, it is important to consider different approaches to structured vs open inquiry . For many students and at earlier grade levels, a scaffolded approach is ideal when students first encounter an inquiry investigation. Considering a variety of scaffolding and differentiation approaches will ensure that students’ different learning needs are addressed. Over time, with more practice, the teacher can gradually release decision-making and control to the students. It is also helpful for the teacher to consider the types of questions they will ask students to support their inquiry process.
Lesson and Activity Resources
The following sites showcase examples of learning experiences that are inquiry- and problem-based.
All Content Areas
Visible Thinking : A site with a variety of thinking routines that can be used to scaffold students’ inquiry and problem solving skills.
Design Thinking : Resources for facilitating design thinking with students curated by Hawai‘i educators.
Mathematics
Making Sense of Problems : A site with examples of classroom practice of the standards for mathematical practice, including, “Making sense of problems and persevere in solving them.”
Hawai‘i educators developed inquiry-based STEM Units that align to the FAIR features of STEM learning experiences .
Social Studies
C3 Hawai‘i Hub : Provides inquiry lessons for social studies developed by Hawai‘i educators using the inquiry design model.
Science: GRC Lessons
Provides inquiry lessons for science developed by Hawai‘i and other U.S. educators using the Gather - Reason - Communicate Approach and 5E instructional sequence.
Back to School Design
BACK TO HOME
Activity Ideas / Math / Planning & Teaching
How to Teach Math Using Inquiry-Based Learning
Teaching math has slowly been evolving from a subject that, for many students, feels useless and trivial, to one that helps provide relevance and build important learning skills inside and outside of the classroom. Inquiry learning helps provide students with opportunities to develop critical thinking, teamwork, and resilience – skills that are absolutely essential to be “good” at math.
Teaching math using inquiry-based learning is a powerful way to reinforce the skill of problem-solving for students. In the inquiry math classroom, students are not passive recipients of formulas and facts, but are instead encouraged to ask questions, investigate rules and patterns, and persevere when confronted with challenges. Using an inquiry approach in math helps build important skills such as resilience, problem-solving, and perseverance.
The post below outlines some ways to incorporate an inquiry-based approach into your math classroom (or within your lessons). It also provides some examples of inquiry and PBL projects to help your students gain confidence, build good learning habits, and apply their skills.
Traditional vs. Inquiry-Based Approaches
There’s been a lot of debate about using a “back to basics” traditional approach or an inquiry-based approach when teaching math. Traditional math teaching typically follows a structured curriculum with a focus on rote memorization, guided procedures, and step-by-step instructions, which many would describe as providing a solid foundation for students to build mathematical skills. On the other hand, inquiry-based math emphasizes exploration, critical thinking, and problem-solving through open-ended tasks. Neither of these approaches are wrong.
In my own classrooms, I’ve often opted for a combination of approaches. Recognizing the strengths of each method and using them complementary to each other has resulted in the best outcomes for my students in the past. Traditional math works very well for students who need the reliability of steps and formulas to grasp numerical concepts and skills. Inquiry-based methods work well for students when they need to apply these concepts and skills.
Encouraging students to apply their skills and understanding in the “real world” and helping them learn to make connections between concepts allows them to develop a deeper understanding of math. Combining the two approaches can create a balanced and well-rounded math education, and equip students with not only the procedural knowledge they need, but also the necessary problem-solving skills to apply this knowledge in other contexts.
Why should you use an inquiry approach in math?
For most of us, we’re used to math being presented as a set of rules and procedures we follow to arrive at a correct answer. However, this is usually far from the way math is applied in the real world. A good example is when scientists and climatologists are studying weather patterns – they might ask something like, “how much rain can we expect from this hurricane?”. From there, meteorologists and atmospheric scientists combine data to create models to help approach the question. They apply their understanding of mathematical principles to situations to determine the correct answer, then see if their solution applies in the real world. If not, it’s back to the drawing board.
Framing math in this kind of way, as opposed to the traditional “memorize the formula, practice some questions, then repeat”, can be far more effective. Some of the benefits include:
- Emphasizing the process as opposed to the final product
- Finding several different approaches and choosing the one(s) that work for you
- Seeing math from a more holistic viewpoint
- Teaching students that mistakes are where we learn the most
- Reducing the stigma that math is “hard”, “useless”, or “irrelevant”
- Building critical thinking habits and resilience to persevere through difficulties
- Having the ability to transfer skills to all types of mathematical problems
- Learning more deeply and in a more problem-based way
Below are some unique ways to incorporate an inquiry focus into your math lessons. These ideas can be used as stand-alone projects or as complements to your traditional lessons. They also work well as projects that call for the application of new learning.
Math Inquiry Idea #1 – Urban Planning and Sustainable Cities
For this inquiry idea, students play the role of an urban planner tasked with designing a new layout for a sustainable city. To start, discuss the concepts of urban planning and sustainability.
Ask students how the two are related and ask them what characteristics a sustainable city has. Some characteristics include:
- Reliance on renewable energy (such as wind, solar, and smart grid technologies)
- Plenty of green spaces and biodiversity
- Examples of urban agriculture, such as community gardens
- Climate change adaptation
- Efficient transportation and waste management services
- Mixed-use development
- Community engagement and participation
Spend some time discussing and envisioning what a sustainable city would look like. Allow them to utilize videos, books, and maps to explore their ideas.
Suggested resources:
- Green City: How One Community Survived a Tornado and Rebuilt for a Sustainable Future (hardcover, ages 5-8)
- Sustainable Development Goals (PDF from the United Nations for your learning wall)
- How Cities Work (Lonely Planet hardcover/interactive book)
- Climate Change and Sustainable Cities (NRDC)
Provide students with resources that highlight real-world examples of sustainable urban planning projects. Guide them to explore how these cities are laid out and where the major roads and buildings are. Are there green spaces? Where are they? Where could they go? Are there clear public transportation links? Could unused space be utilized in a more efficient way?
Once students have an idea of what features they’d like their city to have, it’s time to draw. Using paper or computers, have students create a map that lays out their city and all of its features. Utilize geography concepts like scale, elevation, and topography so that students understand the connection between math and other subjects. Encourage students to use materials like cardboard, wood, foam, or recyclable materials to bring their maps to life.
Provide students opportunities to reflect on their learning and the challenges they encountered during the design process. Ask questions about the application of mathematical principles and how they helped students with things like scale, measurement, and spacing. Facilitate discussions that encourage problem-solving and allow other students to respectfully ask questions about the sustainability features they see. Prompt students to consider the trade-offs and compromises they had to make in their decisions.
Incorporating Mathematical Concepts
There are a few different ways that math can be applied to this inquiry project:
1. Creating scale models
Determine the scale at which you want to create the model city. Common scales include 1:100, 1:200, or 1:500. Encourage students to plan a layout for the city, including streets, buildings, parks, landmarks, and other features of a sustainable city. Consider the use of grids and geometric shapes for simpler planning. or use pattern blocks to map out the features of the city.
2. Simple calculations
Planning a sustainable city requires the calculation of things like distance, areas, and volume in order to optimize for land use, transportation routes, and building placements. For example, calculating the distance between a major airport and the city centre, or calculating the amount of rainwater that a barrel can hold.
3. Statistical analysis
Through examining articles of pre-existing cities, students gather and analyze demographic data, population trends, and socioeconomic indicators to identify patterns. These can then be used to inform decisions along the way. Students could also conduct surveys to find out what features their classmates would want in a sustainable city and analyze that data too.
4. Graphing and equations
If students conduct surveys or collect forms of data from their peers, they can create formulas for tracking things like future population growth, resource demand, and infrastructure needs. They could also predict greenhouse gas emissions or energy consumption trends too. Encourage students to graph their results so the data can be understood simply.
5. Financial costs
Building a sustainable city isn’t cheap. Discuss the cost of materials, paying workers, and other variables that go into the construction of a city. Older students may choose to calculate the cost-effectiveness of sustainable initiatives and projects by using financial metrics, or conduct a risk assessment to evaluate the probability of things like natural disasters and climate-related events.
Related Reading :
Solving the Problems of Vacant Spaces and Empty Rooftops (ideas #2 and 3) Blueprints for a Greener Footprint PDF (WEF) The City in 2050: Creating Blueprints for Change (ULI) SmartAfrica Blueprint (PDF)
Math Inquiry Idea #2 – Applying Mathematics to Cryptography
It’s no surprise that coding and math share a lot of similarities. This inquiry idea is suitable for older students studying computer science and other high-school level mathematics or coding courses.
Cryptography is the science of using math to hide data behind encryption. This helps store, secure, and protect communication. Math and cryptography share a deep connection because math is what provides the foundation for creating secure cryptographic systems.
Most cryptographic systems combine two things:
1. A set of rules that specify the mathematical steps needed to encipher or decipher data (also known as a process or algorithm) 2. A cryptographic key (a string of numbers of characters), or keys
In simpler terms, imagine you had a recipe for baking a cake. In the same way the recipe tells you the steps to follow to bake the cake, a cryptographic algorithm is a set of rules that tells a computer how to turn secret information (plaintext) into scrambled information (ciphertext).
Imagine you put that recipe in a locked box. Having the key to unlock that secret box is like having a cryptographic key, which is a string of numbers or characters that the algorithm uses to scramble and unscramble your data. Without the right keys, you can’t get into the box.
So, when you combine the algorithm with the cryptographic key, it’s like following a secret recipe with a special key to unlock and lock your secret message. The key is what keeps your recipe safe, and the algorithm is the set of rules that keeps everything secure.
Inquiry Projects Involving Cryptography
1. self-guided inquiry.
Students can explore the various theories involved in encryption schemes, including:
- Diffie-Hellman key exchange scheme
- Chinese remainder theorem
- Probability theory
- The RSA encryption algorithm
- Complexity theory
- Elliptic curve cyptography
- EIGamal encryption system
- Information theory
Challenge students to not only understand one of the above theories, but to simplify it so that younger students can understand. Similar to the cake example, students use their knowledge and experiment with different analogies to help others understand in an easy-to-understand way. Students can choose to share their information as a presentation, workshop, or through a demonstration.
2. Simple ciphers
Show students simple substitution ciphers, such as:
Caesar cipher – each plaintext letter is replaced with a different one a fixed number of places down the alphabet. For example, a left shift of three places, as seen below:
Pigpen cipher – each plaintext letter is replaced with a simple geometric picture symbol (created using grids and crosses so that each letter is represented by fragments of a grid or cross with or without a dot)
Provide them with decoding charts and encourage them to encode and decode their messages using these ciphers. For example, provide math equations where each answer corresponds to a letter in the alphabet, and challenge students to decode the message by solving the equations. These types of problems utilize deductive reasoning and problem-solving skills as well as helping to solidify basic concepts such as addition, subtraction, multiplication, and division.
Related Reading:
Guide to Cryptography Mathematics Explorer Academy: Code-Breaking Activity Adventure (National Geographic) Code Cracking for Kids (Codes and Ciphers) Cryptography as a Teaching Tool
Math Inquiry Idea #3 – Sports and Statistics
While not uncommon in the math classroom, sports and statistics can help foster critical thinking skills in a way that appeals to a lot of students. By encouraging students to explore the connection between math and sports, they get the chance to see how mathematical concepts apply to familiar, real-life situations.
Of course, not every student is interested in sports. In these cases, it helps to point out the fact that sports aren’t just about the rules, plays, and competition. Instead, merging sports and mathematics offers students new and interesting ways to apply their learning in strands such as geometry, statistics, algebra, and measurement.
To get students thinking about the intersection of math and sports, consider starting off by discussing the importance of statistics and how they are used in sports such as basketball, soccer, baseball, or football.
Students may suggest the following points:
- Statistics help track player performance and evaluation (helpful for managers and scouts to assess a players’ strengths and weaknesses)
- They allow coaches and managers to notice trends and patterns in team dynamics (which helps identify areas for improvement or adjustment)
- The use of statistics helps coaches and team members make strategic and informed decisions (helpful in making lineup changes, substitutions, and set goals)
- Statistics also help enhance fan engagement by providing valuable insight into the dynamics of the game (which fuels discussions and debates within the sports community)
Encourage students to generate questions and inquiries related to sports statistics. Prompt them to think about what types of data are collected in sports, and what they’re curious about in general. Provide them with time and opportunities to research statistics for players or sports they’re interested in. Statistics can come from a variety of courses, such as online databases, sports websites, and historical data archives. Guide students in collecting and organizing data on player and team stats, game scores, and other relevant metrics.
From here, students can take their learning in several directions. Some suggestions are below:
1. Drawing comparisons
Students love making comparisons, whether between each other, celebrities, sports stars, or their favourite sports team. In a statistics inquiry, encourage students to make comparisons between different players, teams, or seasons using measures such as averages, percentages, ratios, and rates. For example, students can compare the shooting percentages of basketball players, betting averages of baseball players, or the win-loss records of sports teams.
Guide students in exploring mathematical concepts and principles embedded in these statistics. Prompt them to ask inquiry questions to guide their own learning. One example might be, “how did Tom Brady’s passing yards change from when he played for New England vs. when he played for Tampa Bay?” Here are some other examples:
- How does a player’s shooting percentage affect their team’s overall performance?
- What factors influence a team’s success in a particular sport?
- How do player statistics change over the course of a season?
Facilitate investigations by helping students analyze their data, generate hypotheses, conduct experiments, and draw conclusions based on their findings. Encourage students to share their learning and insights through various inquiry-based presentation formats, including traditional presentations, discussions, the use of charts or graphs, and multimedia presentations. Ensure they are explaining their mathematical reasoning and encourage peer feedback.
2. Creating a “Statistics Palette”
Have you ever heard of “movie palettes”? Basically, it’s a set of specific colours used throughout a particular movie or film. They’re carefully curated to evoke specific moods and feelings, convey thematic elements, and enhance storytelling. Movie palettes are relatively recent and can be purchased as a unique piece to showcase your favourite movie. During the process, the dominant colour from each scene of your favourite movie is turned into a vertical strip, and arranged chronologically, side-by-side, onto a canvas. This results in a unique piece of artwork that represents your favourite movie as a colour palette.
Students can apply this idea to a sports statistics inquiry in math by assigning colours to specific data categories. For example, examine Tom Brady’s passing yards when he played for the New England Patriots and compare them to his passing yards when he played for the Tampa Bay Buccaneers. Group his statistics by number of yards (for example <1000 per season, 1000-1500 per season, etc.) and assign each group a colour, shown below:
The United States of Sports (Sports Illustrated for Kids) It’s a Numbers Game! (Co-authored by Patrick Mahomes) Sports Reference (website full of up-to-date sports statistics)
Math Inquiry Idea #4 – Geometry in Architecture
This inquiry idea is great because it allows teachers to facilitate learning in a cross-curricular way , often incorporating several subjects. To start, give students the opportunity to explore the history and significance of geometry in architecture, from ancient civilizations to the contemporary world. Students can examine great architectural wonders of the past, including:
- The Great Pyramid of Giza (Egypt, 2560 BCE)
- The Hanging Gardens of Babylon (Iraq, 600 BCE)
- The Colossus of Rhodes (Greece, 280 BCE)
- Chichen Itza (Mexico, 700 AD)
- The Taj Mahal (India, 1648 AD)
By examining architectural masterpieces from the past, students will gain an appreciation for the role of geometry in creating these structures. As facilitator, help students to identify geometric shapes and principles found within these structures and explore how proportions, patterns, and culture influence architectural design and construction processes.
Recognizing Historical Successes
A great example is the use of geometry by the Inca at Machu Picchu, where they built intricate terraces and staircases, both for agricultural purposes and in order to navigate the steep Andean terrain. In this case, geometry helped them design and construct these structures with precision. Show students a photo of the staircases and terraces, and ask students to identify their characteristics; for example, the terraces follow the contours of the mountainside, and the staircases were engineered to conform to the natural topography of the landscape.
Once students feel comfortable identifying these characteristics, have them explore the use of water irrigation systems and aqueducts. Encourage them to make inferences about how the architectural design of these systems resulted in optimization water distribution, minimal erosion, and the conservation of natural resources.
As students continue to gain confidence in exploring these architectural features, encourage them to use more precise mathematical language. For example, instead of saying that the Inca “made steep paths”, they can say that the Inca “constructed steep slopes”.
Solving Modern-Day Problems
Once students understand the link between geometry and construction, and have had some time to explore the impact of mathematics on construction, challenge them to apply their understanding to real-world design challenges and problems. This is where a wonderful intersection of subjects – math, design, engineering, physics, and environmental science – occurs. Students may be tasked with designing and construction scale models of popular landmarks, exploring principles of stability and load-bearing, or analyzing the benefits of using specific shapes such as arches, domes, and columns.
A good application of these skills is to tackle the challenge of how to create safe and stable wildlife crossings over busy roads and highways. This is a problem that a lot of students show interest in because they want to see animals be able to coexist safely in our modern world. The use of bridges, tunnels, overpasses to allow animals to safely cross roads and highways requires problem-solving skills and spatial thinking in addition to mathematical thinking. A good place to start is by getting familiar with the Banff Wildlife Crossings Project , which has reduced animal-vehicle collisions in the province of Alberta by more than 80% since its construction.
The use of manipulatives such as pattern tiles, tile boards, and geoboards ( available in a set of 6 here ) help students to connect the building process with geometric concepts. Encourage them to explore how shape, symmetry, and proportion work together to create stunning buildings, homes, and other architectural wonders. Challenge students to sketch their ideas, incorporating geometric elements such as a variety of shapes, angles, patterns, tessellations, and symmetry in their designs.
Teachers can prompt students to consider other elements, such as:
- Proportions and measurements of windows, doors, and other features
- Sustainable and eco-friendly features, such as solar panels, wind turbines, rainwater harvesting, living walls, green roods, and natural ventilation
- Aesthetics (for example, creating building that mesh nicely with the culture and traditions of a particular place)
Once students have visualized their ideas, they are encouraged to display their learning in a way that is most meaningful to them. For example, if they sketched a scale-drawing of a wildlife bridge, the next natural step would be to create a physical model or prototype to demonstrate how it would look in real-life. Some may choose to conduct a gallery walk, showing their peers and others their idea progress through rough sketches and blueprints. Regardless of their presentation method, students should be encourage to provide feedback on both their own work and their peers’ work. Review sessions can be conducted to facilitate the exchange of constructive feedback and suggestions for improvement, which are a natural part of the inquiry process.
Related reading:
Wildlife Crossings (National Geographic article) The Wallis Annenberg Wildlife Crossing Wildlife Crossings and Fish Passages (AIL)
Final Thoughts
Fusing math and inquiry learning in the classroom is important in all 21st century classrooms. An inquiry-based approach encourages students to ask questions, explore mathematical principles, and see how math relates to many different aspects of their lives. By allowing students to make connections and participate in authentic learning, they will hopefully develop an appreciation for the relevance and applicability of mathematics in everyday life, even if they are not keen to pursue a job that requires extensive math knowledge.
Key Takeaways:
(1) Teaching math using inquiry-based learning is a powerful way to reinforce the skill of problem-solving for students
(2) Applying inquiry-based learning in math doesn’t have to be a “one or the other” approach. Rather, you can combine elements of traditional math teaching and incorporate tenets of inquiry along the way to solidify concepts and apply learning in new contexts
(3) Using scenario-based learning or delving into real-life problems that involve math helps build problem-solving and communication skills, as well as perseverance in students of all ages
(4) Employing a cross-curricular approach allows students to appreciate the applicability of math in everyday life
(5) Inquiry-based learning in math empowers students to take ownership of their learning and draw conclusions independently or collaboratively
Cover photo by Alena Darmel
Have you used any of these math inquiry project ideas in your classroom? Do you have any other ideas you’d like to share? Feel free to comment down below, or browse some more ideas on Pinterest !
- Previous story How to Set Up Learning Provocations in the Classroom
Leave a Reply Cancel reply
Your email address will not be published. Required fields are marked *
Activity Ideas / Math
Exciting Opportunities for Inquiry Learning in the New Ontario Math Curriculum
1 Jul, 2020
Planning & Teaching / Social Studies
How to Integrate Ontario Curriculum Expectations with Inquiry Learning
15 Jun, 2021
Activity Ideas / Citizenship / Inquiry Skills / Planning & Teaching / Science / Social Studies
Your Complete Guide to Using Scenario-Based Learning in the Classroom
15 Oct, 2020
Planning & Teaching / Starting with Inquiry Learning
Helping Students See The Value in Their Contributions
27 Nov, 2020
Inquiry Skills / Starting with Inquiry Learning
20 Guaranteed Ways You Can Tell Inquiry-Based Learning is Working
28 Mar, 2020
9 Feb, 2024
Privacy Overview
Automated page speed optimizations for fast site performance
Primed To Learn
Embark on Your Learning Journey
Inquiry-Based Learning: 11 Benefits of Fostering Curiosity and Critical Thinking
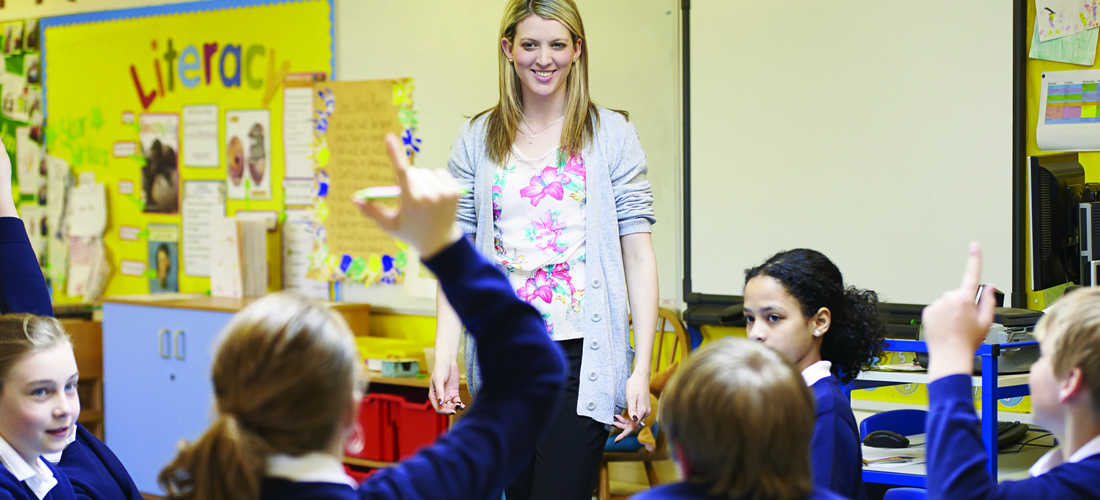
Inquiry-based learning is a dynamic and immersive educational approach that is reshaping classrooms worldwide. This pedagogical strategy turns the traditional model of instruction on its head by encouraging students to lead their own learning journey, fostering curiosity, exploration, and critical thinking skills. This blog post will delve into the principles and benefits of inquiry-based learning, providing valuable insights for educators seeking to invigorate their teaching methods and inspire their students.
Nurturing curiosity, critical thinking, and problem-solving skills is crucial in fostering Inquiry-Based Learning. This pedagogical approach encourages students to question, explore, and make sense of the world around them. By doing so, it instills a lifelong love for learning, cultivates intellectual curiosity, and empowers learners to seek knowledge independently.
Critical thinking enables students to analyze information objectively and make reasoned judgments, fostering intellectual independence. Equally essential are problem-solving skills, which equip learners with the ability to devise effective solutions to challenges, promoting resilience and adaptability. Hence, nurturing these skills not only enriches the learning experience but also prepares students for a complex, rapidly-changing world.
Benefits of Inquiry-Based Learning
Inquiry-Based Learning (IBL) offers a wide range of benefits that contribute to a more effective and engaging educational experience for students. These benefits go beyond rote memorization and standardized testing, focusing on fostering skills and attitudes that are crucial for success in the modern world. Here are some of the key benefits of IBL:
1. Fostering Curiosity and Engagement
Inquiry-based learning taps into students’ natural curiosity by presenting them with intriguing questions and real-world problems to solve. This approach ignites their interest, driving them to explore topics in-depth and stay engaged throughout the learning process.
2. Developing Critical Thinking Skills
Inquiry-based learning encourages students to think critically and analytically. They must evaluate information, analyze evidence, consider multiple perspectives, and make informed decisions. These skills are essential for making sense of complex information and navigating the challenges of the 21st century.
3. Promoting Problem-Solving Abilities
By grappling with open-ended questions and real-world challenges, students develop strong problem-solving skills. They learn to approach problems systematically, apply creative thinking, and devise effective solutions – a valuable skill set for both academic and real-life scenarios.
4. Encouraging Active and Self-Directed Learning
Inquiry-based learning places students in the driver’s seat of their education. They actively seek out information, conduct research, and construct their understanding. This self-directed learning approach equips students with the ability to take ownership of their education beyond the classroom.
5. Building Communication and Collaboration Skills
Collaborative learning is integral to Inquiry-based learning. Students work together, exchange ideas, and engage in discussions to solve complex problems. This enhances their communication skills, teaches them how to collaborate effectively, and prepares them for teamwork in diverse settings.
6. Enhancing Information Literacy
In an era of vast information availability, IBL teaches students how to find reliable sources, critically evaluate information, and distinguish between credible and unreliable content. These skills are essential for making informed decisions in a data-rich world.
7. Cultivating Lifelong Learning Habits
Inquiry-based learning nurtures a love for learning that extends beyond formal education. Students develop the mindset and skills to seek out information, ask questions, and continue learning throughout their lives, adapting to new challenges and opportunities.
8. Empowering Creativity and Innovation
Inquiry-based learning encourages students to think outside the box, experiment with ideas, and generate innovative solutions. This creative approach helps students develop an entrepreneurial spirit and adapt to rapidly changing industries and technologies.
9. Increasing Retention and Deep Understanding
Inquiry-based learning promotes meaningful learning by connecting new information to existing knowledge and personal experiences. This approach enhances retention and fosters a deeper, more comprehensive understanding of concepts.
10. Preparation for Real-World Challenges
Inquiry-based learning simulates real-world problem-solving scenarios, better preparing students for the challenges they will face in their personal and professional lives. The skills developed through IBL are directly applicable to a wide range of situations.
11. Boosting Intrinsic Motivation
Engaging in self-directed exploration and problem-solving inherently boosts students’ intrinsic motivation to learn. The sense of accomplishment that comes from finding solutions to complex questions or issues serves as a powerful motivator.
Incorporating Inquiry-based learning into education nurtures well-rounded individuals equipped with the skills, attitudes, and habits necessary for success in a rapidly changing world. By fostering curiosity, critical thinking, and collaboration, IBL empowers students to become lifelong learners and active participants in their own education.
Understanding Inquiry-Based Learning
Inquiry-Based Learning (IBL) is a dynamic and student-centered approach to education that encourages learners to seek answers to their questions through exploration and investigation. In this framework, education becomes less about memorizing facts, and more about constructing knowledge and understanding through the process of inquiry. In essence, IBL puts the student in the driver’s seat of their learning journey, stimulating curiosity, critical thinking, and problem-solving skills.
The role of questions in this learning process cannot be overstated. Questions serve as the catalysts that ignite the spark of curiosity in learners, inspiring them to embark on a quest for knowledge. These are not merely answers to be found, but challenges to be unraveled. They provide direction to the learning process, guiding learners as they navigate the vast seas of knowledge. Every answer found opens up new questions, keeping the cycle of inquiry alive, and establishing a lifelong love for learning.
Key Features of Inquiry-Based Learning
Inquiry-Based Learning (IBL) is characterized by several key features that distinguish it from traditional teaching methods. These features emphasize active engagement, critical thinking, and student autonomy in the learning process. Let’s discuss these features in more detail:
1. Open-Ended Questions and Problems
In IBL, learning begins with thought-provoking questions or real-world problems that do not have a single correct answer. These questions encourage students to explore, research, and investigate various perspectives and solutions, fostering deeper understanding and curiosity.
2. Student Autonomy and Ownership of Learning
IBL places students at the center of their learning journey. They have the freedom to choose their topics, formulate their own questions, and design their research strategies. This autonomy cultivates a sense of responsibility for their education and enhances intrinsic motivation.
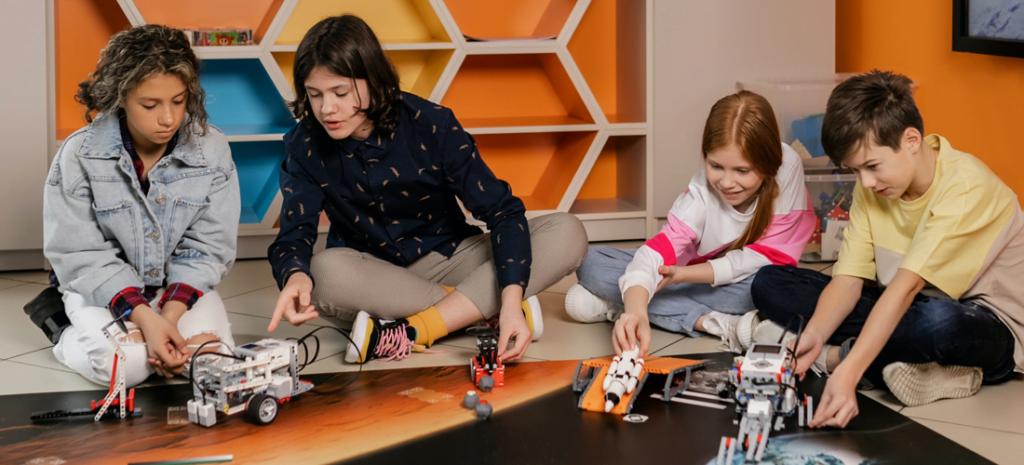
3. Collaborative Learning and Peer Interaction
Collaboration is a crucial component of IBL. Students are encouraged to work together in groups, share ideas, discuss findings, and provide feedback to one another. This collaborative environment mirrors real-world scenarios and promotes teamwork and effective communication skills.
4. Active Engagement and Exploration
IBL encourages active participation and hands-on experiences. Students engage in experiments, fieldwork, research, and projects that require them to apply theoretical concepts to practical situations. This active involvement deepens comprehension and helps retain knowledge.
5. Real-World Relevance
IBL connects classroom learning to real-life contexts. By addressing authentic problems or issues, students recognize the relevance of their studies in the broader world. This approach enhances motivation and helps students see the practical applications of what they are learning.
6. Critical Thinking and Problem-Solving
Critical thinking is at the heart of IBL. Students are challenged to analyze information critically, evaluate evidence, make informed judgments, and develop creative solutions to complex problems. This cultivates higher-order cognitive skills essential for lifelong learning.
7. Iterative Process of Inquiry
IBL is not a linear process; it involves iteration and refinement. Students continually refine their questions, hypotheses, and methodologies based on new information and insights gained during their investigation. This iterative approach mirrors the dynamic nature of real-world problem-solving.
8. Instructor as a Facilitator
In IBL, educators take on the role of facilitators rather than authoritative lecturers. They guide students’ inquiries, provide resources, and support the learning process, while allowing students to take the lead in their exploration.
9. Interdisciplinary Connections
IBL often encourages interdisciplinary learning, as students explore topics that cross traditional subject boundaries. This approach highlights the interconnectedness of knowledge and prepares students to address complex, multifaceted challenges.
10. Emphasis on Process and Reflection
The learning process itself is emphasized in IBL, not just the final outcomes. Students engage in reflection, self-assessment, and metacognition, helping them understand how they learn, what strategies are effective, and how to improve their learning skills over time.
Incorporating these key features of IBL into teaching practices can have a transformative impact on education. By fostering curiosity, critical thinking, collaboration, and independent learning, IBL equips students with the skills and mindset needed to thrive in an ever-evolving world.
Implementing Inquiry-Based Learning
Implementing Inquiry-Based Learning (IBL) involves creating an environment that supports student-driven exploration, critical thinking, and active engagement. Here are some key strategies and methods for successfully implementing IBL in the classroom:
1. Design Thought-Provoking Questions
Start with open-ended questions that stimulate curiosity and prompt investigation. These questions should encourage students to explore, analyze, and seek out information to develop their understanding.
2. Choose Real-World Problems
Select problems that are relevant to students’ lives and have real-world applications. This helps students see the value and significance of their learning and motivates them to find solutions.
3. Provide Autonomy and Choice
Allow students to choose topics or areas of interest within a broader theme. Giving them ownership of their learning empowers them to take responsibility for their education and explore areas they are passionate about.
4. Structure Inquiry Phases
Guide students through the inquiry process by breaking it into manageable phases. These phases might include asking questions, researching, analyzing data, brainstorming solutions, and presenting findings.
5. Utilize Multiple Learning Resources
Offer a variety of resources such as books, articles, videos, and online platforms to support students’ research. Encourage them to explore diverse sources and evaluate their credibility.
6. Facilitate Collaborative Learning
Foster a collaborative environment where students work in groups to share ideas, brainstorm solutions, and engage in discussions. Collaboration enhances critical thinking and communication skills.
7. Provide Scaffolding
Offer guidance and support as needed, especially at the beginning of the inquiry process. Gradually reduce assistance as students become more comfortable with self-directed learning.
8. Use Inquiry-Based Projects
Assign projects that require students to investigate, research, and present their findings. These projects can take various forms, such as presentations, reports, debates, or multimedia creations.
9. Encourage Reflection
Incorporate reflection periods where students evaluate their progress, the strategies they’ve used, and the challenges they’ve encountered. This helps them develop metacognitive skills and improve their learning approach.
10. Leverage Technology
Use technology tools to facilitate research, collaboration, and presentation. Online databases, research platforms, and communication tools can enhance the inquiry process.
11. Promote Questioning Skills
Teach students how to formulate effective questions. Encourage them to ask both lower-level and higher-order questions to guide their investigations.
12. Provide Flexibility in Assessment
Rethink traditional assessment methods. Consider using rubrics, peer evaluations, self-assessments, and portfolios that emphasize the learning process and growth rather than just final outcomes.
13. Incorporate Fieldwork and Experiments
Engage students in hands-on experiences, field trips, and experiments to apply theoretical concepts in practical settings. These experiences deepen their understanding and appreciation for the subject matter.
14. Model Inquiry
Demonstrate the inquiry process by engaging in discussions, conducting research, and asking questions yourself. Your enthusiasm for learning will inspire students to do the same.
15. Adapt to Individual Learning Styles
Recognize that students have different learning styles and paces. Provide opportunities for independent work, group activities, and one-on-one guidance to accommodate diverse needs.
16. Create a Safe Learning Environment
Foster a classroom atmosphere where students feel comfortable asking questions, taking risks, and expressing their thoughts without fear of judgment.
17. Share Success Stories
Share examples of previous students’ successful inquiries to inspire and motivate current learners. This can demonstrate the potential outcomes of the inquiry process.
Remember that the implementation of IBL may require adjustments and experimentation. It’s important to be flexible, responsive to student needs, and open to refining your approach based on the feedback and outcomes you observe.
Overcoming Challenges in Inquiry-Based Learning
Overcoming challenges in Inquiry-Based Learning (IBL) necessitates strategic planning and implementation. Here are some ways to address common obstacles:
1. Time Constraints
IBL requires more time than traditional teaching methods. To manage this, plan ahead, clearly establish learning objectives, and ensure the inquiry questions are concise yet thought-provoking. Incorporate time-saving tools like online collaboration platforms to enhance efficiency.
2. Lack of Resources
Lack of appropriate resources can inhibit IBL. Teachers can overcome this by using open-source resources available online, partnering with local organizations for field trips, or engaging industry professionals to provide real-world insights.
3. Student Resistance
Students accustomed to traditional learning methods may resist IBL. Gradual introduction of IBL, along with continuous guidance and encouragement can alleviate this issue.
4. Assessment Difficulties
Assessing IBL activities can be challenging due to their open-ended nature. Rubrics designed specifically for IBL, focusing on critical thinking, collaboration, and problem-solving skills, can be used to evaluate student performance effectively.
5. Teacher Preparation
Educators may find it difficult to shift from direct instruction to a facilitator role. Professional development, collaborative planning, and peer support can equip teachers with the necessary skills for implementing IBL.
While challenges exist, they can be overcome with strategic planning, resourcefulness, gradual implementation, and an appropriate assessment approach. In the end, the benefits of IBL — enhancing students’ critical thinking, problem-solving skills, and fostering a love for learning — far outweigh the challenges.
Inquiry-Based Learning serves as a powerful tool in the educational landscape, fostering curiosity, encouraging critical thinking, and nurturing lifelong learning habits in students. By shifting the focus from mere information delivery to active exploration, this approach motivates learners to take ownership of their education, leading to a deeper understanding and appreciation of the subject matter. Remember, the goal is not just to teach, but to inspire a thirst for knowledge that transcends the confines of the classroom.
You might also like:
- Goal Setting for Effective Learning: 12 Top Benefits
- Adaptive Learning: 7 Remarkable Benefits of Personalized Learning in the Digital Age
Steps for Inquiry and Problem-Solving
Although problem-solving and inquiry are technically different, they require many of the same skills. At a minimum, students need to recognize the language that they will need and the processes involved. Critical thinking skills are also crucial for successful inquiry projects. Teachers can model some critical thinking skills, such as
- analyzing arguments, claims, or evidence
- making inferences using inductive or deductive reasoning
- judging or evaluating
- making decisions or solving problems
- asking and answering questions for clarification
- defining terms
- identifying assumptions
- interpreting and explaining
- reasoning verbally
- seeing both sides of an issue (Lai, 2011, pp. 9-10)
Critical thinking skills do not necessarily come naturally with second language learning, and they are culturally situated, so students need to learn and practice them before, during, and after each step in the process. According to Molnar, Boninger, and Fogarty (2011), an environment that “encourages students to ask questions, to think about their thought processes, and thus to develop habits of mind that enable them to transfer the critical thinking skills they learn in class to other, unrelated, situations” is where critical thinking is cultivated (p. i).
Although sources describe the steps in the inquiry process differently, most sources include the same five basic steps of establishing orientation , conceptualization , investigation , conclusion , and discussion (Pedaste, et al., 2015). These steps are addressed to the learner and include:
- What are you interested in? Ask a question that has meaning, define the problem, and figure out what you need to do to answer it.
- Investigate by researching. Plan, gather resources and information, and record what you have found.
- Create new ideas, thoughts, and directions for action. Make sense of the information you have gathered by summarizing, synthesizing, and interpreting.
- Discuss with others. Interaction can shed new light on the question, the investigation, and the process. Share what you have learned and then use the feedback to return to the process.
- Reflect on the inquiry process. Did the process lead to unexpected conclusions? Is there something else that needs researching? Has the problem been solved?
For younger or less proficient learners, Freeman and Freeman (1998) present six steps that follow these same basic guidelines. They call this the “Wonderfilled Way of Learning,” and the steps are addressed to the teacher:
- Ask the students: What do we know about ______ ?
- Ask the students: What do we wonder about _____ ?
- Ask the students: How can we find out about _____ ?
- With the students, work out a plan of action, and, at the same time, work school district curriculum requirements into the unit.
- Plan an event to celebrate what you have learned together.
- Learning is continuous. From any unit, more topics and questions come up. Begin the cycle again. (pp. 138–139)
Regardless of which set of guidelines you and your students follow, inquiry projects can be used to support language and content learning.
In the chapter’s opening scenario, Ms. Petrie guides the students through learning experiences. She has planned that throughout the project, they will not only learn techniques for inquiry such as planning, brainstorming, reflecting, and evaluating, but through their interactions, they will also acquire a variety of language content and structures. These activities facilitate many of the CALL principles—for example, students have many opportunities for language input and output, they have many choices (structure/autonomy), they are motivated to learn because they are answering meaningful questions (authenticity and connection), and they interact with peers and community members (social interaction and feedback).

Share This Book
Feedback/errata.
Comments are closed.
- Increase Font Size

IMAGES
VIDEO
COMMENTS
Problem-Based-Learning can be classified as guided inquiry where the teacher-presented question is an unsolved, real-world problem. For example, in a Middle Eastern Studies course, the main problem posed by the instructor could be "Propose a solution to the Israeli-Palestinian conflict.". This question will motivate the study of the ...
Inquiry-based learning is an education approach that focuses on investigation and problem-solving. Inquiry-based learning is different from traditional approaches because it reverses the order of learning. Instead of presenting information, or 'the answer', up-front, teachers start with a range of scenarios, questions and problems for ...
Often it is viewed as an approach to solving problems and involves the application of several problem solving skills (Pedaste & Sarapuu, 2006). Inquiry-based learning emphasizes active participation and learner's responsibility for discovering knowledge that is new to the learner (de Jong & van Joolingen, 1998).
Balancing disciplinary knowledge and practical reasoning in problem solving is needed for meaningful learning. In STEM problem solving, science subject matter with associated practices often appears distant to learners due to its abstract nature. Consequently, learners experience difficulties making meaningful connections between science and their daily experiences. Applying Dewey's idea of ...
Inquiry-based learning can be used to help students develop critical thinking skills and make meaningful connections that will serve them in their academic and professional lives. Through inquiry-based learning, teachers can help students explore their own curiosities and develop their problem-solving abilities.
Facilitate students to apply disciplinary knowledge, critical thinking and problem-solving skills in safe, real-life contexts. Case, scenario, problem and inquiry-based learning are active learning strategies suitable for a face-to-face, online or hybrid environment. These approaches require students to apply their disciplinary knowledge, critical thinking and problem-solving skills in a safe ...
What is Inquiry-Based Learning. Humans have been practicing various forms of inquiry-based learning for centuries, including great thinkers like Einstein and Socrates. It happens when learners are curious and engaged in solving an authentic, meaningful problem. That's the basic gist of it: asking intriguing questions and searching for useful ...
Inquiry-based learning (also spelled as enquiry-based learning in British English) is a form of active learning that starts by posing questions, problems or scenarios. It contrasts with traditional education, which generally relies on the teacher presenting facts and their knowledge about the subject.Inquiry-based learning is often assisted by a facilitator rather than a lecturer.
Cooperative learning, where students work in small groups on problem-based tasks, is one approach to learning that underpins inquiry. While meta-analyses consistently document the academic and social benefits that students derive from working cooperatively together (Johnson et al., 2014), successful group work is very dependent on how the groups are structured and the types of tasks they ...
Problem-based learning (PBL) and inquiry learning are among many innovative approaches to education. They are often regarded as education's response to the rapidly changing demands of society and work. Such instructional and learning approaches situate learning in problem solving or scientific investigation of complex phenomena or problems ...
Benefits of Inquiry-Based Learning Enhanced Critical Thinking and Problem-Solving Skills. One of the most significant benefits of IBL is its capacity to enhance critical thinking and problem-solving skills. By engaging students in the process of questioning, exploring, and synthesizing information, IBL encourages a deeper level of cognitive ...
Its greatest benefit is that it requires the use of higher-order thinking skills such as problem solving to reach conclusions. There are five phases to an inquiry based learning approach. The 5 phases are: Orientation - discovering the problem. Conceptualization - finding a research question. Investigation - collecting evidence and ...
Inquiry in Problem Solving. Inquiry, according to Dewey (), involves the direct control of unknown situations to change them into a coherent and unified one.Inquiry usually encompasses two interrelated activities—(1) thinking about ideas related to conceptual subject-matter and (2) engaging in activities involving our senses or using specific observational techniques.
Inquiry-based learning helps students develop problem-solving skills. When they are given the opportunity to explore real-world problems, they are forced to think outside the box and come up with their own solutions. This is an important skill that will help them in their future careers. 3. Encourages creativity.
3. Problem-based inquiry. Ideal for problems that address real-world issues in areas like math and engineering, this approach to inquiry-based learning makes it easy for students to use what they've already learned to find answers to questions that require strong problem-solving skills. 4. Guided Inquiry
Developed in the 1960s, many teachers see inquiry-based learning as a new pedagogy -- meaning they have questions about how to use it and if it's worthwhile. Like problem-based learning, proponents state that letting students investigate solutions to open questions has a range of advantages.But the pedagogy must be shaped by research-backed approaches to reap these advantages.
Adopting an inquiry-based learning (IBL) approach in my classroom has been the most meaningful change I have made in my teaching. The benefit of increased student agency in learning, the authentic connections we make to the world around us, and the 21st-century skills IBL nurtures are great reasons to explore how inquiry can enhance what you are doing in your classroom.
The What. Inquiry and problem solving refer to an array of learner-centered processes that facilitate deep engagement with a question or problem and strategies to develop subsequent solutions and explanations. With any approach to inquiry and problem-solving, students follow a series of phases or specific discipline-based practices to take them ...
Inquiry-based learning (IBL) is a learner-centred approach which starts with an essential question. Learners investigate the topic to find answers to the question, developing language and skills throughout the inquiry. ... empathy and problem-solving skills. IBL allows students and teachers space for these skills. Read more about Specific ...
Key Takeaways: (1) Teaching math using inquiry-based learning is a powerful way to reinforce the skill of problem-solving for students. (2) Applying inquiry-based learning in math doesn't have to be a "one or the other" approach. Rather, you can combine elements of traditional math teaching and incorporate tenets of inquiry along the way ...
Inquiry-based learning is a student-centered teaching method that encourages students to ask questions and investigate real-world problems. It often enables students to connect what they are learning in school to the real world. The heart of inquiry-based learning is that students identify a question, problem, or topic that is rooted in the real world; they investigate the issue by using their ...
Inquiry-Based Learning (IBL) is an innovative approach to education that puts students' questions, ideas, and analyses front and center. ... Inquiry-based learning simulates real-world problem-solving scenarios, better preparing students for the challenges they will face in their personal and professional lives. The skills developed through IBL ...
Learning is continuous. From any unit, more topics and questions come up. Begin the cycle again. (pp. 138-139) Regardless of which set of guidelines you and your students follow, inquiry projects can be used to support language and content learning. In the chapter's opening scenario, Ms. Petrie guides the students through learning experiences.
Continuous learning not only improves decision-making but also contributes to personal and professional growth. Add your perspective Help others by sharing more (125 characters min.) Cancel
Enjoy the "Creative Problem-Solving" activity calendar and find meaningful ways to integrate media in creative and developmentally-appropriate ways. NOTE: The Support Materials are also available in Spanish.