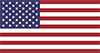
Official websites use .gov A .gov website belongs to an official government organization in the United States.
Secure .gov websites use HTTPS A lock ( ) or https:// means you've safely connected to the .gov website. Share sensitive information only on official, secure websites.
- Animal Health and Welfare

Wildlife Research
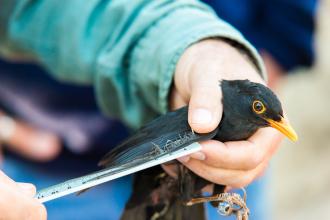
Wildlife research encompasses the study of ecology, management, and conservation involving wild mammals, fishes, amphibians, reptiles, birds, and invertebrates. When conducting this type of research, it's important for scientists to understand how it impacts wild animals, their environment, and their welfare. Use the resources and guidelines below to learn how the 3Rs (replacement, reduction and refinement of animal use) can be incorporated into wildlife research and how to conduct various procedures and techniques while considering animal welfare. From an Institutional Animal Care and Use Committee (IACUC) standpoint, it's key to understand the IACUC's role in oversight of wildlife research as well as how to review wildlife study protocols. Find information below that addresses these topics.
Laws and Regulations
Research with free-living wild animals in their natural habitat and the animal welfare act.
USDA's Animal and Plant Health Inspection Service (APHIS) provides guidance on the Animal Welfare Act's definition of "field study".
Frequently Asked Questions: Public Health Service (PHS) Policy on Humane Care and Use of Laboratory Animals
NIH's Office of Laboratory Animal Welfare (OLAW) answers questions regarding wildlife research and field studies under the PHS Policy.
Animal Welfare Challenges in Research and Education on Wildlife
This 2022 workshop held by the Institute for Laboratory Animal Research (ILAR) discusses laws and permits associated with wildlife research as well as restraint and handling of animals, transition of wild animals to captive settings, and other topics.
3Rs Alternatives in Wildlife Research
The results below are from literature searches on using the 3Rs in wildlife research and evaluating wildlife research protocols. If you want to find literature on these topics for a certain species, you can build upon these search strings by including animal keywords in the search string. To learn more about building and editing search strings, visit AWIC's alternatives literature searching page or, if you need help finding information, contact us .
NAL's Collections
Towards more compassionate wildlife research through the 3rs principles: moving…, animal welfare from mouse to moose—implementing the principles of the 3rs in wi…, a unique application to the iacuc for studies of wild animals in or from natura…, does the animal welfare act apply to free-ranging animals, a review of non-invasive sampling in wildlife disease and health research: what…, institutional animal care and use committee considerations for the use of wildl…, the ethics of wildlife research: a nine r theory., incorporation of the principles of the three rs in wildlife research.
The National Centre for the Replacement, Refinement & Reduction of Animals in Research (NC3Rs) provides examples of how the 3Rs: replacement (e.g. computer modeling), reduction (e.g. sharing data) and refinement (e.g. non-invasive sample collection) can be used in studies involving wild animals.
3Rs Principles in Wildlife Research
Dr. Miriam Zemanova, ecologist and wildlife geneticist, uses her site to share peer-reviewed studies that have implemented non-lethal or non-invasive methods that could be used as guidance for applying the 3Rs in wildlife research.
Guidelines for Using Wildlife Species in Research and Testing
2016 guidelines of the american society of mammologists for the use of wild mammals in research and education.
Journal of Mammalogy; academic.oup.com.
Information on current professional techniques and regulations involving the use of mammals in research and teaching including details on capturing, marking, housing, and humanely killing wild mammals.
Guidelines for the Use of Fishes in Research
American Fisheries Society.
These 2014 guidelines provide general recommendations on field and laboratory activities, such as sampling, holding, and handling fishes; information on regulations and permits; and advice concerning ethical questions, such as perceptions of pain or discomfort that may be experienced by experimental subjects.
Guidelines for the Use of Live Amphibians and Reptiles in Field and Laboratory Research [pdf, 42 pages]
American Society of Ichthyologists and Herpetologists.
These 2004 guidelines address various groups of reptiles and amphibians and provide guidance on techniques that are known to be humane and effective in field research.
Guidelines to the Use of Wild Birds in Research
The Ornithological Council.
These 2022 guidelines address techniques relevant to birds and are formulated with consideration to animal welfare and research needs.
Guidelines on the Care and Use of Wildlife [pdf, 70 pages]
Canadian Council on Animal Care (CCAC).
These 2023 recommendations are for various procedures such as capture, restraint, handling, translocation, holding, and euthanasia of wild animals in research, management, teaching, and testing.
Page Content Curated By
Thank you for visiting nature.com. You are using a browser version with limited support for CSS. To obtain the best experience, we recommend you use a more up to date browser (or turn off compatibility mode in Internet Explorer). In the meantime, to ensure continued support, we are displaying the site without styles and JavaScript.
- View all journals
- Explore content
- About the journal
- Publish with us
- Sign up for alerts
- Brief Communication
- Published: 02 November 2014
Rovers minimize human disturbance in research on wild animals
- Yvon Le Maho 1 , 2 , 3 ,
- Jason D Whittington 1 , 2 , 3 , 4 , 5 ,
- Nicolas Hanuise 1 , 2 ,
- Louise Pereira 1 , 2 ,
- Matthieu Boureau 1 , 2 ,
- Mathieu Brucker 1 , 2 ,
- Nicolas Chatelain 1 , 2 ,
- Julien Courtecuisse 1 , 2 ,
- Francis Crenner 1 , 2 ,
- Benjamin Friess 1 , 2 ,
- Edith Grosbellet 1 , 2 ,
- Laëtitia Kernaléguen 1 , 2 ,
- Frédérique Olivier 6 , 7 ,
- Claire Saraux 1 , 2 , 8 ,
- Nathanaël Vetter 1 , 2 ,
- Vincent A Viblanc 1 , 2 , 9 ,
- Bernard Thierry 1 , 2 ,
- Pascale Tremblay 3 ,
- René Groscolas 1 , 2 &
- Céline Le Bohec 3 nAff10
Nature Methods volume 11 , pages 1242–1244 ( 2014 ) Cite this article
5671 Accesses
35 Citations
521 Altmetric
Metrics details
- Behavioural methods
- Data acquisition
- Hardware and infrastructure
Investigating wild animals while minimizing human disturbance remains an important methodological challenge. When approached by a remote-operated vehicle (rover) which can be equipped to make radio-frequency identifications, wild penguins had significantly lower and shorter stress responses (determined by heart rate and behavior) than when approached by humans. Upon immobilization, the rover—unlike humans—did not disorganize colony structure, and stress rapidly ceased. Thus, rovers can reduce human disturbance of wild animals and the resulting scientific bias.
This is a preview of subscription content, access via your institution
Access options
Subscribe to this journal
Receive 12 print issues and online access
251,40 € per year
only 20,95 € per issue
Buy this article
- Purchase on Springer Link
- Instant access to full article PDF
Prices may be subject to local taxes which are calculated during checkout
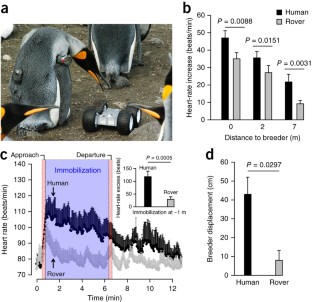
Similar content being viewed by others
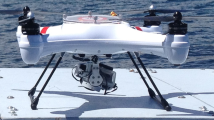
Behaviour reactions of bottlenose dolphins (Tursiops truncatus) to multirotor Unmanned Aerial Vehicles (UAVs)
Ticiana Fettermann, Lorenzo Fiori, … Barbara Bollard
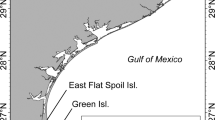
Drone Surveys Do Not Increase Colony-wide Flight Behaviour at Waterbird Nesting Sites, But Sensitivity Varies Among Species
Jared R. Barr, M. Clay Green, … Thomas B. Hardy
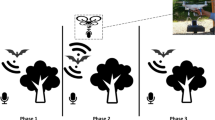
Fewer bat passes are detected during small, commercial drone flights
Gabrielle Ednie, David M. Bird & Kyle H. Elliott
Ellenberg, U., Mattern, T., Seddon, P.J. & Jorquera, G.L. Biol. Conserv. 133 , 95–106 (2006).
Article Google Scholar
French, S.S., DeNardo, D.F., Greives, T.J., Strand, C.R. & Demas, G.E. Horm. Behav. 58 , 792–799 (2010).
Article CAS Google Scholar
Viblanc, V.A., Smith, A.D., Gineste, B. & Groscolas, R. BMC Ecol. 12 , 10 (2012).
Le Maho, Y. et al. Am. J. Physiol. 263 , R775–R781 (1992).
CAS PubMed Google Scholar
Jones, T.T. et al. Meth. Ecol. Evol. 4 , 1178–1186 (2013).
Culik, B., Wilson, R. & Bannasch, R. Mar. Ecol. Prog. Ser. 98 , 209–214 (1993).
Saraux, C. et al. Nature 469 , 203–206 (2011).
Le Maho, Y. et al. C. R. Biol. 334 , 378–384 (2011).
Gendner, J.P., Gauthier-Clerc, M., Le Bohec, C., Descamps, S. & Le Maho, Y. J. Field Ornithol. 76 , 138–142 (2005).
Nicolaus, M., Bouwman, K. & Dingemanse, N. Ardea 96 , 286–292 (2008).
O'Shea, T.J., Ellison, L.E. & Stanley, T.R. J. Mamm. 92 , 433–443 (2011).
Caswell, J.H., Alisauskas, R.T. & Leafloor, J.O. J. Wildl. Mgmt. 76 , 1456–1461 (2012).
Chiaradia, A.F. & Kerry, K.R. Mar. Ornithol. 27 , 13–20 (1999).
Google Scholar
Johnston, P., Bérubé, F. & Bergeron, N.E. J. Fish Biol. 74 , 1651–1661 (2009).
Grémillet, D., Puech, W., Garçon, V., Boulinier, T. & Le Maho, Y. Open Ecol. J. 2 , 49–57 (2012).
Viblanc, V.A., Valette, V., Kauffmann, M., Malosse, N. & Groscolas, R. Behav. Ecol. 23 , 1178–1185 (2012).
Nimon, A.J., Schroter, R.C. & Oxenham, R.K.C. Physiol. Behav. 60 , 1019–1022 (1996).
Nephew, B.C., Kahn, S.A. & Romero, L.M. Gen. Comp. Endocrinol. 133 , 173–180 (2003).
Cyr, N.E., Dickens, M.J. & Romero, L.M. Physiol. Biochem. Zool. 82 , 332–344 (2009).
Groscolas, R., Viera, V., Guerin, N., Handrich, Y. & Côté, S.D. J. Exp. Biol. 213 , 153–160 (2010).
Viera, V.M., Viblanc, V.A., Filippi-Codaccioni, O., Côté, S.D. & Groscolas, R. Anim. Behav. 82 , 69–76 (2011).
Lengagne, T., Jouventin, P. & Aubin, T. Behaviour 136 , 833–846 (1999).
Jouventin, P. La Terre et La Vie 25 , 510–586 (1971).
Gutzwiller, K.J. & Marcum, H.A. J. Wildl. Mgmt. 61 , 935–947 (1997).
Peltier, M.R., Wilcox, C.J. & Sharp, D.C. J. Anim. Sci. 76 , 847–849 (1998).
Download references
Acknowledgements
This study was undertaken within Program 137 of the Institut Polaire Français Paul-Emile Victor (IPEV), with support from the French National Research Agency (A.N.R.) “PICASO” grant. We are very grateful to the mine-clearing services of the French Ministry of the Interior for the gift of a mine-clearing rover for preliminary tests. All technical developments were supported by Total Corporate Foundation. M. Brucker, N.C., J.C., F.C., B.F., R.G. and Y.L.M. were supported by the CNRS. C.L.B. and P.T. were supported by Centre Scientifique de Monaco (CSM). V.A.V. was supported by the AXA Research Fund. Y.L.M., C.L.B., P.T. and J.D.W. collaborated under the framework of the Laboratoire International Associé 647 BioSensib between the Institut Pluridisciplinaire Hubert Curien (CNRS and University of Strasbourg) and the CSM.
Author information
Céline Le Bohec
Present address: Present addresses: Institut Pluridisciplinaire Hubert Curien, Université de Strasbourg, Laboratoire International Associé LIA-647 BioSensib (CSM-CNRS-UdS), Strasbourg, France, and CNRS, Unité Mixte de Recherche 7178, LIA-647 BioSensib, Strasbourg, France.,
Authors and Affiliations
Institut Pluridisciplinaire Hubert Curien, Université de Strasbourg, Laboratoire International Associé LIA-647 BioSensib (CSM-CNRS-UdS), Strasbourg, France
Yvon Le Maho, Jason D Whittington, Nicolas Hanuise, Louise Pereira, Matthieu Boureau, Mathieu Brucker, Nicolas Chatelain, Julien Courtecuisse, Francis Crenner, Benjamin Friess, Edith Grosbellet, Laëtitia Kernaléguen, Claire Saraux, Nathanaël Vetter, Vincent A Viblanc, Bernard Thierry & René Groscolas
Centre National de la Recherche Scientifique (CNRS), Unité Mixte de Recherche 7178, LIA-647 BioSensib, Strasbourg, France.,
Centre Scientifique de Monaco (CSM), LIA-647 BioSensib, Monaco.,
Yvon Le Maho, Jason D Whittington, Pascale Tremblay & Céline Le Bohec
Nordic Centre for Research on Marine Ecosystems and Resources under Climate Change, University of Oslo, Oslo, Norway
Jason D Whittington
Department of Biosciences, Centre for Ecological and Evolutionary Synthesis, University of Oslo, Oslo, Norway
John Downer Productions Ltd, Bristol, UK
Frédérique Olivier
Institute for Marine and Antarctic Studies, University of Tasmania, Hobart, Tasmania, Australia.,
Institut Français de Recherche pour l'Exploitation de la Mer, Sète, France
Claire Saraux
Centre d'Ecologie Fonctionnelle et Evolutive, CNRS, Unité Mixte de Recherche 5158, Montpellier, France
Vincent A Viblanc
You can also search for this author in PubMed Google Scholar
Contributions
Y.L.M., team leader of the IPEV project, designed and performed the study in the field, worked on the analyses and cowrote the paper; C.L.B., project co-leader, and J.D.W. worked on the analyses and cowrote the paper; N.H. and L.P. worked on the analyses and provided useful comments; technical developments pertaining to rover conception, development and construction were performed by M. Brucker, N.C., J.C., F.C. and B.F.; M. Boureau, L.K., E.G. and N.V. performed the study in the field and provided useful comments; R.G. suggested the use of HR monitoring to test the impact of approaching rovers, proposed a field test, performed some analyses and helped in revising the paper; B.T. helped in interpreting data within an ethological framework and in revising the paper; F.O. added useful modifications to the manuscript; V.A.V. and C.S. ran some preanalyses and greatly contributed in the paper's revision; P.T. participated in a prestudy on the field and added useful modifications to the manuscript.
Corresponding authors
Correspondence to Yvon Le Maho or Céline Le Bohec .
Ethics declarations
Competing interests.
The authors declare no competing financial interests.
Integrated supplementary information
Supplementary figure 1 rover camouflaged with an emperor penguin chick model “huddling” with chicks in a crèche..
The rover was able to infiltrate the emperor penguin crèche without disturbance, thus demonstrating the possibility to use such a camouflaged rover to collect data from locations within a colony that are not accessible to a human investigator.
Supplementary information
Supplementary text and figures.
Supplementary Figure 1 (PDF 156 kb)
King penguin circulating among incubating breeders
Incubating king penguins are stationary, generally maintaining neighbors and birds in transit at a minimum distance determined by the reach of their beak and flippers. (MOV 19948 kb)
Rover circulating among incubating breeders
The reactions of incubating king penguins to the rover was similar in magnitude to their reactions to conspecifics and ceased as soon as the rover stopped moving. (MOV 30018 kb)
Rover approaching southern elephant seals on a beach
Resting southern elephant seals let the rover approach to within contact distances to their heads and also to their tails, where they are usually tagged, with no visible disturbance. (MOV 26114 kb)
Source data
Source data to fig. 1, rights and permissions.
Reprints and permissions
About this article
Cite this article.
Le Maho, Y., Whittington, J., Hanuise, N. et al. Rovers minimize human disturbance in research on wild animals. Nat Methods 11 , 1242–1244 (2014). https://doi.org/10.1038/nmeth.3173
Download citation
Received : 05 March 2014
Accepted : 30 August 2014
Published : 02 November 2014
Issue Date : December 2014
DOI : https://doi.org/10.1038/nmeth.3173
Share this article
Anyone you share the following link with will be able to read this content:
Sorry, a shareable link is not currently available for this article.
Provided by the Springer Nature SharedIt content-sharing initiative
This article is cited by
Steps toward an ethics of environmental robotics.
- Justin Donhauser
- Aimee van Wynsberghe
- Alexander Bearden
Philosophy & Technology (2021)
Machine learning enables improved runtime and precision for bio-loggers on seabirds
- Joseph Korpela
- Hirokazu Suzuki
Communications Biology (2020)
Fasting: the Switch of Life – Tagungsbericht zum 18. Internationalen Kongress der Ärztegesellschaft Heilfasten und Ernährung e. V. (ÄGHE)
- Felicia Kleimaier
- Caroline Klatte
- Daniela Koppold-Liebscher
Bundesgesundheitsblatt - Gesundheitsforschung - Gesundheitsschutz (2019)
Long lasting breeding performance differences between wild-born and released females in a reinforced North African Houbara bustard (Chlamydotis undulata undulata) population: a matter of release strategy
- Alexandre Robert
- Yves Hingrat
Biodiversity and Conservation (2019)
Closed-loop interactions between a shoal of zebrafish and a group of robotic fish in a circular corridor
- Frank Bonnet
- Alexey Gribovskiy
- Francesco Mondada
Swarm Intelligence (2018)
Quick links
- Explore articles by subject
- Guide to authors
- Editorial policies
Sign up for the Nature Briefing: Anthropocene newsletter — what matters in anthropocene research, free to your inbox weekly.

ENCYCLOPEDIC ENTRY
Wildlife conservation.
Wildlife conservation aims to protect plant and animal species as the human population encroaches on their resources.
Biology, Ecology, Conservation, Storytelling, Photography
Asian Elephant Family
Filmmakers and photographers are essential to conservation efforts. They take the photographs, such as these Asian elephants (Elephas maximus indicus), and the films that interest others in protecting wildlife.
Photograph by Nuttaya Maneekhot
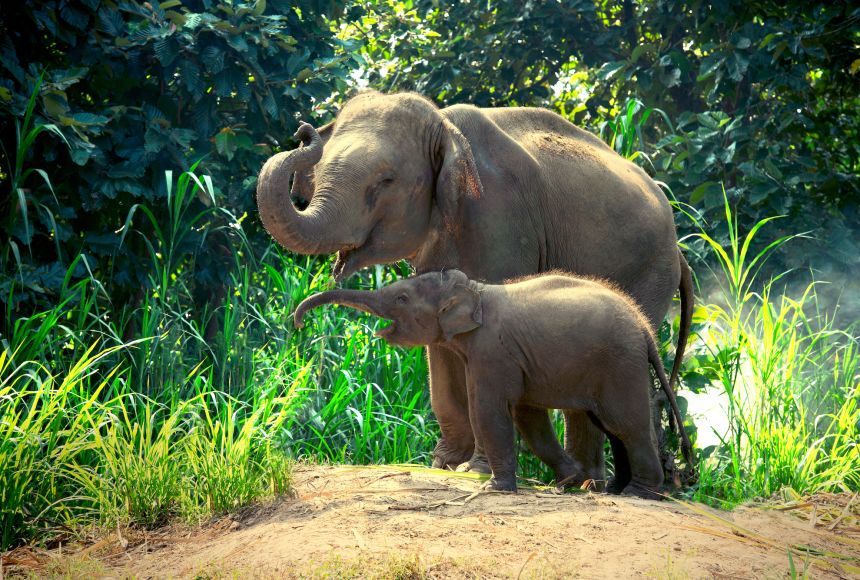
Wildlife conservation is the practice of protecting plant and animal species and their habitats . Wildlife is integral to the world’s ecosystems , providing balance and stability to nature’s processes. The goal of wildlife conservation is to ensure the survival of these species, and to educate people on living sustainably with other species. The human population has grown exponentially over the past 200 years, to more than eight billion humans as of November 2022, and it continues to rapidly grow. This means natural resources are being consumed faster than ever by the billions of people on the planet. This growth and development also endangers the habitats and existence of various types of wildlife around the world, particularly animals and plants that may be displaced for land development, or used for food or other human purposes. Other threats to wildlife include the introduction of invasive species from other parts of the world, climate change, pollution, hunting, fishing, and poaching. National and international organizations like the World Wildlife Fund, Conservation International, the Wildlife Conservation Society, the United Nations, and National Geographic, itself, work to support global animal and habitat conservation efforts on many different fronts. They work with the government to establish and protect public lands, like national parks and wildlife refuges . They help write legislation, such as the Endangered Species Act (ESA) of 1973 in the United States, to protect various species. They work with law enforcement to prosecute wildlife crimes, like wildlife trafficking and illegal hunting (poaching). They also promote biodiversity to support the growing human population while preserving existing species and habitats. National Geographic Explorers, like conservation biologists Camille Coudrat and Titus Adhola, are working to slow the extinction of global species and to protect global biodiversity and habitats. Environmental filmmakers and photographers, like Thomas P. Peschak and Joel Sartore, are essential to conservation efforts as well, documenting and bringing attention to endangered wildlife all over the world.
Media Credits
The audio, illustrations, photos, and videos are credited beneath the media asset, except for promotional images, which generally link to another page that contains the media credit. The Rights Holder for media is the person or group credited.
Production Managers
Program specialists, last updated.
April 8, 2024
User Permissions
For information on user permissions, please read our Terms of Service. If you have questions about how to cite anything on our website in your project or classroom presentation, please contact your teacher. They will best know the preferred format. When you reach out to them, you will need the page title, URL, and the date you accessed the resource.
If a media asset is downloadable, a download button appears in the corner of the media viewer. If no button appears, you cannot download or save the media.
Text on this page is printable and can be used according to our Terms of Service .
Interactives
Any interactives on this page can only be played while you are visiting our website. You cannot download interactives.
Related Resources
We can help you reset your password using the email address linked to your BioOne Complete account.

- BioOne Complete Titles
- BioOne eBook Titles
- About BioOne Digital Library
- How to Subscribe & Access
- Library Resources
- Publisher Resources
- Instructor Resources
Publisher: CSIRO Publishing
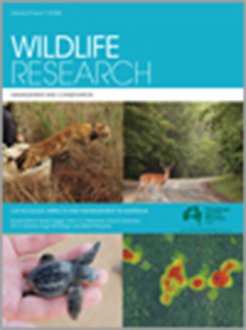
- Featured Content
- Scope & Details
- Editorial Office
- Author Guidelines
Wildlife Research represents an international forum for the publication of research and debate on the ecology, management and conservation of wild animals in natural and modified habitats. The journal combines basic research in wildlife ecology with advances in science-based management practice. Subject areas include: applied ecology; conservation biology; ecosystem management; management of over-abundant, pest and invasive species; global change and wildlife management; diseases and their impacts on wildlife populations; human dimensions of management and conservation; assessing management outcomes; and the implications of wildlife research for policy development.
Readers can expect a range of papers covering well-structured field studies, manipulative experiments, and analytical and modelling studies. All papers aim to improve the practice of wildlife management and contribute conceptual advances to our knowledge and understanding of wildlife ecology.

KEYWORDS/PHRASES
Publication title:, collection title:, publication years.
- Skip to primary navigation
- Skip to main content
Texas A&M AgriLife wildlife data included in global study
Research provides unprecedented insight into wildlife response during covid-19 lockdown.
April 17, 2024
Camera trap data collected by a Texas A&M AgriLife researcher contributed to one of the largest international studies on wildlife response to changes in human activity as a result of the COVID-19 pandemic.
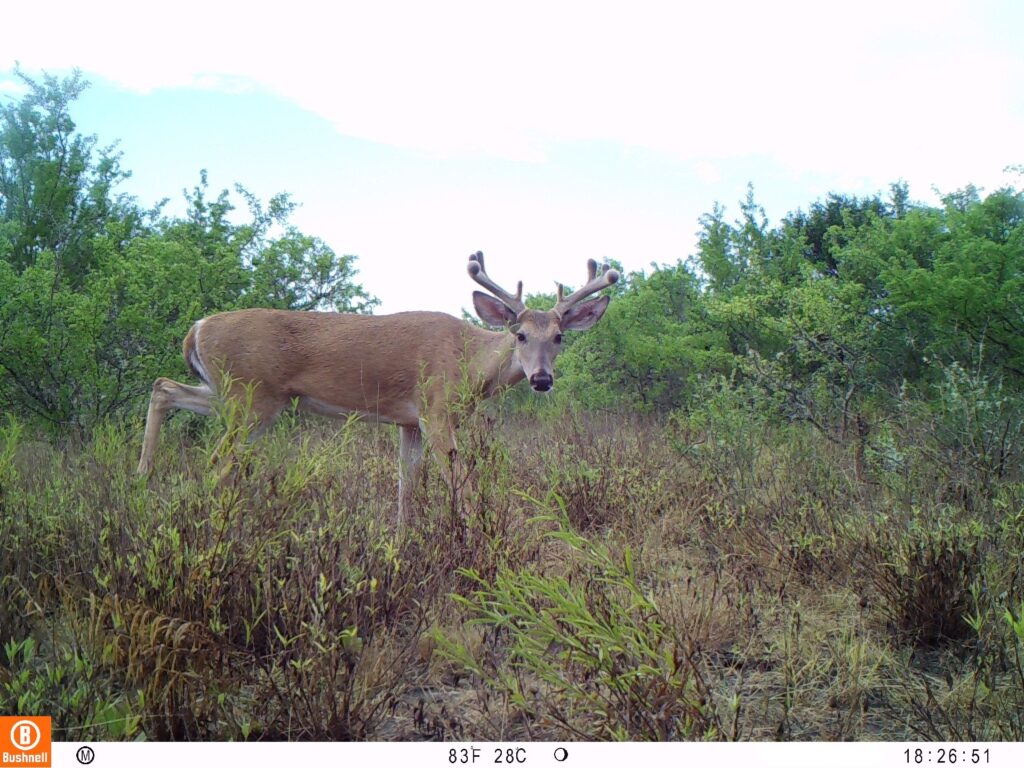
The study, published in Nature Ecology and Evolution , involved more than 220 researchers, 163 mammal species and over 5,000 camera traps worldwide. Its findings revealed that wildlife react differently to human activity, depending on where the animals live and their position in the food web.
Research born out of existing projects and partnerships
This far-reaching endeavor was made possible by Snapshot USA , a collaborative effort started in 2019 to sample mammal populations across the U.S. using camera traps, said Stephen Webb, Ph.D., Texas A&M Natural Resources Institute research assistant professor in the Texas A&M College of Agriculture and Life Sciences Department of Rangeland, Wildlife and Fisheries Management , Bryan-College Station.
“Biologists realized the importance of bringing together the vast amount of information being collected from existing camera trap projects taking place across the country,” Webb said. “By joining this effort, participating researchers agreed their camera trap data could be used by other biologists to investigate and analyze wildlife behavior.”
The researchers knew this vast data set would help establish baselines for wildlife presence, abundance and distribution, but they had no way of knowing how valuable the shared information would be following the drastic changes in human activity during the pandemic, he said.
COVID-19 ‘anthropause’ sees exceptional alteration in human activity
As activity-related restrictions were implemented across the globe in early 2020 to slow the spread of COVID-19 — a period of reduced human movement referred to by researchers as the “anthropause” — biologists saw an extraordinary opportunity for research.
“COVID-19 mobility restrictions gave researchers a unique opportunity to study how animals responded when the number of people sharing their landscape changed drastically over a relatively short period,” said Cole Burton, Ph.D., lead author of the study, associate professor of forest resources management at the University of British Columbia and Canada Research Chair in Terrestrial Mammal Conservation.
Researchers accessed data from 5,400 camera-trap locations, primarily in Europe and North America, and utilized a variety of analytical models to quantify variation in animal responses across sites based on species traits, landscape modification and other site characteristics. They also incorporated the level of documented change in human activity prior to, during and after pandemic travel restrictions.
Webb said this statistical combination of results from multiple studies is referred to as a meta-analysis.
Findings differ from popular anecdotes on animal response to lockdown
While popular news stories during pandemic lockdowns promoted the narrative of wildlife roaming free and nature “reclaiming” urban development, the study’s findings indicate wildlife response to human presence or absence is far more nuanced.
“We did not see an overall pattern of ‘wildlife running free’ while humans sheltered in place,” Burton said. “Rather, we saw great variation in activity patterns of people and wildlife, with the most striking trends being that animal responses depended on landscape conditions and their position in the food chain.”
The study showed larger herbivores like deer or moose tended to become more active when humans were present, while carnivores like wolves or wolverines were less active.
Animals such as deer or raccoons in urban areas that are accustomed to humans may become more active around people and access anthropomorphic resources, such as garbage or plants, at night. However, animals living farther from cities and other developed areas are more cautious of possible human encounters.
Webb said these findings make sense when one considers the baseline of human exposure an animal is accustomed to.
“If wildlife are exposed to higher levels of human activity, they’re going to behave differently because they’re habituated to humans and kind of know what to expect,” Webb said. “However, animals that are truly in the wild or more rural areas aren’t accustomed to human presence. You must consider what the baseline of human exposure is for the animal to understand how it might respond.”
Studies like this, which include such a large amount of data on diverse wildlife species located across various geographic areas, help scientists analyze the subtleties of wildlife behavior beyond broad generalizations, he said.
Separate research to investigate impact of habitat management on wildlife
Webb is also utilizing camera traps to investigate the impact of habitat management on wildlife at the Department of Rangeland, Wildlife and Fisheries Management’s La Copita Demonstration Ranch and Research Area west of Corpus Christi.
Established in 1981, the 2,726-acre ranch is planning a variety of management activities including cattle grazing, brush removal, prescribed fire, fence construction and more.
Camara traps located throughout the property will allow Webb and other Texas A&M AgriLife researchers to quantify how these activities affect the presence and abundance of a variety of wildlife.
While Webb will conduct his own independent research using the camera traps, the data will also be available to other researchers for additional studies.
“When researchers collaborate and share information like this, it’s a powerful tool to better understand how our actions and habitat management techniques affect wildlife,” Webb said. “In turn, that empowers us to make better management decisions to advance wildlife conservation.”
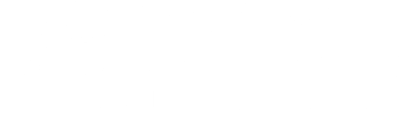
- Resources for Press & Media
- Story Suggestion
Watch CBS News
Scientists trying to protect wildlife from extinction as climate change raises risk to species around the globe
By Grace Manthey , David Schechter , Tracy J. Wholf , Chance Horner , Haley Rush , Alexis Guerrero
Updated on: April 22, 2024 / 1:02 PM EDT / CBS News
From clean air and water to healthy soil and medicines, our survival and prosperity rely heavily on the rich diversity of plants and animals that make up our world. A 2019 U.N. report found that around 1 million plant and animal species could be threatened with extinction around the globe. But new research finds climate change could drive up to 6 million different species to extinction over the next 50 years, including in communities across the United States.
CBS News and Stations explored how a warming planet and unchecked development are leading to significant population declines in species, why that's bad for humanity, and the heroic lengths some scientists will go to protect life on Earth.
For some species, it's already too late. For others, there are innovative ways to help rehabilitate a species decimated by climate change. Scientists are using a range of tools to protect species in creative and unique ways and to anticipate future changes.
In Bisbee, Arizona, one scientist who has spent his career studying evolutionary biology and ecology is tracking a 3-million-year-old lizard population dying at one of the fastest rates ever recorded.
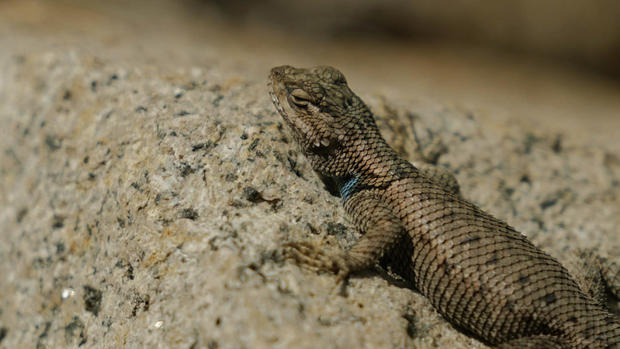
Another group is trying to save the Puerto Rican parrot , one of the most critically endangered birds in the world, as more destructive hurricanes jeopardize the parrot's ecosystem.
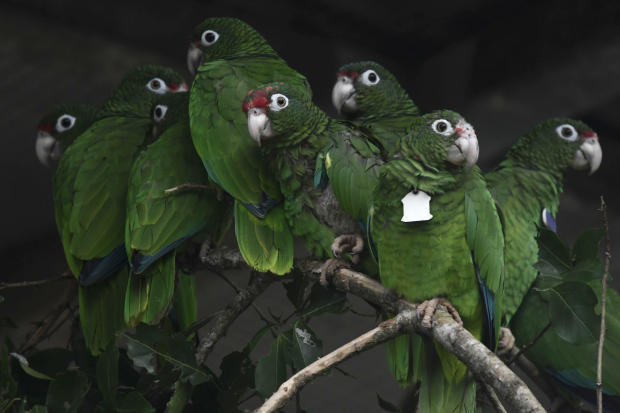
See more reporting on how groups are trying to save certain species
More stories will be added above as they are published.
Endangered species by the numbers
The U.S. Endangered Species Act was established in 1973 and provides federal protection for wildlife in danger of becoming extinct.
The main agency responsible for carrying out the act, the U.S. Fish and Wildlife Service, lists nearly 1,700 species endangered or threatened as of April 1. Nearly 1,400 species on the list have active recovery plans. New species are added every year.
A 2019 study estimated the Endangered Species Act had prevented the extinction of nearly 300 species since its passage.
But not every species in danger of extinction gets listed. A 2016 study found that typically, species waited 12 years to receive protection, for those reviewed between 1973 and 2014. The deadlines included in the act dictate it should only take two years when initiated by a third party.
Still, nearly every county in the U.S. has at least one species in danger of disappearing from the planet.
Number of endangered or threatened species by county in the U.S.
Roll over or click on a county below to see more information about species in the area.
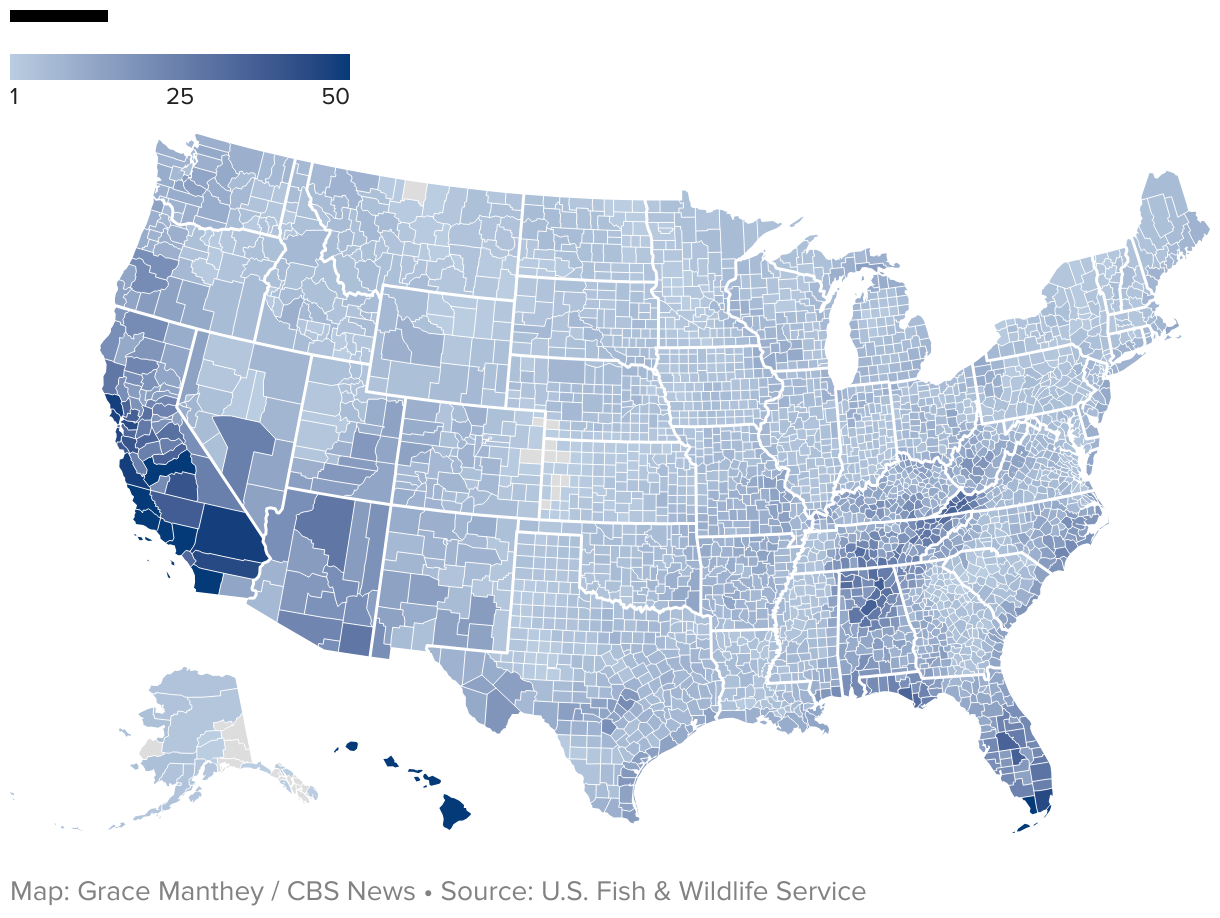
Across all U.S. states, Hawaii has the greatest number of species listed as endangered or threatened by the U.S. Fish and Wildlife Service — estimated at nearly 500 species.
Endangered or threatened species in Hawaii
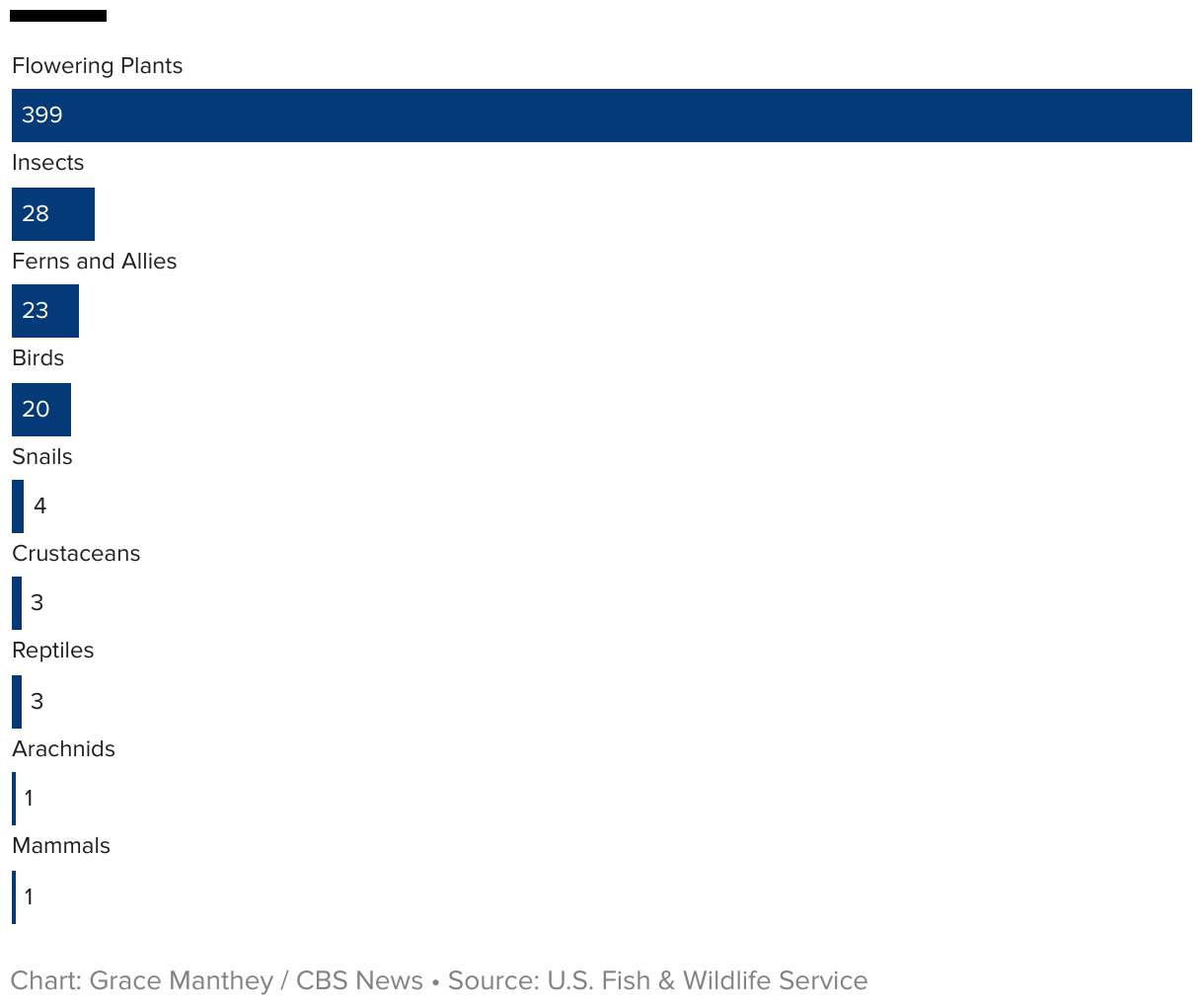
The number is driven mostly by flowering plants, including the iconic state flower, the ma'o hau hele, or native yellow hibiscus flower.
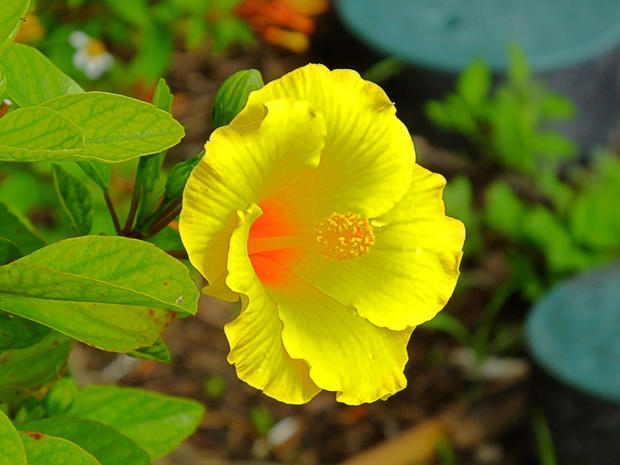
California has the second highest number listed, with nearly 300 endangered or threatened species. This includes the San Joaquin kit fox and the Lange's metalmark butterfly. Like Hawaii, the high number is driven by the roughly 170 species of flowering plants. There are higher numbers in coastal, central and southern counties.
Number of endangered threatened species by county in California
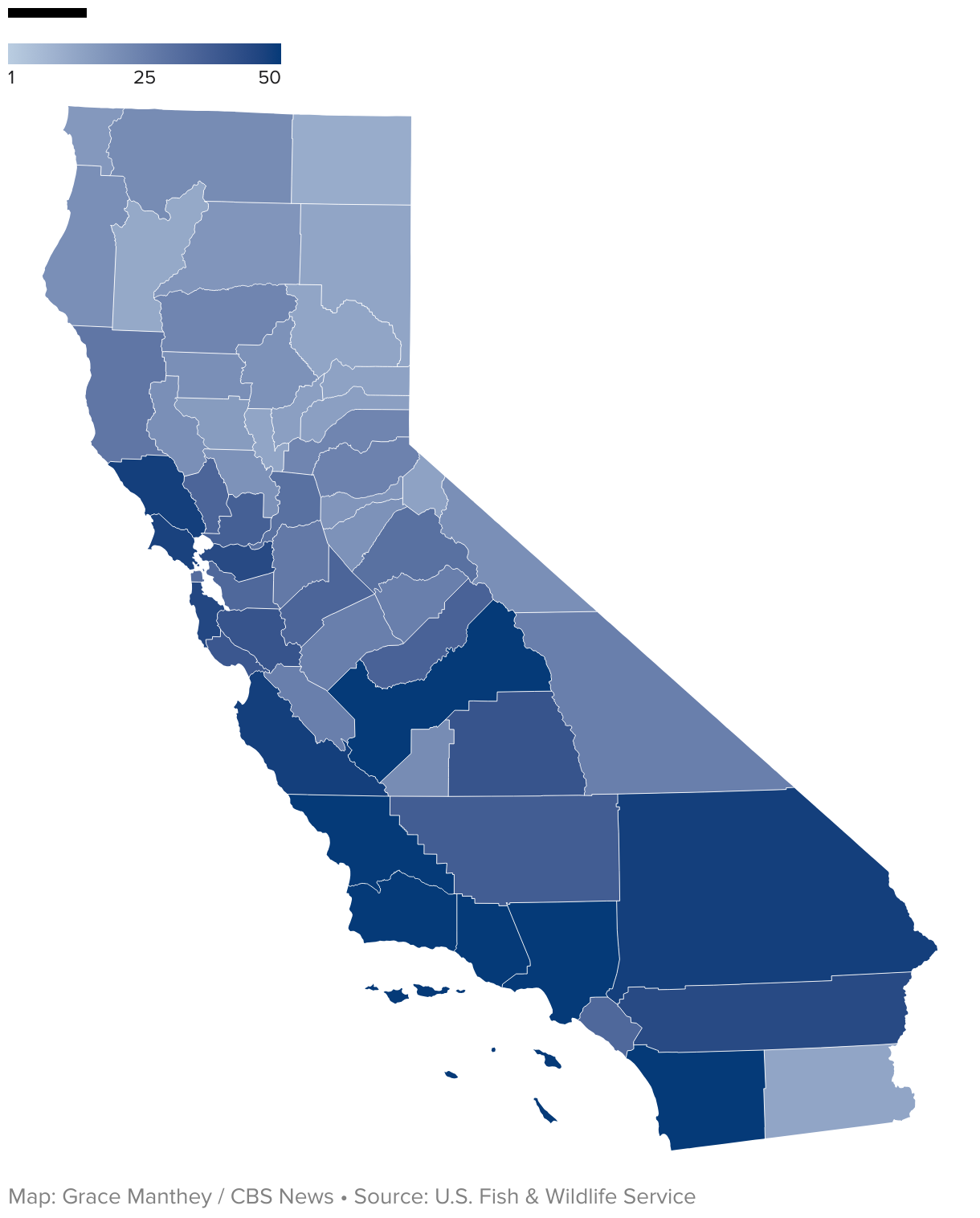
- Endangered Species Act
- Climate Change
- Endangered Species
Grace Manthey is the senior visual data journalist and data team coordinator for CBS News and Stations. She is an Emmy-winning journalist and visual storyteller with a passion for telling stories that matter.
More from CBS News
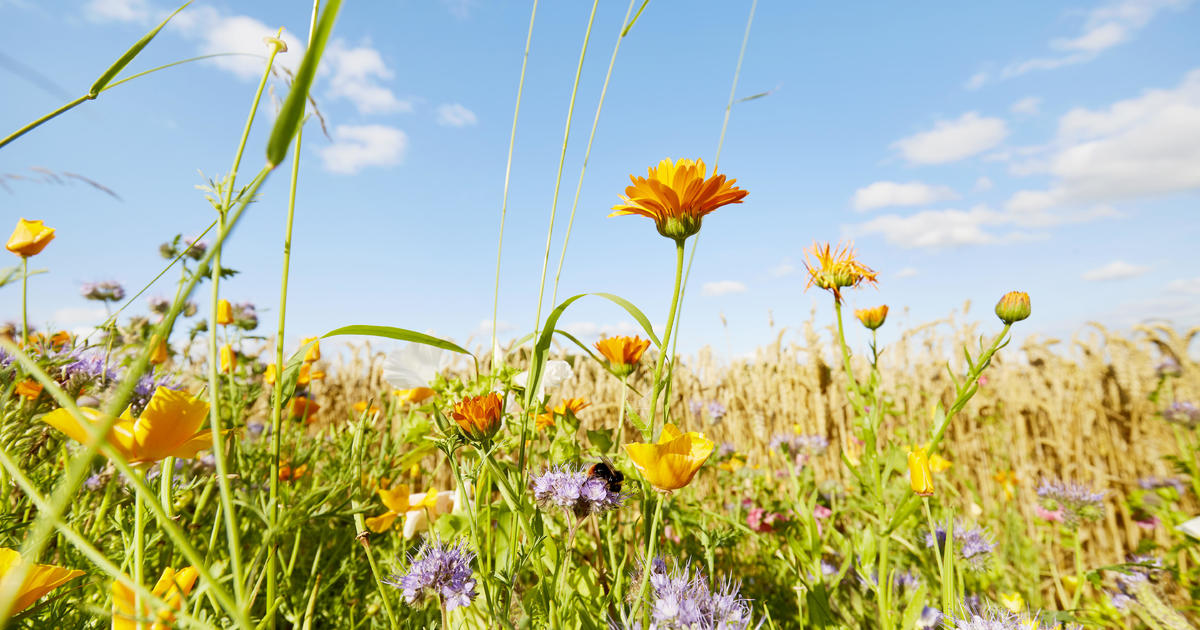
What is biodiversity?
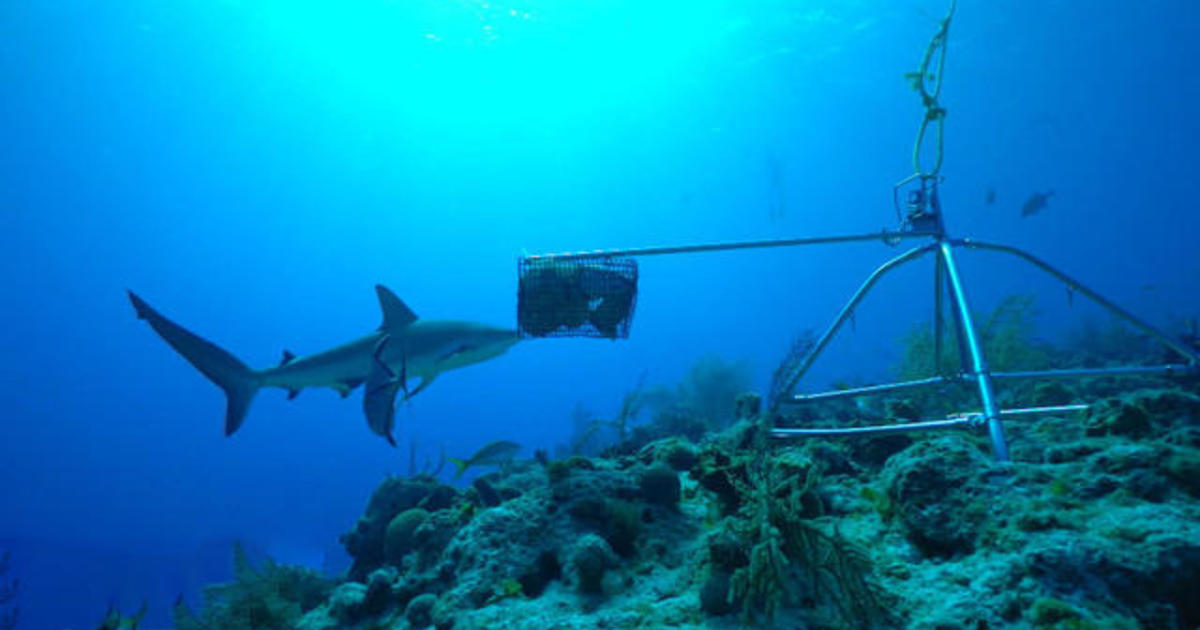
How countries are using innovative technology to preserve ocean life
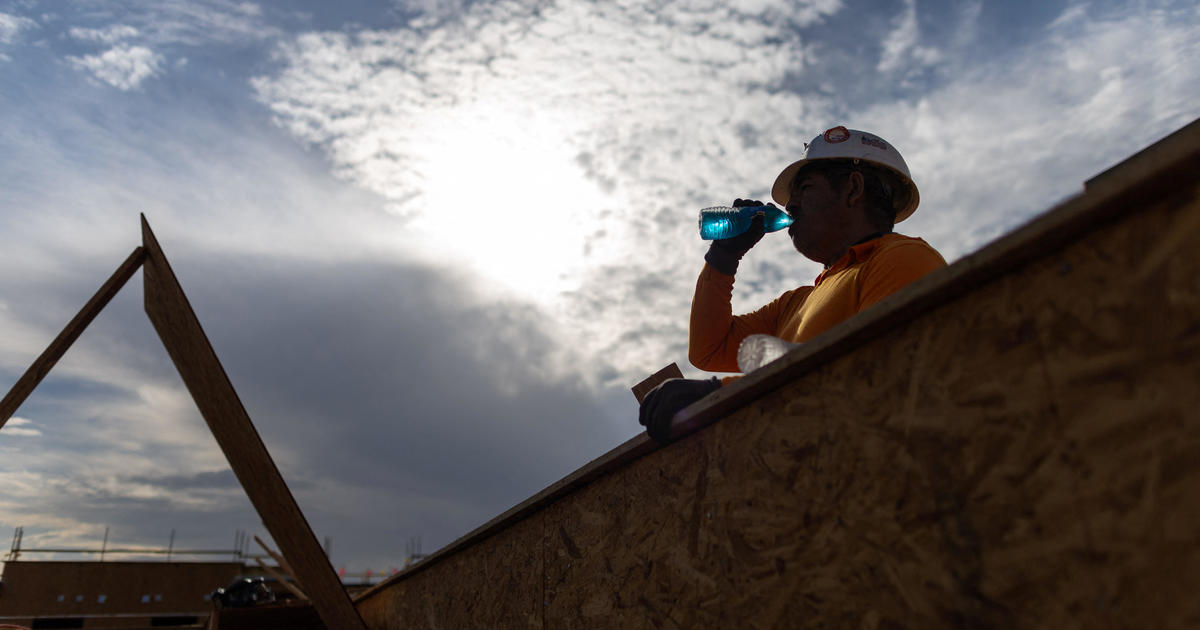
Climate change a health risk for 70% of world's workers, UN warns
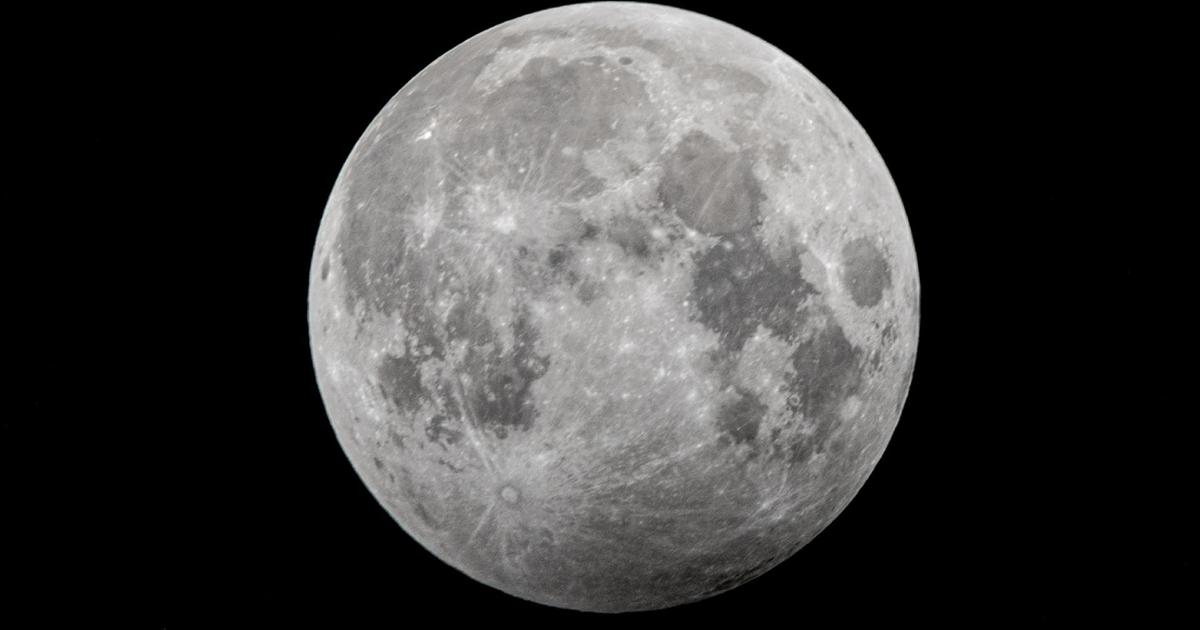
Could some species dying on Earth be saved in outer space?
share this!
April 20, 2024
This article has been reviewed according to Science X's editorial process and policies . Editors have highlighted the following attributes while ensuring the content's credibility:
fact-checked
peer-reviewed publication
trusted source

Study uncovers neural mechanisms underlying foraging behavior in freely moving animals
by Patrick Kurp, Rice University
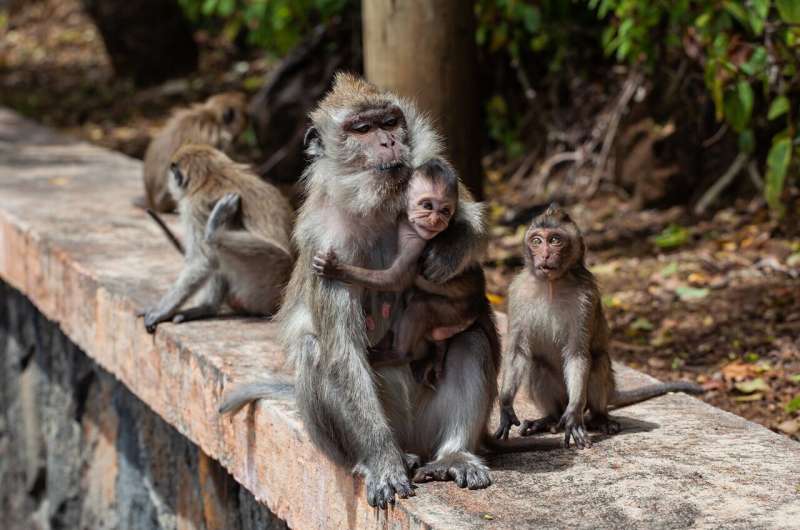
While foraging, animals including humans and monkeys are continuously making decisions about where to search for food and when to move among possible sources of sustenance.
"Foraging behavior is something we perform daily when we go to the grocery store to pick up food, and we make choices based on the degree of reward each choice provides. It's a classical problem common to every species on the planet," said Valentin Dragoi, professor of electrical and computer engineering at Rice, professor of neuroscience at Weill Cornell Medical College and scientific director of the Methodist/Rice Center for Neural Systems Restoration.
In a paper published in Nature Neuroscience , Dragoi and collaborators investigate the brain processes involved in searching for food.
"In this study, we describe the use of a new integrated wireless system for recording brain activity in the frontal areas of their brain and for oculomotor and behavioral tracking. We examine in real time how this ubiquitous task of foraging unfolds, which is something we naturally perform every day," Dragoi said.
Macaques are a genus of monkeys native to Asia, North Africa and Southern Europe (Gibraltar). They most often eat fruit, seeds and other plant-based food. "We study macaques," Dragoi said, "because foraging is a natural behavior and the macaque brain is quite similar to the human brain in terms of organization and function."
Until now, it was difficult to examine the neural basis of foraging in naturalistic environments because previous approaches relied on restrained animals performing trial-based foraging tasks. Dragoi and his research partners allowed unrestrained macaques to freely interact with reward options while wirelessly recording neural activity in their prefrontal cortex.
"Animals decided when and where to forage based on whether their predictions of reward were fulfilled or violated. The predictions were not based exclusively on a history of reward delivery, but also on the understanding that waiting longer improves the chance of reward," Dragoi said.
The results indicate that foraging strategies are based on a cortical model of reward dynamics as animals freely explore their environment.
"We learned that we can predict choices even in complex situations by simply reading out the responses of dozens of neurons in the frontal lobe. This can potentially move in the direction of prosthetic devices to influence or bias choice, even noninvasively. More fundamentally, it allows us to understand how the brain works when engaged in this natural behavior," Dragoi said.
Next, the Dragoi lab will combine foraging in a social context and record from two animals simultaneously while they cooperate to seek food as a reward. This is a daunting technical challenge but Dragoi believes he and his research partners are close to achieving such goals. This may enable a solution to the challenge of cortical implants to assist patients with brain dysfunction and enable their behavioral decisions.
The lead author of the article is Neda Shahidi, a former Ph.D. student in Dragoi's lab, currently group leader at Georg-Elias-Müller-Institute for Psychology, Georg August-Universität, Göttingen.
Journal information: Nature Neuroscience
Provided by Rice University
Explore further
Feedback to editors

Electron videography captures moving dance between proteins and lipids
10 minutes ago

New findings shed light on how bella moths use poison to attract mates
14 minutes ago

AI tool creates 'synthetic' images of cells for enhanced microscopy analysis
17 minutes ago

Announcing the birth of QUIONE, a unique analog quantum processor
34 minutes ago

World's oases threatened by desertification, even as humans expand them

NASA's Voyager 1 resumes sending engineering updates to Earth

Researchers break down pizza box recycling challenges, one slice at a time

Why is methane seeping on Mars? NASA scientists have new ideas

Record-breaking heat and humidity predicted for tropics this summer
2 hours ago

Astrophysics research advances understanding of how gamma-ray bursts produce light
Relevant physicsforums posts, major evolution in action, the cass report (uk).
Apr 20, 2024
If theres a 15% probability each month of getting a woman pregnant...
Apr 19, 2024
Can four legged animals drink from beneath their feet?
Apr 15, 2024
Mold in Plastic Water Bottles? What does it eat?
Apr 14, 2024
Dolphins don't breathe through their esophagus
More from Biology and Medical
Related Stories

Live from the brain: Visual cues inform decision to cooperate
Feb 14, 2024

Researchers decode neuronal basis of decision-making processes to predict actions
Mar 6, 2024

Re-frame of mind: Do our brains have a built-in sense of grammar?
Jan 9, 2024

Study suggests the brain's reward system works to make others happy, not just ourselves
Apr 16, 2024

How social behavior is encoded in the monkey brain during everyday tasks
Mar 15, 2024

New study shows how the brain translates motivation into goal-oriented behavior
Recommended for you.

Lemur's lament: When one vulnerable species stalks another

Why zebrafish can regenerate damaged heart tissue, while other fish species cannot

Mycoheterotrophic plants as a key to the 'Wood Wide Web'

Global study finds there really are more insects out after dark

Seeing is believing: Scientists reveal connectome of the fruit fly visual system
Let us know if there is a problem with our content.
Use this form if you have come across a typo, inaccuracy or would like to send an edit request for the content on this page. For general inquiries, please use our contact form . For general feedback, use the public comments section below (please adhere to guidelines ).
Please select the most appropriate category to facilitate processing of your request
Thank you for taking time to provide your feedback to the editors.
Your feedback is important to us. However, we do not guarantee individual replies due to the high volume of messages.
E-mail the story
Your email address is used only to let the recipient know who sent the email. Neither your address nor the recipient's address will be used for any other purpose. The information you enter will appear in your e-mail message and is not retained by Phys.org in any form.
Newsletter sign up
Get weekly and/or daily updates delivered to your inbox. You can unsubscribe at any time and we'll never share your details to third parties.
More information Privacy policy
Donate and enjoy an ad-free experience
We keep our content available to everyone. Consider supporting Science X's mission by getting a premium account.
E-mail newsletter

An official website of the United States government
The .gov means it’s official. Federal government websites often end in .gov or .mil. Before sharing sensitive information, make sure you’re on a federal government site.
The site is secure. The https:// ensures that you are connecting to the official website and that any information you provide is encrypted and transmitted securely.
- Publications
- Account settings
Preview improvements coming to the PMC website in October 2024. Learn More or Try it out now .
- Advanced Search
- Journal List
- Conserv Physiol
- v.7(1); 2019
Chronic captivity stress in wild animals is highly species-specific
Clare parker fischer.
Department of Biology, 200 College Ave. Tufts University, Medford, MA 02155 USA
L Michael Romero
Lay summary:
A review that compares changes in body mass, glucocorticoid and sympathetic responses, and reproductive and immune function, in wild animals recently introduced into captivity to their wild counterparts. Conclusion is that captivity can be a powerful chronic stressor that may be possible to mitigate, but the impact is highly species-specific.
Wild animals are brought into captivity for many reasons—conservation, research, agriculture and the exotic pet trade. While the physical needs of animals are met in captivity, the conditions of confinement and exposure to humans can result in physiological stress. The stress response consists of the suite of hormonal and physiological reactions to help an animal survive potentially harmful stimuli. The adrenomedullary response results in increased heart rate and muscle tone (among other effects); elevated glucocorticoid (GC) hormones help to direct resources towards immediate survival. While these responses are adaptive, overexposure to stress can cause physiological problems, such as weight loss, changes to the immune system and decreased reproductive capacity. Many people who work with wild animals in captivity assume that they will eventually adjust to their new circumstances. However, captivity may have long-term or permanent impacts on physiology if the stress response is chronically activated. We reviewed the literature on the effects of introduction to captivity in wild-caught individuals on the physiological systems impacted by stress, particularly weight changes, GC regulation, adrenomedullary regulation and the immune and reproductive systems. This paper did not review studies on captive-born animals. Adjustment to captivity has been reported for some physiological systems in some species. However, for many species, permanent alterations to physiology may occur with captivity. For example, captive animals may have elevated GCs and/or reduced reproductive capacity compared to free-living animals even after months in captivity. Full adjustment to captivity may occur only in some species, and may be dependent on time of year or other variables. We discuss some of the methods that can be used to reduce chronic captivity stress.
Introduction
The tens of thousands of vertebrate species on this planet are adapted to every condition from the Arctic to the tropics and from the mountain tops to the ocean depths. For all species, the environment contains both predictable changes (e.g. day–night transitions or seasonal variation) and unpredictable, uncontrollable threats to homeostasis and survival ( Romero and Wingfield, 2016 ). Vertebrates have evolved a suite of defenses against the myriad unpredictable ‘shocks that flesh is heir to’ (Shakespeare, Hamlet , 3.1)—a set of conserved physiological responses known as the ‘stress response’. While the stress response can help an animal survive a threatening event, if noxious conditions are repeating or unrelenting two physiological changes take place. First, the reactive scope of the animal shrinks thereby decreasing the animal’s ability to cope ( Romero et al ., 2009 ). Second, the stress response itself can begin to cause physiological problems, a condition known as ‘chronic stress’. Even though there is no generally agreed upon definition of chronic stress or the time-frame of its onset, long-term stressor exposure or chronic stress, can lead to weight loss, immunosuppression, reproductive failure and psychological distress ( Sapolsky et al ., 2000 ). Because the stress response occurs when situations are perceived as threatening, regardless of whether the animal is experiencing physical damage, a drastic change of conditions can lead to symptoms of chronic stress even when the animal is unharmed. Consequently, when a wild animal is brought into captivity for the first time, symptoms of chronic stress can occur even though the physical needs of the animal are attended to.
In captivity, animals are provided with shelter and ample food. Nevertheless, captivity can often result in negative physiological outcomes, particularly for newly-captured animals. The conditions of captivity can be perceived as threatening, and if the perceived threat does not decrease, symptoms associated with chronic stress may result. The sources of stress in captivity are many, including cage restraint, human presence, an unfamiliar environment, and other, more subtle stressors, such as artificial light conditions (reviewed in Morgan and Tromborg, 2007 ). When wild animals are newly brought into captivity, it is frequently for research, conservation, agriculture (e.g. fisheries) or the exotic animal trade. To keep these animals healthy, symptoms of chronic stress should be minimized or eliminated. It is often assumed that with time, animals will adjust to captivity conditions and stress will disappear. Indeed, many animals seem to thrive in captivity. Unfortunately, many other species do not ( Mason, 2010 ). In this review, we surveyed the literature to answer the following two questions: do wild animals eventually adjust to captivity conditions? And if so, how long does the period of adjustment typically take? This literature survey exclusively addressed wild animals introduced to captivity and not animals born in captivity.
We focused on several aspects of physiology that may be particularly affected by long-term stressor exposure. The acute stress response involves many behavioral and physiological changes, including activation of two hormonal pathways. The adrenomedullary response occurs within seconds of the onset of a stressor ( Romero and Wingfield, 2016 ). The catecholamine hormones epinephrine and norepinephrine are rapidly released from the adrenal medulla. These cause an increase in heart rate, as well as an increase in muscle tone, an increase in blood pressure and other physiological and behavioral changes that enable an animal to survive a sudden stressor, such as a predator attack. The second hormonal response is initiated within minutes of the onset of a stressor, when a hormonal cascade triggers the synthesis and release of glucocorticoids (GCs)—steroid hormones that have wide-ranging effects on the body ( Romero and Wingfield, 2016 ). While baseline levels of GCs help regulate metabolism, increased levels trigger an ‘emergency life history stage’, ( Wingfield et al. , 1998 ), where resources and behaviors are directed towards survival of the crisis and away from long term investments. GCs have a strong impact on the immune and reproductive systems ( Sapolsky et al ., 2000 ). In this review, we focus on captivity’s effects on mass (one of the best-documented outcomes of chronic stress), GC concentrations and the immune, reproductive and adrenomedullary systems. We also document how the adjustment to captivity is impacted by time of year and how captivity effects persist after release. Finally, we discuss some of the ways that captivity stress may be mitigated.
We surveyed the literature and gathered studies that compared wild-caught animals as they adjusted to captivity. We conducted a literature search through Web of Science using the search terms ‘captivity’ and ‘stress’ and ‘physiology’ or ‘endocrinology’ and related words. Because many papers reported on aspects of the stress response on animals that were in captivity but did not examine the effects of captivity itself, we were unable to devise search terms that included the studies we were interested in but excluded other research on stress in wild animals. We therefore devised the following criteria to determine whether papers should be included: (i) wild species were brought into captivity and physiological variables measured over the days to months of adjustment to captive conditions OR (ii) wild-caught captive animals were compared to free-living conspecifics AND (iii) the total captivity duration was at least 3 days (we did not include the many studies that measure only the acute stress effects of capture in the first 30 min to 48 hours). We further excluded two broad types of studies. One, we excluded studies where we could determine that all captive animals were captive-bred, as we were specifically interested in how well wild animals can adjust to captive conditions when taken from the wild (though we included some studies where the origin of captive animals was unclear). Second, we excluded studies of wild animals undergoing rehabilitation because it is not possible to distinguish between responses to captivity and responses to clinical interventions in animals that were injured or sick at capture. Once we had created a list of papers, we also checked the cited references of these studies for any important works our search terms missed.
There are many studies that focused on behavioral changes in captivity. However, the variables measured can be quite species-specific and difficult to interpret in a context of stress. Although we recognize the importance of behavior for the welfare of wild animals (reviewed in McPhee and Carlstead, 2010 ), we limited our focus to studies that included some physiological measurements (e.g. weight changes, hormone concentrations or immune measurements).
We found little standardization in experimental design in the papers examining the effect of captivity on physiology. We visually summarize the four most common experimental designs in Fig. 1 . Many researchers compared animals that had been exposed to captivity (duration: 3 days to several years) to those that had not ( Fig. 1A ). In some cases, the free-living population was sampled when the captive population was initially captured. This was often the case in species where only a single blood sample could be drawn from an individual. In other studies, the free-living population was sampled entirely separately from the captive group. This was often the case for long-term captives, such as zoo-housed animals. Another common technique was to take a single pre-captivity sample and a single post-captivity sample on the same animal (duration of captivity 5 days to 3 months) ( Fig. 1B ). Other researchers used repeated sampling techniques—either sampling the same individual multiple times, or keeping different individuals in captivity for different durations before sampling. Some focused narrowly on the first few days of captivity ( Fig. 1C ), while others did not take a second sample until several weeks had passed ( Fig. 1D ). Furthermore, captive conditions varied between studies, with some studies bringing animals into closed indoor situations, whereas others placed captive animals into open outdoor pens. We considered each situation to represent captivity, but we were not able to contrast any differences in responses.

Examples of experimental designs to assess the effects of captivity on a physiological variable (e.g. GC concentration) ( A ) Comparison of captive individuals to free-living populations. In some cases, the free-living samples were acquired at the same time that the study population was brought into captivity. In other designs, the free-living samples were taken from entirely different populations than the origin of the captive animals (e.g. comparing zoo-housed animals to wild conspecifics). ( B ) Each individual measured immediately at capture and again after a period of captivity (days to months). ( C and D ) Each individual measured immediately at capture and resampled at multiple timepoints. Some studies focused on the first few days, with sampling points relatively close together (C). Other studies may not have taken another sample until several weeks after capture (D).
We created summary figures for the trends we observed in weight, GC hormones and the immune system with respect to captivity duration ( Figures 2 – 4 ). To construct these, we tallied the total number of studies that reported on the variable for a particular time window and determined whether the variable was above, below or equal to what it was in a free-living population. If a single report showed two different patterns (e.g. males and females had different patterns or two species were reported in the same paper), each pattern of was included separately. Therefore, one ‘study’ might be included multiple times in the figure. This also holds true for reporting patterns in the literature in the text and in the tables—if one paper reported multiple patterns in different groups of individuals, it was included more than once in calculating percentages of studies and was given more than one line on the tables. We did not include studies in the figures if there were marked seasonal differences in one species (see Section 9 for seasonal differences).

Weight change as a function of captivity duration. Data were collected from 35 studies listed in Table 1 , with studies counted multiple times if they measured multiple time points after introduction to captivity. The number of species that lost weight in captivity (relative to wild, free-living animals) decreased with captivity duration.

Changes in neutrophil or heterophil (N or H:L) to lymphocyte ratio in captivity as a function of time. Data were collected from 19 studies listed in Table 4 , with studies counted multiple times if they measured multiple time points after introduction to captivity. The percent of studies that recorded elevated N or H:L ratio in captivity decreased with the amount of time spent in captivity.
Because most of the papers we collected did not report effect sizes, a formal meta-analysis was not possible. Consequently, we focused on qualitative differences.
Mass and body condition in captivity
After being brought into captivity from the wild, animals frequently experience a period of weight loss ( Table 1 ). In 64% of studies (23 of 36), there was a documented decrease in mass associated with captivity during at least the initial capture period. Weight loss in captivity is likely to be attributable to chronic stress. Captive animals are not calorically restricted (as long as they choose to eat), which is not always the case in the wild, and they are not likely to use as many calories because cage restraint limits the amount of exercise that an animal can get in a day. Experimentally induced chronic stress has been demonstrated to lead to weight loss in mammals (e.g. Flugge, 1996 ), birds (e.g. Rich and Romero, 2005 ) and fish (e.g. Peters et al., 1980 ). In fact, weight loss is the most consistently seen effect of chronic stress ( Dickens and Romero, 2013 ).
Mass changes with captivity in wild animals
1 No at-capture values—first measured at 2 months.
2 Low sample sizes at each time point.
3 Captive pups were rehabilitated after rescue.
4 Slight weight loss from Day 10 to Day 60.
5 Females did not reach at capture weight, but all spontaneously aborted or gave birth.
* Data from this paper were used to generate Fig. 2 .
In 39% of studies where animals lost weight (9 of 23), the animals eventually regained the weight they had lost. In some cases, weight loss may be very transitory and last only a couple of days. For example, North Island saddlebacks (a bird native to New Zealand) lost weight on the first day of captivity, but by Day 3, they had not only regained weight, they were heavier than they were at capture ( Adams et al ., 2011 ). Transitory weight loss may be related to adjustment to the captive diet and not to major physiological problems. In other species, it may take weeks or months to regain the lost mass. House sparrows lose weight by Day 5–7 of captivity ( Lattin et al ., 2012 ; Fischer and Romero, 2016 ). In a long-term study of the species, they did not regain the weight they had lost for nearly 5 weeks ( Fischer et al ., 2018 ). Similarly, female possums lost weight for 5 weeks before beginning to gain again, and although they were kept for 20 weeks, they never fully recovered their lost weight ( Baker et al ., 1998 ). In 61% of studies (14 of 23), weight that was lost was never regained, though the studies may not have been long enough for weight to stabilize.
In some cases, weight loss depended on the characteristics of the animal at capture. For example, female possums lost weight over the first 5 weeks of captivity but some males gained weight during that period ( Baker et al ., 1998 ). When curve-billed thrashers were captured, birds from urban environments had higher body condition than desert birds, but after 80 days in captivity, their body conditions had converged to an intermediate value ( Fokidis et al ., 2011 ). Captivity may impact individuals differently depending on sex, population of origin or other individual characteristics, including transitory physiological states. (See Section 9 for the effects of time of year on the ability to adjust to captivity.)
Weight loss was not the only pattern seen in captivity. In 17% of studies (6 of 36), animals gained mass above their starting condition. Some animals may benefit from the increased calories available in captivity and be able to maintain their weight. In other animals, however, ad libitum access to food and limits to exercise may cause them to become obese and face the myriad negative consequences of a high body mass or body fat content ( West and York, 1998 ). In a study of domesticated budgerigars, birds were given ad libitum food and confined to cages that limited exercise. High body mass at the end of 28 days correlated with more DNA damage ( Larcombe et al ., 2015 ).
We visually summarized the patterns of weight changes in Fig. 2 . We graphed the total percent of studies that showed weight gain, weight loss or no change in weight at different time points after introduction to captivity. There were no studies that recorded weight gain in the first day. Most weight gain seems to be reported at 15–28 days of captivity (38% of studies showed weight gain in that window). The percent of studies reporting weight loss decreased with increasing captivity duration, reflecting the fact that many studies show eventual regain of lost weight. This suggests that for many species where weight was lost, it would eventually be regained.
It is possible that seasonal fluctuations in weight may interfere with the assumptions that weight gain or loss is due to captivity. Captive ruffs and red knots have strong seasonal weight fluctuations in captivity associated with weight gain for migration and breeding ( Piersma et al., 2000 ). If semi-naturalistic conditions are maintained in captivity (for example, if the animals are exposed to natural day length or are housed outdoors), then they may continue to experience seasonal weight changes that are not due to overfeeding or to long-term stressor exposure.
Changes in GCs during the adjustment to captivity
One of the most common variables to measure when assessing the stress of captivity was GC concentrations. GC hormones (primarily cortisol in fish and most mammals; primarily corticosterone in reptiles, birds, amphibians, and rodents) are produced in the adrenal cortex, have multiple roles throughout the body, and can influence many other physiological systems. Acute stressors cause a transitory increase in GCs, which is eventually brought down by negative feedback. Long-term stressor exposure frequently results in changes in GC regulation, although the part of the GC response affected (baseline concentrations, stress-induced concentrations, or negative feedback) and the direction of the change are different in different species and circumstances ( Dickens and Romero, 2013 ).
GCs can be assessed in several ways ( Sheriff et al., 2011 ). The most common method is to measure circulating plasma GCs by taking a blood sample. The sampling procedure itself can cause an increase in GCs, so researchers usually try to acquire the first sample as quickly as possible—within 3 minutes of capture or disturbance is generally considered a good guideline ( Romero and Reed, 2005 ). In many studies, it was not possible for the researchers to meet this standard because of the difficulty of capturing and bleeding the animals. In addition, some papers were written before the 3-minute standard had been established. It is also possible to assess GCs through other means. Fecal samples can be collected to measure metabolized GCs. Fecal samples provide an integrated profile of GC secretion over several hours to several days, depending upon the species, and reflect both baseline GCs and acute stress events ( Wasser et al ., 2000 ). Fecal sampling is convenient for many species when rapid capture and blood sampling is impractical. If the first fecal sample is collected soon after capture, it will not reflect the stress of captivity and may be considered a good free-living reference. Some researchers also used urinary GC metabolites, particularly in amphibian species, where animals could be left alone in a container of water from which excreted steroids were measured.
The initial capture and handling of wild animals is expected to cause an increase in circulating GC levels (an acute stress response). While some researchers investigated captivity-induced changes in the acute stress response itself (e.g. taking a plasma sample after a standardized 30-minute restraint stress at capture and again after a period in captivity), others incorporated the acute response to capture in the same analysis as longer-term captivity effects (e.g. taking a sample at 0, 2, 6, 18, 24, 48 and 72 hours post capture). Because of the variety of different measures used, we focused particularly on the captivity effects on baseline and integrated GCs ( Table 2 ). However, we will also discuss the effects of captivity on the acute stress response and negative feedback of GC production ( Table 3 ). Some researchers looked for the effects of captivity at different times of year—we do not include those studies in our calculations or in Tables 2 and and3 3 (see Section 9 ).
Patterns of change in baseline and integrated GCs when wild animals are brought into captivity (this table does not include studies where the pattern was different in different seasons—those studies may be found in Table 6)
1 Cortisol results only.
2 No difference in GCs in females pre-breeding—GCs elevated in both sexes during breeding season.
3 Captive population may include some captive-raised individuals.
4 Blood sampling took longer in some samples.
5 GC spike in many animals during first 2 weeks, but then drops well below at capture levels.
6 GCs increased in non-calling toads, but sample sizes low.
7 Some animals treated with long-acting neuroleptic, which had no effect on GC levels, so values were pooled.
* Data from this paper are incorporated into Fig. 3 .
Patterns of change in stress-induced GCs and negative feedback with captivity in wild animals
1 SI GCs lower post captivity in early winter, but no change during any other time of year.
2 Outside of breeding season and molt.
3 During the breeding season.
4 During the post-breeding/molting season.
5 SI GCs higher post captivity in pre-breeding and breeding season, not in winter.
Captivity does not influence GCs in all species. In 17% (10 of 59) studies, there was no recorded difference in GCs during or after the captivity period compared to free-living levels. In most studies, however, captivity caused a change in baseline or integrated GCs. In 42% of studies (25 of 59), wild animals had increased GCs at the end of the capture period compared to concentrations in free-living animals (periods of 3 days to several years). Elevated GCs are traditionally interpreted as an indication that animals are chronically stressed. Experimentally induced chronic stress can often lead to elevated baseline GCs, although this is by no means a universal response ( Dickens and Romero, 2013 ). Adrenal hypertrophy may be an underlying mechanism explaining the long-term elevation of GCs. For example, long-term captivity led to increased adrenal mass in African green monkeys ( Suleman et al., 2004 ) and mouse lemurs ( Perret, 1982 ). In nine-banded armadillos, 6 months of captivity (but not 3 months) caused adrenal changes similar to those after a harsh winter ( Rideout et al., 1985 ) and in herring gulls 28 days of captivity led to adrenal lesions ( Hoffman and Leighton, 1985 ).
However, many studies that reported elevated GC concentrations at the end of the captivity period may eventually have shown decreased GCs had the study been carried out for longer. For example, house sparrows had elevated baseline GCs after 1–7 days in captivity ( Kuhlman and Martin, 2010 ; Lattin et al., 2012 ; Fischer and Romero, 2016 ). But when captive house sparrows were sampled repeatedly over 6 weeks of captivity, the high baseline GCs seen at Day 7 were dramatically reduced over Days 11–42 and approached at-capture concentrations in one study ( Fischer et al ., 2018 ), but did not decrease in another study ( Love et al ., 2017 ).
The duration of captivity in the studies we collected was quite variable, ranging from 3 days to several years. To consolidate the patterns from multiple studies with different sampling times, we graphed the percent of studies with elevated GCs (relative to free-living levels) against captivity duration ( Fig. 3 ). We expected the percent of studies with elevated GCs to decrease as captivity duration increased (as shown in Fig. 1C and D ). This pattern would indicate an adjustment to captivity conditions and is a typical a priori prediction in the literature. However, we found that 45% (5 of 11) of species continued to have elevated GCs after 3 months or more of captivity. This suggests that for many species, there is never a complete adjustment to captivity. It is also possible that a publication bias exists in the papers we collected. When researchers did not see a difference between long-term captives and free-living animals, they may have been less likely to publish, or perhaps included those results in other studies that did not appear in our literature searches. It is interesting to note that the fewest studies reported elevated GCs at around two weeks post captivity, the amount of time that many researchers allow for their study species to become acclimated to laboratory conditions (e.g. Davies et al., 2013 ; Lattin and Romero, 2014 ; McCormick et al., 2015 ).

Change in baseline or integrated GCs as a function of captivity duration. Data were collected from the 47 studies listed in Table 3 that had a well-defined wild baseline value (i.e. plasma samples were collected within minutes of capture; fecal or urine samples were collected shortly after capture), with studies counted multiple times if they measured multiple time points after introduction to captivity. This figure does not include studies with seasonal effects on the GC response to capture.
The analysis in Fig. 3 contains data collected from many different taxa, study designs, etc. A more informative methodology to investigate how GCs change over time in captivity is to compare multiple timepoints within the same experiment. We found 38 studies that used repeated sampling. Researchers either repeatedly sampled individuals or captured many subjects at once and sampled them after different captivity durations. In study designs with repeated sampling, 42% of studies (16 of 38) showed an early increase in GCs followed by a decrease back to free-living levels (e.g. Fig. 1C and D , the a priori prediction for GC adjustment to captivity). Of the remaining studies, 32% (12 of 38) matched the pattern in Fig. 3 with no decrease in GC concentrations over time, 13% (5 of 38) showed decreased GC concentrations in captivity and 11% (4 of 38) reported no change in GCs whatsoever. When the expected peak and fall of GCs was observed, the timescale of adjustment to captivity varied. Baseline GCs in mouse lemurs returned to at-capture levels by Day 5 ( Hamalainen et al ., 2014 ) while the Fijian ground frog had elevated urinary GCs until Day 25 post capture ( Narayan and Hero, 2011 ).
In some studies with repeated measures designs, the researchers did not or could not obtain a sample that represented free-living animals. In these cases, the first sample could not be acquired until minutes, hours or even days after capture. In all nine studies where this was the case (see Table 2 ), initially high concentrations of GCs decreased over the study period in at least some animals. This is consistent with the pattern we expect for animals successfully adjusting to captivity: capture, handling and the initial transfer to captivity result in high GCs that decrease as the animal adjusts. For example, female brushtail possums were not sampled until days after their capture and transfer to captivity, but showed decreasing plasma GCs from week 1 to week 20 of captivity ( Baker et al ., 1998 ).
These studies on baseline GCs together demonstrate a pattern wherein approximately half of species appear to adjust to captivity. Although some species seem to take longer to acclimate to captive conditions than others, it appears that many species will eventually show a reduction in GCs after an initial peak. We see this pattern across taxonomic groups, in birds, fish, reptiles, amphibians and mammals. However, we should be careful to not interpret a reduction in circulating baseline GCs, fecal GC metabolites or urinary GCs as a complete adjustment to captivity or an elimination of chronic stress. Even when baseline GCs have returned to free-living levels, other aspects of the animals’ physiologies may be negatively impacted. For example, even though circulating GCs were only elevated for 1 day in African green monkeys, adrenal mass was almost doubled after 45 days in captivity ( Suleman et al ., 2004 ). Similarly, while it is tempting to conclude that elevated GCs are diagnostic of chronic stress, it should be kept in mind that baseline GCs have many functions in metabolism and energy use. A change of baseline GCs in captivity could merely reflect a change in energy requirements and not the physiological damage we associate with chronic stress. Furthermore, a reduction in GCs in captivity, as seen in 14% of studies (8 of 59), could be interpreted as a reduction in allostatic load or as the exhaustion of adrenal capacity.
Impact of captivity on acute stress response and negative feedback of GC production
Relatively few researchers have explicitly investigated the effects of captivity on the acute GC stress response (see Table 3 ). Of those that have, 65% (11 of 17) found no effect of captivity (captivity duration 5–80 days). The six studies that reported changes in stress-induced GCs showed changes in opposite directions. In two studies, stress-induced GCs were decreased in captivity, even though the captive periods of 9 days ( Dickens et al., 2009a ) and 1 year ( Romero and Wingfield, 1999 ) were quite different. In contrast, stress-induced GCs were increased in captivity in four studies over similar time frames. Three studies had animals in captivity for about a year ( Romero and Wingfield, 1999 ; Berner et al ., 2013 ; Quispe et al ., 2014 ), with 5–8 days in the fourth study ( Sykes and Klukowski, 2009 ).
The negative feedback of the GC response to stress, where high GC levels lead to the inhibition of GC production, is very important for the control of physiological stress ( Vitousek et al ., 2019 ). Although chronic stress has frequently been found to affect the negative feedback of GC production ( Dickens and Romero, 2013 ), we found only three studies that explicitly measured negative feedback strength in animals immediately at capture and after a period of captivity. In each case, animals were injected with a synthetic GC (dexamethasone) after mounting a stress response to stimulate maximum negative feedback. The strength of negative feedback increased slightly in house sparrows after 5 days of captivity ( Lattin et al ., 2012 ), but in the same species showed no change after 21, 42 or 66 days ( Love et al ., 2017 ). In contrast, negative feedback strength decreased after 5 days of captivity in chukar partridges but returned to its at-capture strength by 9 days ( Dickens et al ., 2009b ). This is an important aspect of stress physiology, one that is critical for the total amount of GC exposure, and warrants further study to determine whether it is impacted by the stress of captivity in many species.
Immune consequences of captivity
Stress has well-documented, but sometimes complex, effects on the immune system. In large part, these changes are due to the acute or long-term effects of elevated GCs on leukocyte populations. GCs can cause immune redistribution, moving lymphocytes out of the bloodstream and into the skin, spleen and lymph nodes, where they will be available in case of a wound ( Dhabhar and McEwen, 1997 ; Johnstone et al ., 2012 ). GCs can also cause proliferation or mobilization of neutrophils (most vertebrates) or heterophils (birds and some reptiles) ( Dale et al ., 1975 ; Gross and Siegel, 1983 ; Johnstone et al ., 2012 ). Together, these effects on leukocyte populations result in a change in the neutrophil or heterophil to lymphocyte ratio (N or H:L ratio) ( Dhabhar and McEwen, 1997 ; Johnstone et al ., 2012 ). A change in the N or H:L ratio does not necessarily mean that an animal’s immune system is hypo- or hyperactive. Instead, this acts as another metric similar to GC secretion. A long-term increase in N or H:L ratio, like a long-term increase in circulating GCs, can be an indication that an animal is suffering from chronic stress ( Davis et al ., 2008 ).
We summarized the 23 studies that reported leukocyte counts in Table 4 . Although the N or H:L is a useful metric, in some studies the researchers chose to report total number or percent of different leukocyte types without calculating or performing statistics on the relative abundances of neutrophils/heterophils and lymphocytes. In these cases, we inferred the direction (or presence) of change after captivity of the N or H:L ratio based on the changes in leukocyte counts or percentages that were reported. In two studies, only the total number of leukocytes was reported without further subdivision of leukocyte types. In 48% of studies (10 of 21), N or H:L ratio was elevated at the end of the measured captivity duration relative to its free-living value. 29% of studies (6 of 21) documented no change in N or H:L ratio over the study period. N or H:L ratio was decreased in 24% of studies (5 of 21). In one study (in the Fijian ground frog), the N:L ratio was elevated for 15 days in captivity, but then returned to wild levels by Day 25, resulting in no overall change ( Narayan and Hero, 2011 ). Kuhlman and Martin (2010) further investigated leukocyte redistribution to the skin in house sparrows, comparing Day 1of captivity to Day 30. They concluded that the changes in H:L ratio were not due to redistribution of leukocytes, at least in this instance. We summarized the overall patterns of N or H:L ratio compared to captivity duration in Fig. 4 . The number of studies reporting an increase in N or H:L ratio decreases with captivity duration. This suggests that many or most species do adjust to captivity, and an initially high N or H:L ratio may decrease given sufficient time.
Changes in leukocytes during captivity
Timeframe refers to the longest duration of captivity measured. WBC = total white blood cells; H = heterophils; N = neutrophils; L = lymphocytes; n.c. = not calculated (in this case, a count or percentage of heterophils or neutrophils and lymphocytes was measured in the paper, but H or N:L ratio was not directly compared. Presence/direction of change in the rctypes); ↑ or ↓ = higher or lower than free-living; – = no change from free-living.
1 Pattern only seen in rhinos translocated from high to low (not high to high) elevation.
2 Total WBCs and N:L ratio also compared to free-living wild populations of a similar species—there was no difference.
3 Comparison to values collected in another study and species (llamas and alpacas).
4 Circulating leukocytes and skin-infiltrating leukocytes were measured. See text for skin leukocyte patterns.
Some studies also reported the total leukocyte counts, sometimes without further subdividing them into classes. While decreased circulating leukocytes has been associated with stress (generally because of redistribution rather than destruction of cells) ( Dhabhar, 2002 ), there was no clear pattern with the number of leukocytes in captivity. 53% of studies (9 of 17) showed no change in total white blood cells compared to free-living animals by the end of the captivity period; 23.5% (4 of 17) showed a decrease in circulating leukocytes; and 23.5% (4 of 17) showed an increase (captivity duration 3 days to 1 year, see Table 4 ).
Importantly, neither total leukocyte numbers nor the N or H:L ratio provide a very strong indication of immune capacity. Some researchers have used more direct measurements of immune functionality. The bacterial killing assay is a way to determine how effectively fresh whole blood can eliminate bacteria. This assay has the advantage of determining the real effectiveness of the immune system against pathogens ( Millet et al ., 2007 ). In the cururu toad, whole blood was less effective at killing bacteria after 13 days of captivity ( de Assis et al ., 2015 ) and in two other toad species, killing capacity decreased by 60 but not 30 days ( Titon et al ., 2017 , 2018 ). Similarly, in red knots held in captivity for 1 year, whole blood was less effective at eliminating two Staphylococcus species than in wild living birds (though there was no difference in Escherichia coli elimination) ( Buehler et al ., 2008 ). In contrast, there was an increased proportion of E. coli killed after 3 weeks of captivity in house sparrows ( Love et al ., 2017 ).
Another way to measure immune responsiveness is by measuring a proliferative response against non-specific antigens. In some studies, this is done by culturing a sample of blood along with an antigen and quantifying cell division. In male brushtail possums, the proliferative response to the plant toxin phytohemagglutinin decreased over 20 weeks but increased by 1 year ( Baker et al ., 1998 ). In female possums, the proliferative response increased from 11 to 15 weeks in captivity, and then remained at that high level for at least a year ( Baker et al ., 1998 ). In another study in male brushtail possums, leukocyte proliferation to a Mycobacterium protein derivative increased after 4 and 6 weeks of captivity, but only when the animals were housed in high-density pens to create crowding ( Begg et al ., 2004 ). The proliferative response to phytohemagglutinin can also be measured in-vivo if PHA is injected into the skin and the degree of swelling is quantified. In zebra finches, there was no difference in the in vivo PHA response between newly captured birds and those held for 10 or 16 days ( Ewenson et al ., 2001 ).
Two studies have attempted to quantify the strength of the adaptive immune system in captivity. In red knots, plasma was plated with rabbit red blood cells. The degree of hemolysis and hemagglutination provided a measure of complement and natural antibody action. Hemolysis and hemagglutination were similar in wild and captive birds when they were measured at the same time of year, which suggests that the strength of the adaptive immune response is unaffected by captivity ( Buehler et al ., 2008 ). Conversely, newly captured killifish had a stronger response to antigen after immunization than 4–6-week captives, suggesting that the adaptive immune system was less effective after captivity ( Miller and Tripp, 1982 ).
Overall, there does not seem to be a single pattern for immune regulation with captivity. While captivity has been shown to repress immune function in some species (e.g. reduced bacterial killing in red knots and toads), in other species, the immune system may be hyperactivated. For example, in house sparrows, gene expression for pro-inflammatory cytokines was elevated in captive birds (2- and 4-week captives) compared to newly caught animals, which was interpreted as hyperinflammation in captive birds ( Martin et al ., 2011 ). Changes in the immune response with chronic stress are thought to be most strongly tied to GC release. However, the impacts of GCs on the immune system can be complex. In the short term, GCs typically induce an immune response, while they can be immunosuppressive over the long term, although these interactions tend to be context-dependent ( Dhabhar and McEwen, 1997 ; Martin, 2009 ). As the interaction between GCs and immunity is complex and context specific, and as the interaction of GCs to captivity can be complex as well (see Changes in GCs during the adjustment to captivity ), it is not currently possible to predict whether captivity conditions will result in appropriate or inappropriate immune activity. However, there has been limited work in this area.
Effects of captivity on the reproductive system
Captivity has well-documented negative impacts on reproductive biology. In many species, captive breeding for research or conservation purposes can be a challenge. Even the house sparrow, so commonly used as a model species, does not readily breed in captivity ( Lombardo and Thorpe, 2009 ). In 74% of studies (17 of 23), the transition to captivity resulted in reduced reproductive capacity in wild species ( Table 5 ). Note, however, that these papers do not cover an extensive literature on captive breeding, including in individuals who have spent decades in captivity or were born in captivity, which is beyond the scope of this review. Here, we focus only on those papers that studied reproductive capacity of recent captives (only within the first year) and that examined a mechanism for reduced reproduction. There was no obvious taxonomic pattern for species that had reduced reproductive ability in captivity compared to those that had no documented reproductive problems. Duration of captivity did not appear to be a factor either. In one study of water frogs, reproduction in both males and females were negatively impacted by only 3 days of captivity ( Zerani et al ., 1991 ), while in jack mackerel, reproduction was inhibited after a full year of adjusting to captivity ( Imanaga et al ., 2014 ).
Reproductive effects of captivity in wild animals (if multiple times of year were examined, only breeding season is included in this table)
1 Different photosimulation and social stimulation tested—maximal testicular regrowth (long days + females) still below wild, though in that group, T was the same as wild.
2 Vitellogenin levels recovered by E2 use.
3 T spikes during the first 24–48 hours of captivity, but decreases below at-capture levels.
4 E2 spikes during first hours of captivity, but quickly decreases below at-capture levels.
5 E2 higher in captive than wild.
6 There was a transitory increase in LH at around Weeks 1–3 that came back to at-capture levels in multiple experiments.
7 T lower in captivity, but only after egg-laying.
8 E2 spike in first 6 hours of captivity but then returns to at-capture levels.
Different researchers measured different variables for reproductive capacity. Many studies analyzed reproductive steroid hormones (primarily testosterone in males and estrogen and/or progesterone in females). However, other variables were also measured, including gonad size and development, behavior and gamete development. In house sparrows, Lombardo and Thorpe (2009) found decreased sperm production, reduced testes size and a change in beak color from breeding-season black to wintering brown after 3 months of captivity. Female anole lizards experienced a rapid decrease in plasma vitellogenin (a protein necessary for yolk production) followed by regression of developing follicles ( Morales and Sanchez, 1996 ). In electric fish, behavioral differences between males and females were reduced in captivity until they disappeared or even reversed. This occurred concurrently with decreases in testosterone and 11-ketotestosterone (a potent fish androgen) in males ( Landsman, 1993 ).
The reduction of reproductive capacity might be tied to GC levels. GCs can be powerful suppressors of reproductive steroids ( Sapolsky et al ., 2000 ). Prolonged GC exposure can lead to decreased production of testosterone or estradiol, which can then have downstream effects on gonad development, egg maturation, sperm production and behavior. In green treefrogs, a decrease in sex steroids was concurrent with an increase in GCs ( Zerani et al ., 1991 ). However, in black rhinos, males had suppressed fecal testosterone and females had suppressed fecal progestins even though GC levels were below free-living levels for most of the captivity period ( Linklater et al ., 2010 ).
Captivity did not always result in suppression of reproduction but in most studies that did not show an effect of captivity, reproductive hormones were the only variables measured. The only exception was in the brown treesnake, where 3 days of captivity did not affect either testosterone or ovarian development (both were very low in free-living and captive animals) ( Mathies et al ., 2001 ). However, another study in brown treesnakes found underdeveloped testes in males after 4–8 weeks of captivity ( Aldridge and Arackal, 2005 ). Captivity may affect sexual variables differently in males and females. For example, in water frogs held in captivity for 2 weeks, only males appeared to be negatively affected by captivity ( Gobbetti and Zerani, 1996 ), which is opposite what is typically expected.
Overall, it appears that captivity tends to have a negative impact on reproduction in most species. However, there are relatively few studies that specifically examine the reproductive physiology of newly-captured animals. Furthermore, given that many animals eventually do breed in captivity while others do not, it is not clear how long-lasting these impacts may be or why they impact some species more than others.
Adrenomedullary effects of captivity
The adrenomedullary arm of the stress response can be difficult to measure. Measuring epinephrine or norepinephrine in the blood is relatively straightforward, but these hormones increase within seconds of disturbance, meaning that acquiring a free-living baseline in a wild animal is difficult without substantial acclimation to human presence. We excluded most studies that measured epinephrine or norepinephrine, as sampling techniques between wild and captive animals differed in ways that would obscure the meaning of their results. For example, plasma norepinephrine under anesthesia (collected within 50 minutes) decreased over 19 months of captivity in rhesus macaques, though a free-living sample could not be obtained under the same conditions ( Lilly et a l., 1999 ), and captive-raised bighorn sheep had a higher epinephrine response to a drop-net capture technique than did free-living sheep, though they had similar norepinephrine responses ( Coburn et al ., 2010 ).
Recording heart rate is another way to infer activity of the adrenomedullary system ( Romero and Wingfield, 2016 ). Heart rate recordings typically involve the use of specialized and expensive equipment, but can give instantaneous updates on heart rate. In addition, scientists can measure heart rate variability, which gives a metric of how much relative control the sympathetic and parasympathetic nervous systems have over heart rate ( Romero and Wingfield, 2016 ). However, depending on the type of heart rate recording device, it may be impossible to obtain baseline free-living heart rates. Although several researchers have had success measuring heart rate in free-living animals (e.g. white-eyed vireos; Bisson et al ., 2009 ), to our knowledge, there has not yet been a study that directly compares heart rate in free-living and captive animals of the same species.
Heart rate has been measured in only a few species during the transition to captivity. In newly-captured bighorn sheep, heart rate during restraint and blood sampling decreased from Days 1–2 (when the animals were handled extensively and transported) until Day 14 (Franzman, 1970). The heart rate of newly-captured European starlings was high compared to birds held for more than a year in captivity but decreased to the level of long-term captives within 24 hours ( Dickens and Romero, 2009 ). The adrenomedullary response to captivity was slightly different in house sparrows. Daytime heart rate was elevated above 1 month captive levels for at least 7 days post-capture ( Fischer and Romero, 2016 ). These data led to a long-term repeated-measures investigation during the first 6 weeks of captivity ( Fischer et al ., 2018 ). Heart rate tended to decrease until Day 18, then plateaued. Furthermore, there was a more profound effect on the heart rate response to a sudden noise in the starling study. While long-term captives showed a robust increase in heart rate after a loud noise, a typical adrenomedullary response, newly-captured birds had a virtually eliminated heart rate response for at least 10 days ( Dickens and Romero, 2009 ). A reduction in the startle response (as demonstrated in European starlings) could have negative consequences for animals that are released from captivity into the wild ( Dickens et al ., 2009a ). The adrenomedullary response to sudden noises or other startling events is an adaptation that allows animals to survive sudden traumatic events, such as predator attacks or conspecific aggression. An impaired startle response could result in death if it persists after the animals are released from captivity.
Overall, there are few studies examining the effects of captivity on the adrenomedullary response. The patterns we see in European starlings and house sparrows are different—it does not appear that there is a consistent heart rate response to captivity in passerine birds, much less in all vertebrates. We believe this is an area ripe for future studies. As telemetry equipment becomes cheaper and more available, we hope to see more investigations into the adrenomedullary response to captivity and other stressors.
Effects of captivity on seasonality of hormone regulation
Some studies examined seasonal differences in the response to captivity. Table 6 shows that the time of year when animals are introduced to captivity can have a profound effect on hormonal changes. For example, baseline GCs might increase when free-living birds are in molt, decrease when free-living birds are breeding, and not change when free-living birds are captured during the winter or spring ( Romero and Wingfield, 1999 ). Furthermore, Table 6 indicates that there is no consistent pattern across seasons or taxonomic groups. The implications of these differences are currently unknown, but the season of capture might partly explain the large variation across studies summarized in Figs 2 – 4 . Understanding why there are seasonal differences in the acclimation to captivity would be an important contribution to this field.
Seasonal effects of captivity
Arrows indicate direction of change in captive animals relative to an established free-living level. Captive and wild measurements were taken at the same time of year. GCs glucocorticoids; T testosterone; E2 estradiol.
1 Delay in acquiring wild baseline.
Other physiological consequences of captivity
Some studies, primarily in marine mammals, reported the effects of captivity on thyroid hormone. Unfortunately, there is not a consistent impact. For example, one study of beluga whales reported that thyroid hormone decreased over the first few days of captivity, but increased to a long-term stable level by day 11 ( Orlov et al ., 1991 ), whereas another study reported that thyroid hormone decreased within the first few days and remained low throughout 10 weeks of captivity ( St Aubin and Geraci, 1988 ). Similarly, rehabilitated harbor seal juveniles held in captivity for 4 months had lower thyroid hormone than free-living juveniles ( Trumble et al., 2013 ). In contrast, long-term captive harbor porpoises had the same thyroid hormone levels as wild populations ( Siebert et al ., 2011 ) and in female brushtail possums, thyroid hormone was elevated from Weeks 6–13, the same period when the animals were regaining weight they had lost in captivity ( Baker et al ., 1998 ). Clearly, more work is needed to determine the effect of captivity on thyroid hormone regulation.
Anatomical changes may also occur in captivity. Mountain chickadees showed remarkable reduction in hippocampal volume after 4 months of captivity ( LaDage et al ., 2009 ), an effect mimicked by black-capped chickadees after 4–6 weeks in captivity ( Tarr et al ., 2009 ). In neither species was the telencephalon affected—the effect was localized to the part of the brain involved in location-based memory tasks. This effect persisted even when the environment was enriched to include memory tasks ( LaDage et al ., 2009 ).
Captivity can lead to various pathologies. In a histological study of mouse lemurs that died spontaneously in captivity, lesions in the kidney were strongly correlated with captivity duration and with adrenal size ( Perret, 1982 ). The investigator also concluded that cardiac disease may result from chronic adrenomedullary stimulation, although they did not measure hormone concentrations directly ( Perret, 1982 ). Similarly, herring gulls developed amyloid deposits in the blood vessels of their spleens after 28 days in captivity ( Hoffman and Leighton, 1985 ). There may be many more hidden anatomical changes resulting from captivity, but few studies have looked for them.
Finally, recent data indicates that captivity can have profound effects at the DNA level. Bringing house sparrows into captivity resulted in an approximately doubling of DNA damage in red blood cells ( Gormally et al ., 2019 ). The impact of this damage on the individual remains to be determined.
Amelioration of captivity stress
Captivity can cause a wide array of physiological changes in wild animals that are consistent with chronic stress and are likely to be detrimental to health. However, can anything be done to prevent these changes? Is there a way to protect animals from the negative consequences of captivity stress? While this is not an exhaustive review of the solutions that have been tried, we offer some ideas that have been attempted to relieve symptoms of chronic stress due to captivity conditions.
Adjusting the physical conditions of captivity may be one of the simplest ways to reduce symptoms of chronic stress. Transferal from outdoors cages to indoors cages led to reduced reproductive hormones and behaviors in long term captive European starlings ( Dickens and Bentley, 2014 ) and to weight loss and reduced immune function in water voles ( Moorhouse et al., 2007 ). Cage size and density are also important for the development of chronic stress. High density housing during the initial captivity period resulted in elevated GCs compared to low density housing in flounders ( Nester Bolasina, 2011 ) and wedge sole ( Herrera et al ., 2016 ). However, reducing density by caging animals individually can have negative consequences, particularly in social species. Housing brushtail possums in groups eliminated the infection, weight loss and mortality that were seen when the animals were caged individually ( McLeod et al ., 1997 ). In male brown headed cowbirds, adding a female to the cage (previously solo housed) resulted in reduced plasma GCs, as well as increased testicular regrowth in photostimulated males ( Dufty Jr and Wingfield, 1986 ).
Many animals benefit from the use of behavioral enrichments to reduce abnormal behaviors that develop in captivity (reviewed in Mason et al ., 2007 ). Enrichments have become standard practice in zoo environments and situations where animals are held long-term or bred in captivity. Enrichments consist of providing animals with the means and motivation to practice a full range of natural behaviors, such as foraging opportunities, exercise opportunities and places to bathe or dust bathe. Even in temporary or laboratory conditions, environmental enrichments can be relatively easy to supply. However, we were unable to find any papers where the physiological benefits of enrichment techniques were specifically tested in newly captured animals. Using these techniques to accelerate the adjustment to captivity would be an exciting avenue for future research.
Lighting conditions may be very important for visual species. European starlings show more behavioral signs of chronic stress under fluorescent lights with a low flicker rate than a high flicker rate ( Evans et al ., 2012 ), but the low flicker rate does not elicit a GC response ( Greenwood et al ., 2004 ). Ultraviolet-deficient lighting resulted in higher baseline GCs in European starlings, although immediately after capture, this stressor may be too subtle to make a difference compared with the other stressors of captivity ( Maddocks et al ., 2002 ). Temperature conditions should also be carefully considered, particularly for poikilotherms. Warm conditions during the initial transfer to captivity resulted in high mortality in sardines ( Marcalo et al ., 2008 ) and higher GCs in cane toads ( Narayan et al ., 2012 ).
Overall, by matching captivity conditions as closely as possible to conditions in the wild, with roomy cages, exposure to naturalistic lighting and temperature conditions and animal densities kept relatively low, many animals will be better able to adjust to captivity and may have reduced chronic stress as a result. However, naturalistic housing conditions may be impractical for many situations. Furthermore, some stressors associated with captivity may be unavoidable. For example, nearly any visual or auditory contact with handlers resulted in a heart rate increase in two red-shouldered hawks ( Patton et al ., 1985 ). Therefore, in some cases, it might be beneficial to use pharmaceuticals to reduce symptoms of chronic stress.
Tranquilizers or sedatives are perhaps the most obvious drug classes to consider using in newly-captured animals. However, these may not be particularly effective at eliminating chronic stress symptoms. A long-acting neuroleptic did not result in many physiological changes in newly-caught otters ( Fernandez-Moran et al ., 2004 ). Tranquilizers did not impact any physiological variable in newly caught impala ( Knox et al ., 1990 ) or red-necked wallabies ( Holz and Barnett, 1996 ), although they reduced behavioral agitation to human approach and handling in the later study. Similarly, a long-acting tranquilizer changed behavior but not heart rate response to human approach in captive wildebeest ( Laubscher et al ., 2016 ). The anxiolytic and sedative diazepam did not affect GCs, heart rate, heart rate variability or activity in house sparrows during the first week of captivity (unpublished personal data). Overall, tranquilizers and sedatives do not appear to have long-term physiological benefits in captive animals. However, they may be useful in the short term. For example, by reducing physical agitation, they may prevent animals from injuring themselves during transport (e.g. in nurse sharks being moved into captivity; Smith, 1992 ) or during necessary handling by humans (e.g. in red-necked wallabies; Holz and Barnett, 1996 ).
Another strategy for pharmaceutical reduction of symptoms of chronic stress may be to chemically block the hormones of the stress response. The chemical agent mitotane causes a reversible chemical adrenalectomy, which drastically reduces circulating GCs ( Sanderson, 2006 ). In house sparrows treated with mitotane immediately upon capture, baseline and stress induced GCs were drastically reduced during the initial captivity period, but recovered to the level of untreated birds by Day 10 of captivity ( Breuner et al ., 2000 ). We investigated the effects of mitotane treatment during the first 7 days of captivity in house sparrows and found that it reduced resting heart rate even when it did not cause the expected dramatic decrease in GC (unpublished personal data). The adrenomedullary response can also be pharmaceutically reduced by blocking the receptors of epinephrine and norepinephrine. We used alpha- and beta-blockers (which interfere with binding of epinephrine and norepinephrine to their receptors) during the first week to block chronic captivity stress in house sparrows. We found that while the beta-blocker propranolol had no effect on heart rate, it did prevent the increase in baseline GCs that we typically see in newly-captured members of this species ( Fischer and Romero, 2016 ).
The persistence of captivity effects after release
The physiological changes caused by captivity can persist even after animals have been released back into the wild. Chukar partridges that were held in captivity 10 days and then released to a new location than where they had originated had lasting changes to their GC regulation (decreased negative feedback for at least 30 days, Dickens et al ., 2009a ). Red foxes that were kept in captivity for 2 to 8 weeks were less likely to establish a stable territory upon release than foxes that were caught and immediately released ( Tolhurst et al ., 2016 ). River otters kept in captivity for 10 months had lower survival than otters not kept in captivity ( Ben-David et al ., 2002 ). The captivity effect was strong enough that crude oil ingestion (mimicking the state of oiled otters in rehabilitation) had no further effect on survival ( Ben-David et al ., 2002 ). Rehabilitated barn owls ( Fajardo et al ., 2000 ) and guillemots ( Wernham et al ., 1997 ) had much shorter life expectancies than wild birds.
However, captivity may not necessarily have lasting negative impacts. In Grevy’s zebra, fecal GC metabolites were elevated in captivity, but decreased back to the wild norm quickly after release ( Franceschini et al ., 2008 ). Similarly, released Eastern Bettongs decreased GC metabolites after release from a period of over 30 days of captivity ( Batson et al ., 2017 ). Hermann’s tortoises kept in captivity for 2–8 years following an injury showed no difference in movement, thermoregulation or body condition compared to free-living animals after release to the wild ( Lepeigneul et al ., 2014 ). Captivity up to 3 months did not affect survival in Stellar’s sea lions ( Shuert et al ., 2015 ). Captivity may even have positive effects in some cases. For example, hedgehogs were more likely to survive a translocation event if they were held in captivity for greater than 1 month compared to those held <6 days ( Molony et al ., 2006 ).
Whether an animal will be permanently negatively impacted by captivity or not may depend on the captivity conditions, species, time of year, method of release or individual effects. Wild rabbits held for 2, 4, 6 or 8 weeks in quarantine before release did not differ in survival probability ( Calvete et al ., 2005 ). In another study in that species, GCs did not change over the course of a quarantine period, but animals with higher plasma and fecal GCs were more likely to survive, even though they had worse body condition ( Cabezas et al ., 2007 ). Saddlebacks were more likely to survive post-release when they had a robust GC response to a standardized acute stressor ( Adams et al ., 2010 ). Therefore, captivity may have more profound effects on survival if it negatively and permanently changes GC regulation.
Conclusions
Captivity can cause weight loss, persistent changes in baseline and integrated GCs, changes in the immune system and reproductive suppression. These effects can last for months or years in some species, indicating that some species may never truly adjust to captivity conditions. The welfare implications of chronic captivity stress are obvious, and zoos and other institutions that hold animals in captivity long-term generally have strategies in place to minimize captivity stress. Breeding facilities (for conservation, research and agriculture/fisheries) are particularly invested in reducing chronic captivity stress, given its profound impact on the reproductive system. Figure 3 indicates that many species may continue to have elevated GCs months or years after capture, while Figs 2 and and4 4 suggest that most animals will recover from the weight loss and elevated N or H:L ratios caused by the initial transfer to captivity. Given that weight loss and changes to N or H:L ratio are affected by GCs, it is possible that with continuing high GC concentrations, sensitivity to these hormones decreases in captive animals. The reproductive system tends to be negatively impacted by captivity, presumably because of elevated GC hormones. The negative effects of captivity are species-specific, some species adjust to captivity while others do not (see also Mason, 2010 ).
A captive animal may be physiologically quite different than a wild animal ( Calisi and Bentley, 2009 ). Therefore, the confounding effects of captivity must be considered in physiological studies using captive wild animals, even when stress is not the focus of research. Animals that are held in captivity for research might respond quite differently to a range of experimental treatments than a wild, free-living individual would. For example, environmental contaminants had different effects on wild and captive sea otters ( Levin et al ., 2007 ), and experimentally induced chronic stress caused a change in fecal GCs in free-living but not captive European starlings ( Cyr and Romero, 2008 ).
The existing literature indicates that the effects of captivity on physiology are inconsistent. Some of the differences between animals that adjust and do not adjust to captivity might be explained by life-history features of the different species (see Mason, 2010 ). For example, captive predators that have large ranges in nature tend to show more behavioral anomalies and more infant mortality than those that naturally have smaller ranges ( Clubb and Mason, 2003 ). However, it may be possible to improve the physiological outcome for newly-captured animals by adjusting the season of capture, improving and enriching housing, allowing for an appropriate adjustment period, and possibly by the careful use of pharmaceuticals. Captivity stress will continue to be a factor in captive animal research, and the conditions, timing and duration of captivity must be considered as experiments are designed and interpreted.
Unfortunately, the results of this literature review do not suggest useful overall and/or generalized guidelines to wildlife managers. The overall picture is that wild animals acclimate to captivity in a highly species-specific manner. However, the most important conclusion from this review is that collecting multiple measures of physiology, rather than restricting studies to a single measure (e.g. GC concentrations), will provide a better picture of how well an individual or species is, or is not, coping with introduction to captivity.
This work was supported by the U.S. National Science Foundation [grant number IOS-1655269 to L.M.R.].
- Adams NJ, Farnworth MJ, Rickett J, Parker KA, Cockrem JF (2011) Behavioural and corticosterone responses to capture and confinement of wild blackbirds ( Turdus merula ) . Appl Anim Behav Sci 134 : 246–255. [ Google Scholar ]
- Adams NJ, Parker KA, Cockrem JF, Brunton DH, Candy EJ (2010) Corticosterone responses and post-release survival in translocated North Island saddlebacks ( Philesturnus rufusater ) in New Zealand . Emu 110 : 296–301. [ Google Scholar ]
- Aldridge RD, Arackal AA (2005) Reproductive biology and stress of captivity in male brown treesnakes ( Boiga irregularis ) on Guam . Aust J Zool 53 : 249–256. [ Google Scholar ]
- Baker ML, Gemmell E, Gemmell RT (1998) Physiological changes in brushtail possums, Trichosurus vulpecula , transferred from the wild to captivity . J Exp Zool 280 : 203–212. [ PubMed ] [ Google Scholar ]
- Barry M, Cockrem JF, Brunton DH (2010) Seasonal variation in plasma corticosterone concentrations in wild and captive adult Duvaucel's geckos ( Hoplodactylus duvaucelii ) in New Zealand . Aust J Zool 58 : 234–242. [ Google Scholar ]
- Batson WG, Gordon IJ, Fletcher DB, Portas TJ, Manning AD (2017) The effect of pre-release captivity on the stress physiologyof a reintroduced population of wild eastern bettongs . J Zool 303 : 311–319. [ Google Scholar ]
- Begg D, Kemp R, Griffin F (2004) Normal levels of immunocompetence in possums ( Trichosurus vulpecula ) exposed to different laboratory housing conditions post capture . Immunol Cell Biol 82 : 253–256. [ PubMed ] [ Google Scholar ]
- Ben-David M, Blundell GM, Blake JE (2002) Post-release survival of river otters: effects of exposure to crude oil and captivity . J Wildlife Manage 66 : 1208–1223. [ Google Scholar ]
- Berner NJ, Heil LA, Romero LM (2013) Seasonal variation in corticosterone in free-living and captive eastern red-spotted newts Notophthalmus viridescens viridescens . J Herpetol 47 : 466–470. [ Google Scholar ]
- Bisson IA, Butler LK, Hayden TJ, Romero LM, Wikelski MC (2009) No energetic cost of anthropogenic disturbance in a songbird . Proc R Soc B Biol Sci 276 : 961–969. [ PMC free article ] [ PubMed ] [ Google Scholar ]
- Bolasina SN. (2011) Stress response of juvenile flounder ( Paralichthys orbignyanus , Valenciennes 1839), to acute and chronic stressors . Aquaculture 313 : 140–143. [ Google Scholar ]
- Bonacic C, Macdonald DW (2003) The physiological impact of wool-harvesting procedures in vicunas ( Vicugna vicugna ) . Anim Welf 12 : 387–402. [ Google Scholar ]
- Bosson CO, Palme R, Boonstra R (2009) Assessment of the stress response in Columbian ground squirrels: laboratory and field validation of an enzyme immunoassay for fecal cortisol metabolites . Physiol Biochem Zool 82 : 291–301. [ PubMed ] [ Google Scholar ]
- Bourke RE, Brock J, Nakamura RM (1987) A study of delayed capture mortality syndrome in skipjack tuna, Katsuwonus pelamis (1) . J Fish Dis 10 : 275–287. [ Google Scholar ]
- Breuner CW, Jennings DH, Moore MC, Orchinik M (2000) Pharmacological adrenalectomy with mitotane . Gen Comp Endocrinol 120 : 27–34. [ PubMed ] [ Google Scholar ]
- Buehler DM, Piersma T, Tieleman BI (2008) Captive and free-living red knots Calidris canutus exhibit differences in non-induced immunity that suggest different immune strategies in different environments . J Avian Biol 39 : 560–566. [ Google Scholar ]
- Cabezas S, Blas J, Marchant TA, Moreno S (2007) Physiological stress levels predict survival probabilities in wild rabbits . Horm Behav 51 : 313–320. [ PubMed ] [ Google Scholar ]
- Calisi RM, Bentley GE (2009) Lab and field experiments: are they the same animal? Horm Behav 56 : 1–10. [ PubMed ] [ Google Scholar ]
- Calvete C, Angulo E, Estrada R, Moreno S, Villafuerte R (2005) Quarantine length and survival of translocated European wild rabbits . J Wildlife Manage 69 : 1063–1072. [ Google Scholar ]
- Clearwater SJPN. (1997) The response to capture and confinement stress of plasma cortisol, plasma sex steroids and vitellogenic oocytes in the marine teleost, red gurnard . J Fish Biol 50 : 429–441. [ Google Scholar ]
- Clubb R, Mason G (2003) Captivity effects on wide-ranging carnivores . Nature 425 : 473–474. [ PubMed ] [ Google Scholar ]
- Coburn S, Salman M, Rhyan J, Keefe T, McCollum M (2010) Comparison of endocrine response to stress between captive-raised and wild-caught bighorn sheep . J Wildlife Manage 74 : 532–538. [ Google Scholar ]
- Cyr NE, Romero LM (2008) Fecal glucocorticoid metabolites of experimentally stressed captive and free-living starlings: implications for conservation research . Gen Comp Endocrinol 158 : 20–28. [ PubMed ] [ Google Scholar ]
- Czekala NM, Hodges JK, Gause GE (1980) Lasley BL , Annual circulating testosterone levels in captive and free-ranging male armadillos ( Dasypus novemcinctus ) . J Reprod Fertil 59 : 199–204. [ PubMed ] [ Google Scholar ]
- Dale DC, Fauci AS, Guerry D, Wolff SM (1975) Comparison of agents producing a neutrophilic leukocytosis in man. Hydrocortisone, prednisone, endotoxin, and etiocholanolone . J Clin Investig 56 : 808–813. [ PMC free article ] [ PubMed ] [ Google Scholar ]
- Davidson GW, Thorarensen HT, Lokman M, Davie PS (1997) Stress of capture and captivity in kahawai Arripis trutta (Bloch and Schneider) (Perciformes: Arripidae) . Comp Biochem Physiol A 118 : 1405–1410. [ Google Scholar ]
- Davies S, Rodriguez NS, Sweazea KL, Deviche P (2013) The effect of acute stress and long-term corticosteroid administration on plasma metabolites in an urban and desert songbird . Physiol Biochem Zool 86 : 47–60. [ PubMed ] [ Google Scholar ]
- Davis AK, Maerz JC (2008) Comparison of hematological stress indicators in recently captured and captive paedomorphic mole salamanders, Ambystoma talpoideum . Copeia 2008:613–617. [ Google Scholar ]
- Davis AK, Maney DL, Maerz JC (2008) The use of leukocyte profiles to measure stress in vertebrates: a review for ecologists . Funct Ecol 22 : 760–772. [ Google Scholar ]
- Day TD, O'Connor CE (2000) Behavioural adaptation of brushtail possums ( Trichosurus vulpecula ) to captivity . Anim Welf 9 : 413–420. [ Google Scholar ]
- de Assis VR, Titon SCM, Barsotti AMG, Titon B, Gomes FR (2015) Effects of acute restraint stress, prolonged captivity stress and transdermal corticosterone application on Immunocompetence and plasma levels of corticosterone on the cururu toad ( Rhinella icterica ) . Plos One 10 . [ PMC free article ] [ PubMed ] [ Google Scholar ]
- Dhabhar FS. (2002) Stress-induced augmentation of immune function—the role of stress hormones, leukocyte trafficking, and cytokines . Brain Behav Immun 16 : 785–798. [ PubMed ] [ Google Scholar ]
- Dhabhar FS, McEwen BS (1997) Acute stress enhances while chronic stress suppresses cell-mediated immunity in vivo: a potential role for leukocyte trafficking . Brain Behav Immun 11 : 286–306. [ PubMed ] [ Google Scholar ]
- Dickens MJ, Bentley GE (2014) Stress, captivity, and reproduction in a wild bird species . Horm Behav 66 : 685–693. [ PubMed ] [ Google Scholar ]
- Dickens MJ, Delehanty DJ, Romero LM (2009a) Stress and translocation: alterations in the stress physiology of translocated birds . Proc R Soc Biol Sci Ser B 276 : 2051–2056. [ PMC free article ] [ PubMed ] [ Google Scholar ]
- Dickens MJ, Earle K, Romero LM (2009b) Initial transference of wild birds to captivity alters stress physiology . Gen Comp Endocrinol 160 : 76–83. [ PubMed ] [ Google Scholar ]
- Dickens MJ, Romero LM (2009) Wild European starlings ( Sturnus vulgaris ) adjust to captivity with sustained sympathetic nervous system drive and a reduced fight-or-flight response . Physiol Biochem Zool 82 : 603–610. [ PubMed ] [ Google Scholar ]
- Dickens MJ, Romero LM (2013) A consensus endocrine profile for chronically stressed wild animals does not exist . Gen Comp Endocrinol 191 : 177–189. [ PubMed ] [ Google Scholar ]
- Dufty AM Jr, Belthoff JR (1997) Corticosterone and the stress response in young western screech-owls: effects of captivity, gender, and activity period . Physiol Zool 70 : 143–149. [ PubMed ] [ Google Scholar ]
- Dufty AM Jr, Wingfield JC (1986) Temporal patterns of circulating luteinizing and steroid hormones in a brood parasite the brown-headed cowbird, Molothrus-Ater I. Males . J Zool Ser A 208 : 191–204. [ Google Scholar ]
- Evans JE, Smith EL, Bennett ATD, Cuthill IC, Buchanan KL (2012) Short-term physiological and behavioural effects of high- versus low-frequency fluorescent light on captive birds . Anim Behav 83 : 25–33. [ Google Scholar ]
- Ewenson EL, Zann RA, Flannery GR (2001) Body condition and immune response in wild zebra finches: effects of capture, confinement and captive-rearing . Naturwissenschaften 88 : 391–394. [ PubMed ] [ Google Scholar ]
- Fajardo I, Babiloni G, Miranda Y (2000) Rehabilitated and wild barn owls ( Tyto alba ): dispersal, life expectancy and mortality in Spain . Biol Conserv 94 : 287–295. [ Google Scholar ]
- Fanson KV, Wielebnowski NC, Shenk TM, Lucas JR (2012) Comparative patterns of adrenal activity in captive and wild Canada lynx ( Lynx canadensis ) . J Comp Physiol B 182 : 157–165. [ PubMed ] [ Google Scholar ]
- Fernandez-Moran J, Saavedra D, De La JLR, Manteca-Vilanov X (2004) Stress in wild-caught Eurasian otters ( Lutra lutra ): effects of a long-acting neuroleptic and time in captivity . Anim Welf 13 : 143–149. [ Google Scholar ]
- Fischer CP, Rao R, Romero LM (2017) Exogenous and endogenous corticosterone in feathers . J Avian Biol 48 : 1301–1309. [ Google Scholar ]
- Fischer CP, Romero LM (2016) The use of α- or β-blockers to ameliorate the chronic stress of captivity in the house sparrow ( Passer domesticus ) . Conserv Physiol 4 (1):cow049; doi:10.1093/conphys/cow049. [ PMC free article ] [ PubMed ] [ Google Scholar ]
- Fischer CP, Wright-Lichter J, Romero LM (2018) Chronic stress and the introduction to captivity: how wild house sparrows ( Passer domesticus ) adjust to laboratory conditions . Gen Comp Endocrinol 259 : 85–92. [ PubMed ] [ Google Scholar ]
- Flugge G. (1996) Alterations in the central nervous alpha(2)-adrenoceptor system under chronic psychosocial stress . Neuroscience 75 : 187–196. [ PubMed ] [ Google Scholar ]
- Fokidis HB, Hurley L, Rogowski C, Sweazea K, Deviche P (2011) Effects of captivity and body condition on plasma corticosterone, locomotor behavior, and plasma metabolites in curve-billed thrashers . Physiol Biochem Zool 84 : 595–606. [ PubMed ] [ Google Scholar ]
- Franceschini MD, Rubenstein DI, Low B, Romero LM (2008) Fecal glucocorticoid metabolites as an indicator of stress during translocation and acclimation in an endangered large mammal, the Grevy’s zebra . Anim Conserv 11 : 263–269. [ Google Scholar ]
- Franzman AWT, Thorne ET (1970) Physiologic values in wild bighorn sheep ( Ovis canadensis canadensis ) at capture, after handling, and after captivity . J Am Vet Med Assoc 157 : 647–650. [ PubMed ] [ Google Scholar ]
- Gardiner KJ, Hall AJ (1997) Diel and annual variation in plasma cortisol concentrations among wild and captive harbor seals ( Phoca vitulina ) . Can J Zool 75 : 1773–1780. [ Google Scholar ]
- Gobbetti A, Zerani M (1996) Possible mechanism for the first response to short captivity stress in the water frog, Rana esculenta . J Endocrinol 148 : 233–239. [ PubMed ] [ Google Scholar ]
- Gormally BMG, Fuller R, McVey M, Romero LM (2019) DNA damage as an indicator of chronic stress: correlations with corticosterone and uric acid . Comp Biochem Physiol A Mol Integr Physiol 227 : 116–122. [ PubMed ] [ Google Scholar ]
- Greenwood VJ, Smith EL, Goldsmith AR, Cuthill IC, Crisp LH, Walter-Swan MB, Bennett ATD (2004) Does the flicker frequency of fluorescent lighting affect the welfare of captive European starlings? Appl Anim Behav Sci 86 : 145–159. [ Google Scholar ]
- Gross WB, Siegel HS (1983) Evaluation of the heterophil/lymphocyte ratio as a measure of stress in chickens . Avian Dis 27 : 972–979. [ PubMed ] [ Google Scholar ]
- Grutter AS, Pankhurst NW (2000) The effects of capture, handling, confinement and ectoparasite load on plasma levels of cortisol, glucose and lactate in the coral reef fish Hemigymnus melapterus . J Fish Biol 57 : 391–401. [ Google Scholar ]
- Hamalainen A, Heistermann M, Fenosoa ZS, Kraus C (2014) Evaluating capture stress in wild gray mouse lemurs via repeated fecal sampling: method validation and the influence of prior experience and handling protocols on stress responses . Gen Comp Endocrinol 195 : 68–79. [ PubMed ] [ Google Scholar ]
- Hare JF, Ryan CP, Enright C, Gardiner LE, Skyner LJ, Berkvens CN, Anderson WG (2014) Validation of a radioimmunoassay-based fecal corticosteroid assay for Richardson's ground squirrels Urocitellus richardsonii and behavioural correlates of stress . Curr Zool 60 : 591–601. [ Google Scholar ]
- Herrera M, Rodiles A, Sanchez B, Lopez JM, de La E (2016) Physiological stress responses to captivity in early developmental stages of the wedge sole Dicologoglossa cuneata (Moreau) . Aquacult Res 47 : 732–740. [ Google Scholar ]
- Hoffman AM, Leighton FA (1985) Hemograms and microscopic lesions of herring-gulls during captivity . J Am Vet Med Assoc 187 : 1125–1128. [ PubMed ] [ Google Scholar ]
- Holz P, Barnett JEF (1996) Long-acting tranquilizers: their use as a management tool in the confinement of free-ranging red-necked wallabies ( Macropus rufogriseus ) . J Zoo Wildl Med 27 : 54–60. [ Google Scholar ]
- Imanaga Y, Nyuji M, Amano M, Takahashi A, Kitano H, Yamaguchi A, Matsuyama M (2014) Characterization of gonadotropin-releasing hormone and gonadotropin in jack mackerel ( Trachurus japonicus ): comparative gene expression analysis with respect to reproductive dysfunction in captive and wild fish . Aquaculture 428 : 226–235. [ Google Scholar ]
- Jepsen EM, Ganswindt A, Ngcamphalala CA, Bourne AR, Ridley AR, McKechnie AE (2019) Non-invasive monitoring of physiological stress in an afrotropical arid-zone passerine bird, the southern pied babbler . Gen Comp Endocrinol 276 : 60–68. [ PubMed ] [ Google Scholar ]
- Johnstone CP, Reina RD, Lill A (2012) Interpreting indices of physiological stress in free-living vertebrates . J Comp Physiol B 182 : 861–879. [ PubMed ] [ Google Scholar ]
- Jones SM, Bell K (2004) Plasma corticosterone concentrations in males of the skink Egernia whitii during acute and chronic confinement, and over a diel period . Comp Biochem Physiol 137A : 105–113. [ PubMed ] [ Google Scholar ]
- Kagira JM, Ngotho M, Thuita JK, Maina NW, Hau J (2007) Hernatological changes in vervet monkeys ( Chlorocebus aethiops ) during eight months' adaptation to captivity . Am J Primatol 69 : 1053–1063. [ PubMed ] [ Google Scholar ]
- Knox CM, Hattingh J, Raath JP (1990) The effect of tranquilizers on the immediate responses to repeated capture and handling of boma-kept impala . Comp Biochem Physiol C 95 : 247–251. [ Google Scholar ]
- Kock RA, Mihok SRO, Wambua J, Mwanzia J, Saigawa K (1999) Effects of translocation on hematologic parameters of free-ranging black rhinoceros ( Diceros bicornis michaeli ) in Kenya . J Zoo Wildl Med 30 : 389–396. [ PubMed ] [ Google Scholar ]
- Krams I, Vrublevska J, Cirule D, Kivleniece I, Krama T, Rantala MJ, Kaasik A, Horak P, Sepp T (2013) Stress, behaviour and immunity in wild-caught wintering great tits ( Parus major ) . Ethology 119 : 397–406. [ Google Scholar ]
- Kuhlman JR, Martin LB (2010) Captivity affects immune redistribution to skin in a wild bird . Funct Ecol 24 : 830–837. [ Google Scholar ]
- LaDage LD, Roth TC, Fox RA, Pravosudov VV (2009) Effects of captivity and memory-based experiences on the hippocampus in mountain chickadees . Behav Neurosci 123 : 284–291. [ PubMed ] [ Google Scholar ]
- Landsman RE. (1993) The effects of captivity on the electric organ discharge and plasma-hormone levels in Gnathonemus petersii (Mormyriformes) . J Comp Physiol A 172 : 619–631. [ PubMed ] [ Google Scholar ]
- Larcombe SD, Tregaskes CA, Coffey J, Stevenson AE, Alexander LG, Arnold KE (2015) Oxidative stress, activity behaviour and body mass in captive parrots . Conserv Physiol 3 . [ PMC free article ] [ PubMed ] [ Google Scholar ]
- Lattin CR, Bauer CM, de R, Romero LM (2012) Hypothalamus-pituitary-adrenal axis activity and the subsequent response to chronic stress differ depending upon life history stage . Gen Comp Endocrinol 178 : 494–501. [ PubMed ] [ Google Scholar ]
- Lattin CR, Romero LM (2014) Chronic exposure to a low dose of ingested petroleum disrupts corticosterone receptor signalling in a tissue-specific manner in the house sparrow ( Passer domesticus ) . Conserv Physiol 2 : cou058. [ PMC free article ] [ PubMed ] [ Google Scholar ]
- Laubscher LL, Hoffman LC, Pitts NI, Raath JP (2016) The effect of a slow-release formulation of zuclopenthixol acetate (Acunil (R)) on captive blue wildebeest ( Connochaetes taurinus ) behavior and physiological response . J Zoo Wildl Med 47 : 514–522. [ PubMed ] [ Google Scholar ]
- Lepeigneul O, Ballouard JM, Bonnet X, Beck E, Barbier M, Ekori A, Buisson E, Caron S (2014) Immediate response to translocation without acclimation from captivity to the wild in Hermann's tortoise . Eur J Wildlife Res 60 : 897–907. [ Google Scholar ]
- Levin M, Leibrecht H, Mori C, Jessup D, De S (2007) Immunomodulatory effects of organochlorine mixtures upon in vitro exposure of peripheral blood leukocytes differ between free-ranging and captive southern sea otters ( Enhydra lutris ) . Vet Immunol Immunopathol 119 : 269–277. [ PubMed ] [ Google Scholar ]
- Lilly AA, Mehlman PT, Higley JD (1999) Trait-like immunological and hematological measures in female rhesus across varied environmental conditions . Am J Primatol 48 : 197–223. [ PubMed ] [ Google Scholar ]
- Linklater WL, MacDonald EA, Flamand JRB, Czekala NM (2010) Declining and low fecal corticoids are associated with distress, not acclimation to stress, during the translocation of African rhinoceros . Anim Conserv 13 : 104–111. [ Google Scholar ]
- Lombardo MP, Thorpe PA (2009) Captivity affects sperm production, testes size and beak color in house sparrows ( Passer domesticus ) . Int Stud Sparrows 33 : 5–16. [ Google Scholar ]
- Love AC, Lovern MB, DuRant SE (2017) Captivity influences immune responses, stress endocrinology, and organ size in house sparrows ( Passer domesticus ) . Gen Comp Endocrinol 252 : 18–26. [ PubMed ] [ Google Scholar ]
- Maddocks SA, Goldsmith AR, Cuthill IC (2002) Behavioural and physiological effects of absence of ultraviolet wavelengths on European starlings Sturnus vulgaris . J Avian Biol 33 : 103–106. [ Google Scholar ]
- Mahmoud IY, Guillette LJ, McAsey ME, Cady C (1989) Stress-induced changes in serum testosterone, estradiol-17β and progesterone in the turtle, Chelydra serpentina . Comp Biochem Physiol A 93 : 423–427. [ Google Scholar ]
- Marcalo A, Pousao-Ferreira P, Mateus L, Correia JHD, Stratoudakis Y (2008) Sardine early survival, physical condition and stress after introduction to captivity . J Fish Biol 72 : 103–120. [ Google Scholar ]
- Marra PP, Lampe KT, Tedford BL (1995) Plasma corticosterone levels in two species of Zonotrichia sparrows under captive and free-living conditions . Wilson Bull 107 : 296–305. [ Google Scholar ]
- Martin LB. (2009) Stress and immunity in wild vertebrates: timing is everything . Gen Comp Endocrinol 163 : 70–76. [ PubMed ] [ Google Scholar ]
- Martin LB, Kidd L, Liebl AL, Coon CAC (2011) Captivity induces hyper-inflammation in the house sparrow ( Passer domesticus ) . J Exp Biol 214 : 2579–2585. [ PubMed ] [ Google Scholar ]
- Mason G, Clubb R, Latham N, Vickery S (2007) Why and how should we use environmental enrichment to tackle stereotypic behaviour? Appl Anim Behav Sci 102 : 163–188. [ Google Scholar ]
- Mason GJ. (2010) Species differences in responses to captivity: stress, welfare and the comparative method . Trends Ecol Evol 25 : 713–721. [ PubMed ] [ Google Scholar ]
- Mathies T, Felix TA, Lance VA (2001) Effects of trapping and subsequent short-term confinement stress on plasma corticosterone in the brown treesnake ( Boiga irregularis ) on Guam . Gen Comp Endocrinol 124 : 106–114. [ PubMed ] [ Google Scholar ]
- McCormick GL, Shea K, Langkilde T (2015) How do duration, frequency, and intensity of exogenous CORT elevation affect immune outcomes of stress? Gen Comp Endocrinol 222 : 81–87. [ PubMed ] [ Google Scholar ]
- McLeod BJ, Thompson EG, Crawford JL, Shackell GH (1997) Successful group housing of wild-caught brushtail possums ( Trichosurus vulpecula ) . Anim Welf 6 : 67–76. [ Google Scholar ]
- McPhee ME, Carlstead K (2010) The importance of maintaining natural behaviors in captive mammals In Kleiman DG, Thompson KV, Baer CK, eds, Wild Mammals in Captivity: Principles and Techniques for Zoo Management . University of Chicago Press, Chicago, pp. 303–313. [ Google Scholar ]
- Mellish JE, Calkins DG, Christen DR, Horning M, Rea LD, Atkinson SK (2006) Temporary captivity as a research tool: comprehensive study of wild pinnipeds under controlled conditions . Aquat Mamm 32 : 58–65. [ Google Scholar ]
- Miller NW, Tripp MR (1982) The effect of captivity on the immune response of the killifish, Fundulus heteroclitus L . J Fish Biol 20 : 301–308. [ Google Scholar ]
- Millet S, Bennett J, Lee KA, Hau M, Klasing KC (2007) Quantifying and comparing constitutive immunity across avian species . Dev Comp Immunol 31 : 188–201. [ PubMed ] [ Google Scholar ]
- Molony SE, Dowding CV, Baker PJ, Cuthill IC, Harris S (2006) The effect of translocation and temporary captivity on wildlife rehabilitation success: an experimental study using European hedgehogs ( Erinaceus europaeus ) . Biol Conserv 130 : 530–537. [ Google Scholar ]
- Moore MC, Thompson CW, Marler CA (1991) Reciprocal changes in corticosterone and testosterone levels following acute and chronic handling stress in the tree lizard, Urosaurus ornatus . Gen Comp Endocrinol 81 : 217–226. [ PubMed ] [ Google Scholar ]
- Moorhouse TP, Gelling M, McLaren GW, Mian R, Macdonald DW (2007) Physiological consequences of captive conditions in water voles ( Arvicola terrestris ) . J Zool 271 : 19–26. [ Google Scholar ]
- Morales MH, Sanchez EJ (1996) Changes in vitellogenin expression during captivity-induced stress in a tropical anole . Gen Comp Endocrinol 103 : 209–219. [ PubMed ] [ Google Scholar ]
- Morgan KN, Tromborg CT (2007) Sources of stress in captivity . Appl Anim Behav Sci 102 : 262–302. [ Google Scholar ]
- Narayan E, Hero JM (2011) Urinary corticosterone responses and haematological stress indicators in the endangered Fijian ground frog ( Platymantis vitiana ) during transportation and captivity . Aust J Zool 59 : 79–85. [ Google Scholar ]
- Narayan EJ, Cockrem JF, Hero JM (2011) Urinary corticosterone metabolite responses to capture and captivity in the cane toad ( Rhinella marina ) . Gen Comp Endocrinol 173 : 371–377. [ PubMed ] [ Google Scholar ]
- Narayan EJ, Cockrem JF, Hero JM (2012) Effects of temperature on urinary corticosterone metabolite responses to short-term capture and handling stress in the cane toad ( Rhinella marina ) . Gen Comp Endocrinol 178 : 301–305. [ PubMed ] [ Google Scholar ]
- Nester Bolasina S. (2011) Stress response of juvenile flounder ( Paralichthys orbignyanus , Valenciennes 1839), to acute and chronic stressors . Aquaculture 313 : 140–143. [ Google Scholar ]
- Nikoo M, Falahatkar B, Alekhorshid M, Haghi BN, Asadollahpour A, Dangsareki MZ, Langroudi HF (2010) Physiological stress responses in kutum Rutilus frisii kutum subjected to captivity . Int Aquat Res 2 : 55–60. [ Google Scholar ]
- Olsen DE, Seabloom RW (1973) Adrenocortical response to captivity in Microtus pennsylvanicus . J Mammal 54 : 779–781. [ PubMed ] [ Google Scholar ]
- Orlov MM, Mukhlya AM, Postoyanova NI, Kazanova NV (1991) Biochemical adaptation of cetaceans in captivity . J Evol Biochem Physiol 27 : 331–336. [ Google Scholar ]
- Pankhurst NW, Sharples DF (1992) Effects of capture and confinement on plasma cortisol concentrations in the snapper, Pagrus auratus . Aust J Mar Freshw Res 43 : 345–356. [ Google Scholar ]
- Patton KT, Croonquist MJ, Crawford WC (1985) Management-related stress in the red-shouldered hawk . Zoo Biol 4 : 235–243. [ Google Scholar ]
- Peinado VI, Fernandezarias A, Zabala JL, Palomeque J (1995) Effect of captivity on the blood composition of Spanish ibex ( Capra pyrenaica hispanica ) . Vet Rec 137 : 588–591. [ PubMed ] [ Google Scholar ]
- Perret M. (1982) Influence of captivity and social-groups on lesser mouse lemur physiology.1. Stress-effects in Microcebus murinus . Folia Primatol 39 : 63–114. [ PubMed ] [ Google Scholar ]
- Peters G, Delventhal H, Klinger H (1980) Physiological and morphological effects of social stress in the eel, ( Anguilla anguilla L) . Arch Fischereiwiss 302 : 157–180. [ Google Scholar ]
- Piersma T, Koolhaas A, Dekinga A, Gwinner E (2000) Red blood cell and white blood cell counts in sandpipers ( Philomachus pugnax, Calidris canutus ): effects of captivity, season, nutritional status, and frequent bleedings . Can J Zool 78 : 1349–1355. [ Google Scholar ]
- Piersma T, Ramenofsky M (1998) Long-term decreases of corticosterone in captive migrant shorebirds that maintain seasonal mass and moult cycles . J Avian Biol 29 : 97–104. [ Google Scholar ]
- Plante S, Audet C, Lambert Y, de la J (2003) Comparison of stress responses in wild and captive winter flounder ( Pseudopleuronectes americanus Walbaum) broodstock . Aquacult Res 34 : 803–812. [ Google Scholar ]
- Quispe R, Villavicencio CP, Addis E, Wingfield JC, Vasquez RA (2014) Seasonal variations of basal cortisol and high stress response to captivity in Octodon degus , a mammalian model species . Gen Comp Endocrinol 197 : 65–72. [ PubMed ] [ Google Scholar ]
- Rangel-Negrin A, Alfaro JL, Valdez RA, Romano MC, Serio-Silva JC (2009) Stress in Yucatan spider monkeys: effects of environmental conditions on fecal cortisol levels in wild and captive populations . Anim Conserv 12 : 496–502. [ Google Scholar ]
- Rich EL, Romero LM (2005) Exposure to chronic stress downregulates corticosterone responses to acute stressors . Am J Physiol Regul Integr Comp Physiol 288 : R1628–R1636. [ PubMed ] [ Google Scholar ]
- Rideout BA, Gause GE, Benirschke K, Lasley BL (1985) Stress-induced adrenal changes and their relation to reproductive failure in captive 9-banded armadillos ( Dasypus novemcinctus ) . Zoo Biol 4 : 129–137. [ Google Scholar ]
- Romero LM, Dickens MJ, Cyr NE (2009) The reactive scope model—a new model integrating homeostasis, allostasis, and stress . Horm Behav 55 : 375–389. [ PubMed ] [ Google Scholar ]
- Romero LM, Reed JM (2005) Collecting baseline corticosterone samples in the field: is under three minutes good enough? Comp Biochem Physiol Part A Mol Integr Physiol 140 : 73–79. [ PubMed ] [ Google Scholar ]
- Romero LM, Wingfield JC (1999) Alterations in hypothalamic-pituitary-adrenal function associated with captivity in Gambel's white-crowned sparrows ( Zonotrichia leucophrys gambelii ) . Comp Biochem Physiol 122B : 13–20. [ PubMed ] [ Google Scholar ]
- Romero LM, Wingfield JC (2016) Tempests, Poxes, Predators, and People: Stress in Wild Animals and How They Cope . Oxford University Press, New York [ Google Scholar ]
- Ruiz G, Rosenmann M, Novoa FF, Sabat P (2002) Hematological parameters and stress index in rufous-collared sparrows dwelling in urban environments . Condor 104 : 162–166. [ Google Scholar ]
- Sanchez-Sarmiento AM, Zwarg T, Fernandes-Santos RC, Guimaraes-Luiz T, Genoy-Puerto A, Matushima ER (2015) Hematological parameters and the variations resulting from stress of Alouatta caraya during a wildlife rescue program in Brazil . Am J Primatol 77 : 246–253. [ PubMed ] [ Google Scholar ]
- Sanderson JT. (2006) The steroid hormone biosynthesis pathway as a target for endocrine-disrupting chemicals . Toxicol Sci 94 : 3–21. [ PubMed ] [ Google Scholar ]
- Sapolsky RM, Romero LM, Munck AU (2000) How do glucocorticoids influence stress-responses? Integrating permissive, suppressive, stimulatory, and preparative actions . Endocr Rev 21 : 55–89. [ PubMed ] [ Google Scholar ]
- Sepp T, Sild E, Horak P (2010) Hematological condition indexes in greenfinches: effects of captivity and diurnal variation . Physiol Biochem Zool 83 : 276–282. [ PubMed ] [ Google Scholar ]
- Sheriff MJ, Dantzer B, Delehanty B, Palme R, Boonstra R (2011) Measuring stress in wildlife: techniques for quantifying glucocorticoids . Oecologia 166 : 869–887. [ PubMed ] [ Google Scholar ]
- Shuert C, Horning M, Mellish JA (2015) The effect of novel research activities on long-term survival of temporarily captive Steller Sea lions ( Eumetopias jubatus ) . Plos One 10 :e0141948. [ PMC free article ] [ PubMed ] [ Google Scholar ]
- Siebert U, Pozniak B, Hansen KA, Nordstrom G, Teilmann J, van N, Vossen A, Dietz R (2011) Investigations of thyroid and stress hormones in free-ranging and Captive Harbor porpoises ( Phocoena phocoena ): a pilot study . Aquat Mamm 37 : 443–453. [ Google Scholar ]
- Smith MFL. (1992) Capture and transportation of elasmobranchs, with emphasis on the gray nurse shark ( Carcharias taurus ) . Aust J Mar Freshwater Res 43 : 325–343. [ Google Scholar ]
- Sparkman AM, Bronikowski AM, Williams S, Parsai S, Manhart W, Palacios MG (2014) Physiological indices of stress in wild and captive garter snakes: correlations, repeatability, and ecological variation . Comp Biochem Physiol A Mol Integr Physiol 174 : 11–17. [ PubMed ] [ Google Scholar ]
- St Aubin DJ, Geraci JR (1988) Capture and handling stress suppresses circulating levels of thyroxine T4 and triiodothyronine T3 in beluga whales Delphinapterus leucas . Physiol Zool 61 : 170–175. [ Google Scholar ]
- St Aubin DJ, Geraci JR (1989) Adaptive changes in hematologic and plasma chemical constituents in captive beluga whales, Delphinapterus leucas . Can J Fish Aquat Sci 46 : 796–803. [ Google Scholar ]
- Stead-Richardson E, Bradshaw D, Friend T, Fletcher T (2010) Monitoring reproduction in the critically endangered marsupial, Gilbert's potoroo ( Potorous gilbertii ): preliminary analysis of faecal oestradiol-17 beta, cortisol and progestagens . Gen Comp Endocrinol 165 : 155–162. [ PubMed ] [ Google Scholar ]
- Steyn DG. (1975) The effects of captivity stress on the blood chemical values of the chacma baboon Papio ursinus . Lab Anim 9 : 111–120. [ PubMed ] [ Google Scholar ]
- Suleman MA, Wango E, Sapolsky RM, Odongo H, Hau J (2004) Physiologic manifestations of stress from capture and restraint of free-ranging male african green monkeys ( Cercopithecus aethiops ) . J Zoo Wildl Med 35 : 20–24. [ PubMed ] [ Google Scholar ]
- Sykes KL, Klukowski M (2009) Effects of acute temperature change, confinement and housing on plasma Corticosterone in water snakes, Nerodia sipedon (Colubridae: Natricinae) . J Exp Zool A 311A : 172–181. [ PubMed ] [ Google Scholar ]
- Tarr BA, Rabinowitz JS, Imtiaz MA, DeVoogd TJ (2009) Captivity reduces hippocampal volume but not survival of new cells in a food-storing bird . Dev Neurobiol 69 : 972–981. [ PMC free article ] [ PubMed ] [ Google Scholar ]
- Titon SCM, Assis VR, Titon B, Cassettari BD, Fernandes P, Gomes FR (2017) Captivity effects on immune response and steroid plasma levels of a Brazilian toad ( Rhinella schneideri ) . J Exp Zool A 327 : 127–138. [ PubMed ] [ Google Scholar ]
- Titon SCM, Titon Junior B, Assis VR, Kinker GS, Fernandes PACM, Gomes FR (2018) Interplay among steroids, body condition and immunity in response to long-term captivity in toads . Sci Rep 8 : 17168. [ PMC free article ] [ PubMed ] [ Google Scholar ]
- Tolhurst B, Grogan A, Hughes H, Scott D (2016) Effects of temporary captivity on ranging behaviour in urban red foxes ( Vulpes vulpes ) . Appl Anim Behav Sci 181 : 182–190. [ Google Scholar ]
- Trumble SJ, O'Neil D, Cornick LA, Gulland FMD, Castellini MA, Atkinson S (2013) Endocrine changes in harbor seal ( Phoca vitulina ) pups undergoing rehabilitation . Zoo Biol 32 : 134–141. [ PubMed ] [ Google Scholar ]
- Tyrrell C, Cree A (1994) Plasma corticosterone concentrations in wild and captive juvenile tuatara ( Sphenodon punctatus ) . New Zeal J Zool 21 : 407–416. [ Google Scholar ]
- Van der Weyd LK, Martin GB, Paris MCJ (2016) Monitoring stress in captive and free-ranging African wild dogs ( Lycaon pictus ) using faecal glucocorticoid metabolites . Gen Comp Endocrinol 226 : 50–55. [ PubMed ] [ Google Scholar ]
- Vera F, Antenucci CD, Zenuto RR (2011) Cortisol and corticosterone exhibit different seasonal variation and responses to acute stress and captivity in tuco-tucos ( Ctenomys talarum ) . Gen Comp Endocrinol 170 : 550–557. [ PubMed ] [ Google Scholar ]
- Vitousek MN, Taff CC, Ryan TA, Zimmer C (2019) Stress resilience and the dynamic regulation of glucocorticoids . Integr Comp Biol . [ PubMed ] [ Google Scholar ]
- Wasser SK, Hunt KE, Brown JL, Cooper K, Crockett CM, Bechert U, Millspaugh JJ, Larson S, Monfort SL (2000) A generalized fecal glucocorticoid assay for use in a diverse array of nondomestic mammalian and avian species . Gen Comp Endocrinol 120 : 260–275. [ PubMed ] [ Google Scholar ]
- Weiss SL, Jennings DH, Moore MC (2002) Effect of captivity in semi-natural enclosures on the reproductive endocrinology of female lizards . Gen Comp Endocrinol 128 : 238–246. [ PubMed ] [ Google Scholar ]
- Wernham C, Peach WJ, Browne SJ (1997) Survival rates of rehabilitated guillemots . British Trust for Ornithology. Thetford, Norfolk, UK.
- West DB, York B (1998) Dietary fat, genetic predisposition, and obesity: lessons from animal models . Am J Clin Nutr 67 : 505S–512S. [ PubMed ] [ Google Scholar ]
- Wingfield JC, Maney DL, Breuner CW, Jacobs JD, Lynn S, Ramenofsky M, Richardson RD (1998) Ecological bases of hormone-behavior interactions: the ‘emergency life history stage’ . Integr Comp Biol 38 : 191–206. [ Google Scholar ]
- Wingfield JC, Smith JP, Farner DS (1982) Endocrine responses of white-crowned sparrows to environmental stress . Condor 84 : 399–409. [ Google Scholar ]
- Zerani M, Amabili F, Mosconi G, Gobbetti A (1991) Effects of captivity stress on plasma steroid levels in the green frog, Rana esculenta , during the annual reproductive cycle . Comp Biochem Physiol 98A : 491–496. [ Google Scholar ]
- Share full article

Four Wild Ways to Save the Koala (That Just Might Work)
To protect Australia’s iconic animals, scientists are experimenting with vaccine implants, probiotics, tree-planting drones and solar-powered tracking tags.
A nurse in green scrubs holds an anesthetized koala above a medical table in a wildlife hospital. Credit...
Supported by

By Emily Anthes
Photographs and Video by Chang W. Lee
This story is part of a series on wildlife conservation in Australia, which Emily Anthes reported from Australia and New York.
- April 15, 2024
It was spring in Queensland, Australia, a season when many wild animals find themselves in trouble, and the Currumbin Wildlife Hospital was a blur of fur and feathers.
A groggy black swan emerged from the X-ray room, head swaying on its long neck. A flying fox wore a tiny anesthetic mask. An injured rainbow lorikeet squawked in its cage. (“Very angry,” a sign warned.)
“We see everything,” Dr. Michael Pyne, the hospital’s senior veterinarian. Also on the schedule for the day: three eagles, two carpet pythons, a blue-faced honeyeater, a short-eared brushtail possum and, Dr. Pyne said, “a whole heap of koalas.”
More than a dozen koalas were convalescing in open-air enclosures, wrapping their woolly arms around the trunks of eucalyptus trees. The wards were often full; in 2023, the hospital admitted more than 400 koalas, a fourfold increase from 2010.
The surge has been driven largely by the spread of chlamydia , a devastating bacterial infection. But the hospital was also seeing more koalas with traumatic injuries, including those caused by cars and dogs. Starving, dehydrated koalas came in during droughts; burned koalas appeared after wildfires. Occasionally, koalas even turned up with injuries caused by cows.
“That’s why they’re endangered,” Dr. Pyne said. “Everything’s against them.”

The koala, long an Australian icon, has become an unfortunate emblem of the country’s biodiversity crisis. The animals are threatened by deforestation, climate change and infectious disease. Together, these forces put the koala at the real risk of extinction . Although koalas are notoriously difficult to count, populations in some places have plummeted by as much as 80 percent , scientists estimate.
“We don’t know what the threshold is where there’s a point of no return,” said Tanya Pritchard, the senior manager for species recovery and landscape restoration at the World Wide Fund for Nature-Australia. “So we do need to act pretty urgently.”
Scientists and conservation groups are giving the koala everything they’ve got. Some are pursuing traditional time-tested strategies, including the protection of koala habitats and the advocacy of tougher conservation laws.
Others are trying more experimental approaches, from koala probiotics to tree-planting drones. Many of these projects are in the early stages, and none represent a complete solution. But given the wide array of threats that koalas are facing, saving them might require deploying every available tool.
“At this point,” Ms. Pritchard said, “every koala counts.”
Here are some of the tools in development.

Put shots in arms
Chlamydia, a common sexually transmitted infection in humans, is also widespread in the animal kingdom. How koalas were first infected is unknown, but one possibility is that the marsupials picked up chlamydia from the feces of livestock.
The disease, which can spread through sexual contact and from mothers to joeys, has become staggeringly widespread in parts of Australia. Chlamydia can cause urinary tract infections, blindness and infertility, suggesting that koalas could be in even worse shape than their declining numbers would indicate. “How many of those koalas that are out there can’t breed anymore because chlamydia has made them sterile?” Dr. Pyne said.
Scientists are now collaborating with the Currumbin Wildlife Hospital in Currumbin, Australia, to test a new chlamydia vaccine in wild koalas. So far, the vaccine is producing “quite spectacular results,” said Ken Beagley, an immunologist at the Queensland University of Technology who led the development of the vaccine.
Across two ongoing studies, more than 300 wild koalas have been vaccinated, and many vaccinated females have gone on to have healthy joeys, some of which have now had joeys of their own, Dr. Beagley said. “It was far better than we expected,” he said of the outcome.
Still, it will be challenging to inoculate thousands of wild koalas with the current vaccine, which requires two shots given 30 days apart. So Dr. Beagley and his colleagues are developing a delayed-release vaccine implant , which could be injected under the skin when a koala receives its first shot. Over the course of several weeks, the small capsule would slowly absorb water and then burst, thus delivering the second dose.
Give them the good microbes

Koalas are notoriously picky eaters with highly unusual tastes. “They feed on a really unpalatable diet of eucalyptus leaves, which is high in fiber, low in protein, high in toxins,” said Michaela Blyton, a molecular ecologist and microbiologist at the University of Queensland.
Living on eucalyptus requires a cooperative community of gut microbes, which help digest the leaves. Dr. Blyton’s work suggests that these microbial communities are so finely tuned that they may dictate which eucalyptus species, of the many that dot Australia, an individual koala can eat. That microbial specificity could explain why koalas are sometimes unable to diversify their diets, even in the face of starvation.
In a 2019 study, Dr. Blyton showed that she could shift koalas’ microbiomes, and expand their diets , by giving them fecal transplants from koalas that dined on a different type of eucalyptus. (To perform the transplant, Dr. Blyton packaged fecal samples from donor koalas in small capsules, which were administered orally.)
Now, she is hoping to use the same approach to maintain microbial equilibrium in koalas taking antibiotics, which are the frontline treatment for chlamydia. The drugs can throw the gut microbiome out of whack, prompting koalas to stop eating altogether, with sometimes fatal results. “It’s a hard ask to get the animal going again, and a lot of the time we just can’t,” said Dr. Blyton, who collaborates with Currumbin and other wildlife hospitals.
Dr. Blyton has developed a technique for freeze-drying fecal samples from healthy koalas, yielding shelf-stable capsules that can be given to koalas with chlamydia as a sort of oral probiotic. Unfortunately, early trial results suggested that administering the capsules was stressful for sick koalas. So Dr. Blyton is now trying to turn the freeze-dried fecal samples into a powder that could be added to other nutritional supplements the animals already receive.
Deploy the drones
Koalas — sedentary, tree-dwelling animals — are tricky to spot in the wild, adding to the challenges of tracking how their populations are faring, identifying critical habitats and safeguarding the animals from threats.
Grant Hamilton, a quantitative ecologist at the Queensland University of Technology, has developed a new koala-spotting system that is powered by artificial intelligence. A drone equipped with a thermal camera flies over the treetops, looking for pockets of body heat hidden under the canopy. Machine learning algorithms can quickly process this footage, tallying the koalas. The scientists then use statistical models to estimate the total koala population in a given area.
The scientists are now teaching local conservation groups how to fly the drones in their own neighborhoods. Dr. Hamilton and his colleagues will then analyze the data to help these organizations identify critical koala habitats that might benefit from protection or restoration. “We can use A.I. to help people to manage their backyards or their parks,” he said. “That’s a really exciting idea.”
The World Wide Fund for Nature-Australia, which is currently running a campaign to save or plant two billion trees by 2030, is experimenting with using drones for habitat restoration. Over the course of eight hours, a single tree-planting drone can rain some 40,000 seeds across the landscape.
Drones aren’t suited for all environments, but they offer a way to “scale up this work,” Ms. Pritchard said. “To me, it’s a little bit symbolic of our own plight,” she added. “If we can’t save the koala, as our most important and most loved species, what does that mean for our own situation and the health of our own habitats?”
Harness solar (and people) power
Despite the threats they face, koalas do have one thing going for them. “They are one of the cutest animals on Earth,” said Dr. Romane Cristescu, a conservation ecologist at the University of the Sunshine Coast.
To harness the public’s natural affection for koalas, she and her colleagues are developing a suite of technological tools, including solar-powered, location-tracking ear tags, which send data to a mobile app. The app, which is still undergoing testing, aims to help Australians get to know the koalas that live in their neighborhoods — “where they go, who they meet, their children, their boyfriend,” Dr. Cristescu said. “We’re going to tell people, ‘Hey, look, that koala’s got a life.’”
Dr. Cristescu hopes that people who develop attachments to their local koalas will be more inclined to support conservation efforts and change their own behaviors, like choosing not to cut down the trees in their yards. “We have a lot more empathy for a koala that has a name and a story,” she said.
The app also encourages users to log koala sightings and to report sick koalas, data that can be sent to scientists and wildlife care teams, she said.
The ear tags could be used for other purposes, too, said Dr. Cristescu, who also leads a research program that uses trained dogs to sniff out koalas and koala scat. After Australia’s catastrophic wildfires in 2019 and 2020, her team used dogs and drones to find and rescue injured koalas. The location-tracking ear tags could provide a quicker way to find koalas in danger, she said.

Emily Anthes is a science reporter, writing primarily about animal health and science. She also covered the coronavirus pandemic. More about Emily Anthes
Chang W. Lee has been a photographer for The Times for 30 years, covering events throughout the world. He is currently based in Seoul. Follow him on Instagram @nytchangster . More about Chang W. Lee
Explore the Animal Kingdom
A selection of quirky, intriguing and surprising discoveries about animal life..
To protect Australia’s iconic animals, scientists are experimenting with vaccine implants , probiotics, tree-planting drones and solar-powered tracking tags.
When traditional conservation fails, science is using “assisted evolution” to give vulnerable wildlife a chance , while posing the question whether we should change species to save them?
Two periodical cicada broods are appearing in a 16-state area in the Midwest and Southeast for the first time in centuries. Can you get rid of them? Do they bite? We answer your questions .
Aside from chimps and humans, researchers have found clear evidence of menopause in only five species — all of them whales. A new study looks at the possible causes for it .
Scientists never imagined that the blind cave salamanders called olms willingly left their caves. Then, they discovered several at aboveground springs in northern Italy .
According to a common narrative that male mammals tend to be larger than female ones. A new study paints a more complex picture .
Advertisement
- Skip to main content
- Keyboard shortcuts for audio player
Giving baby squirrels and other injured wildlife a second chance
Jacob Fenston

A volunteer at City Wildlife in Washington, D.C. feeds a baby squirrel with formula. The center helps rehabilitate animals that are injured or orphaned. Jacob Fenston/Jacob Fenston hide caption
A volunteer at City Wildlife in Washington, D.C. feeds a baby squirrel with formula. The center helps rehabilitate animals that are injured or orphaned.
In a nondescript warehouse in Washington, D.C., wedged between a community garden and the Metro train tracks, more than a dozen baby squirrels fill cages in an uncomfortably warm room. A noise machine pumps out forest sounds under the fluorescent lights.
Some squirrels nap in hammocks, cuddled up in furry piles. Others cling to the cages' wire, peering out.
Right now, it's baby squirrel season in much of the country. Spring is when many wild animals start having their offspring, which means it's a busy time for the people charged with rehabilitating animals that are injured or orphaned.
Animal care staffers here at the nonprofit City Wildlife spend much of their time these days hand-feeding baby squirrels.
"We could tell them apart because we paint their ears different colors," says Alessandra Flores, one of the staffers here. She reaches into a cage to grab a squirrel with ears painted pink. Then she holds the animal with one hand while offering it a tiny syringe with a soft nipple on the end, filled with specially formulated squirrel formula.
Some of the animals arrived here after falling from their nests, others were abandoned by mom. Others come in hit by cars.

Julie Edwards, a volunteer at City Wildlife in Washington, D.C., hand feeds one of the baby squirrels. Jacob Fenston/Jacob Fenston hide caption
Julie Edwards, a volunteer at City Wildlife in Washington, D.C., hand feeds one of the baby squirrels.
Squirrels are among the most common wild mammals in North America, so one might ask, why bother? It's not as if the eastern gray squirrel is going extinct any time soon.
"Some people say it's a waste of time on squirrels," says Jim Monsma, executive director of City Wildlife. But he says it's not just about wild animals — it's also about people. The act of saving a tiny, helpless squirrel, he says, generates compassion.
"It's not like you're born with a finite amount of compassion — once you use it up, that's it, you're a jerk. It's not like that. The more compassionate stuff you do, the more kind you become," Monsma says.

Terrance the octopus came to live with a family. Then she laid dozens of eggs
There are wildlife rehabilitation centers like this across the country. They take in all sorts of creatures in need, from turtles with broken shells to bald eagles with lead poisoning.
These centers are often powered by volunteers, like Julie Edwards. On this day, she's wrangling a rambunctious squirrel. His cage has a red label: "escape risk."
"They all have personalities," says Edwards, holding the squirrel. "This guy's a little crazy."
Edwards started volunteering at a rescue center in Houston, Texas. Her husband didn't want her to volunteer at the animal shelter there helping dogs and cats.
"He said I would take everything home," Edwards says. "But at the wildlife center, you can't take it home, so that was fine."
In the midst of the midday feeding session, a new patient comes in — the 16th baby squirrel currently at the center. He's all black and seems terrified, and maybe malnourished.
"I'm assuming someone found him on the sidewalk or something. He is pretty thin," says staffer Flores.
The goal is to get the squirrels healthy and big enough they can be released back into the wilds of the city and surrounding suburbs.

Across the world, migrating animal populations are dwindling. Here's why
Monsma says the vast majority of animals that come in are here because of how we've built our urban areas.
"We have changed our environment to make life very pleasant and efficient and comfortable for us," he says. Things like roads, cars, lawns, and lawnmowers make life easier for people, but much tougher for wild animals.
"Every day is an obstacle course with fatal consequences, potentially, for any slip up. So the victims pile up, and that's why we're here."

Research News
California sea otters nearly went extinct. now they're rescuing their coastal habitat.
As spring turns to summer, there will be even more victims: after baby squirrel season, the baby rabbits start coming in, then the ducklings, then mourning doves. By next fall, staff here will have rescued hundreds of urban animals.
If you find an injured or abandoned squirrel or other wild animal, you can find a local rehabilitator in your area, plus other information on rescuing wildlife, from the National Wildlife Rehabilitators Association .
- Frontiers in Veterinary Science
- Veterinary Epidemiology and Economics
- Research Topics
Reviews in Veterinary Epidemiology and Economics
Total Downloads
Total Views and Downloads
About this Research Topic
Infectious diseases impact the health of livestock, domestic animals, and wildlife and they also affect the life and health of humans. The growing importance of infectious diseases in animals is evidenced by the increased incidence and/or geographic range of some neglected or previously undetected diseases. ...
Keywords : diagnostics, prevention, treatment, epidemiology, ecology, zoonoses, One Health
Important Note : All contributions to this Research Topic must be within the scope of the section and journal to which they are submitted, as defined in their mission statements. Frontiers reserves the right to guide an out-of-scope manuscript to a more suitable section or journal at any stage of peer review.
Topic Editors
Topic coordinators, recent articles, submission deadlines, participating journals.
Manuscripts can be submitted to this Research Topic via the following journals:
total views
- Demographics
No records found
total views article views downloads topic views
Top countries
Top referring sites, about frontiers research topics.
With their unique mixes of varied contributions from Original Research to Review Articles, Research Topics unify the most influential researchers, the latest key findings and historical advances in a hot research area! Find out more on how to host your own Frontiers Research Topic or contribute to one as an author.

Why can zebrafish regenerate damaged heart tissue, while other fish species cannot?
A heart attack will leave a permanent scar on a human heart, yet other animals, including some fish and amphibians, can clear cardiac scar tissue and regrow damaged muscle as adults.
Scientists have sought to figure out how special power works in hopes of advancing medical treatments for human cardiac patients, but the great physiological differences between fish and mammals make such inquiries difficult.
So University of Utah biologists, led by assistant professor Jamie Gagnon, tackled the problem by comparing two fish species: zebrafish, which can regenerate its heart, and medaka, which cannot.
A tale of two fish
The team identified a few possible explanations, mostly associated with the immune system, for how zebrafish fix cardiac tissue, according to newly published research.
"We thought by comparing these two fish that have similar heart morphology and live in similar habitats, we could have a better chance of actually finding what the main differences are," said Clayton Carey, a postdoctoral researcher in the Gagnon lab and lead author on the new study.
Gagnon's team wasn't able to solve the mystery -- yet -- but their study shed new light on the molecular and cellular mechanisms at play in zebrafish's heart regeneration.
"It told us these two hearts that look very similar are actually very different," Gagnon said.
Both members of the teleost family of ray-finned fish, zebrafish ( Danio rerio ) and medaka ( Oryzias latipes ) descended from a common ancestor that lived millions of years ago. Both are about 1.5 inches long, inhabit freshwater and are equipped with two-chamber hearts. Medaka are native to Japan and zebrafish are native to the Ganges River basin.
According to the study, the existence of non-regenerating fish presents an opportunity to contrast the differing responses to injury to identify the cellular features unique to regenerating species. Gagnon suspects heart regeneration is an ancestral trait common to all teleosts.
Understanding the evolutionary path that led to the loss of this ability in some teleost species could offer parallel insights into why mammals cannot regenerate as adults.
With their distinctive horizontal stripes, zebrafish have long been popular as pets in the United States. In the 1970s zebrafish were embraced by biologists as a model organism for studying embryonic development of vertebrates.
Scientists like zebrafish because they can be propagated by the thousands quickly in labs, are easy to study and proved to be extremely hardy.
Cold shock to the heart
To conduct their experiments, the Gagnon lab used a device called a cryoprobe to injure the fish hearts in ways that mimic heart attacks in humans, then extracted the hearts after certain time frames to learn how the two species responded differently.
Carey made the cryoprobe from a piece of copper wire, which was cooled in liquid nitrogen to about minus 170 degrees Celsius. Team members cut tiny incisions in the fish's bellies to expose their hearts, then applied the probe for 23 seconds to the edge of the heart.
In 95% of the cases, the fish survived the procedure, although not for long. After three days or 14 days, their hearts were extracted and dissolved into a single-cell solution, which was then subjected to RNA sequencing in search of markers indicating how the fish responded to the injury.
"Zebrafish have this immune response that is typical of what you might see during a viral infection, called an interferon response," Carey said. "That response is completely absent in medaka."
The study documented differences in immune cell recruitment and behavior, epicardial and endothelial cell signaling, and alterations in the structure and makeup of the heart. For example, medaka lack a certain type of muscle cells that are present in zebrafish.
How zebrafish heal damaged cardiac tissue
"My hunch is the ancestor of all animals could regenerate its heart after an injury, and then that's been repeatedly lost in different types of animals," Gagnon said. "I would like to understand why. Why would you lose this great feature that allows you to regenerate your heart after an injury?"
The study indicates the zebrafish's ability to regenerate has something to do with its immune system, but understanding exactly how would take more research. For example, far more macrophages, specialized immune cells, migrated into the wound site in zebrafish than in medaka.
Unlike medaka, the zebrafish form a transient scar that doesn't calcify into rigid tissue.
"What you do with that scar is what matters," Gagnon said. "We think that the interferon response causes these specialized macrophage cells to come into that wound site and start to promote the growth of new blood vessels."
Over time new muscle replaces the damaged cardiac tissue and the heart heals.
"The more we learn about how animals can regenerate tissues, how those features have been lost in us and other animals, that's going to help us think about our limitations and how we might engineer strategies to help us overcome those," Gagnon said. "Our hope is that we build this knowledge base in animals that are really accessible and can be studied in incredible detail, then use that knowledge to generate more focused experiments in mammals, and then maybe someday in human patients."
- Heart Disease
- Cholesterol
- Immune System
- Wild Animals
- Rainforests
- Exotic Species
- Artificial heart
- Ischaemic heart disease
- Defibrillation
- Coronary heart disease
- Heart failure
Story Source:
Materials provided by University of Utah . Original written by Brian Maffly. Note: Content may be edited for style and length.
Journal Reference :
- Clayton M. Carey, Hailey L. Hollins, Alexis V. Schmid, James A. Gagnon. Distinct features of the regenerating heart uncovered through comparative single-cell profiling . Biology Open , 2024; 13 (4) DOI: 10.1242/bio.060156
Cite This Page :
Explore More
- Flare Erupting Around a Black Hole
- Two Species Interbreeding Created New Butterfly
- Warming Antarctic Deep-Sea and Sea Level Rise
- Octopus Inspires New Suction Mechanism for ...
- Cities Sinking: Urban Populations at Risk
- Puzzle Solved About Ancient Galaxy
- How 3D Printers Can Give Robots a Soft Touch
- Combo of Multiple Health Stressors Harming Bees
- Methane Emission On a Cold Brown Dwarf
- Remarkable Memories of Mountain Chickadees
Trending Topics
Strange & offbeat.

COMMENTS
1 INTRODUCTION. Research involving wild animals covers a wide range of species using different techniques and impacts individual animals and groups, up to the level of whole ecosystems (Sikes & Paul, 2013).Fieldwork may often be conducted in less than ideal conditions—in poor weather, non-sterile environments, areas exposed to climate extremes—and has the potential to harm the study ...
Research to advance understanding of the ecology and biology of wildlife species is more important than ever as the world confronts issues ranging from biodiversity loss to the emergence of zoonotic diseases. However, the current understanding of animal welfare in research and education has been based on laboratory work with specific domesticated species.
Wildlife Research. Wildlife research encompasses the study of ecology, management, and conservation involving wild mammals, fishes, amphibians, reptiles, birds, and invertebrates. When conducting this type of research, it's important for scientists to understand how it impacts wild animals, their environment, and their welfare.
Review our priorities for the field of wild animal welfare science. Papers. Download full texts of our published articles and preprints on our Library page. Research notes. See all Blog. Apr 8, 2022. ... Early-life experiences are a priority in wild animal welfare research. Aug 3, 2021.
Wild animal welfare science is understudied, novel, and uniquely challenging. Viewing wild systems through the lens of welfare raises new questions for researchers and wildlife managers. What are wild animals' lives like? How can we measure their welfare? What can we do to improve their lives? These questions guide our work.
Wildlife Letters is a new open access, rapid publication journal for peer-reviewed, scientific research articles about the ecology, conservation, and management of non-domesticated animals.Published work will be of broad interest and contain substantial findings about the conservation and management of wildlife species or understanding wildlife species responses to global change and ...
Wild animals are used in scientific research in a wide variety of contexts both in situ and ex situ. Guidelines for best practice, where they exist, are not always clearly linked to animal welfare and may instead have their origins in practicality. This is complicated by a lack of clarity about indicators of welfare for wild animals, and to ...
In recent decades, researchers have increasingly documented the impact of anthropogenic activities on wild animals, particularly in relation to changes in behaviour. However, whether human-induced ...
Wildbook, a project of the non-profit Wild Me, is an open-source software platform that blends structured wildlife research with artificial intelligence, community science, and computer vision to ...
Approaching wild animals to collect data on their phenotypic traits induces stress, escape behavior and, potentially, breeding failure 1,2,3 and therefore jeopardizes the quality of the collected ...
Core Concepts. This piece is part of our Core Concepts series, which introduces key topics in wild animal welfare. May 24, 2023. Wild animal welfare science focuses on understanding the subjective experiences of individual wild animals with the goal of improving their welfare. This multidisciplinary science dwells at the intersection of fields ...
Wild animals are used in scientific research in a wide variety of contexts both in situ and ex situ. Guidelines for best practice, where they exist, are not always clearly linked to animal welfare ...
The objectives are to (1) highlight commonalities between wild species and human fertility, (2) demonstrate that research in wild species-assisted reproductive technologies can greatly enhance success in human reproductive medicine, and (3) recognize that human fertility preservation is highly inspiring and relevant to wild species conservation.
While connections from basic research to applied wildlife conservation have been slow to develop, these connections are increasingly strengthening. ... A roadmap for high‐throughput sequencing studies of wild animal populations using noninvasive samples and hybridization capture. Molecular Ecology Resources, 19, 609-622. 10.1111/1755-0998. ...
Editorial on the Research Topic. Wildlife Welfare. Animal welfare relates to the feelings, behavior, and the health status of animals. Nevertheless, animal welfare legislation rarely prescribes what animals should feel or experience, but rather what humans should do to protect the animals in their care from unnecessary suffering, and e.g ...
Wildlife conservation is the practice of protecting plant and animal species and their habitats.Wildlife is integral to the world's ecosystems, providing balance and stability to nature's processes.The goal of wildlife conservation is to ensure the survival of these species, and to educate people on living sustainably with other species.
Scope. Wildlife Research represents an international forum for the publication of research and debate on the ecology, management and conservation of wild animals in natural and modified habitats. The journal combines basic research in wildlife ecology with advances in science-based management practice. Subject areas include: applied ecology ...
This Wild Animal Welfare Research Priorities document is intended to help researchers become aware of the most pressing questions impeding the ability to understand and improve the lives of wild animals. It also presents both the available methods for studying wild animal welfare and those that need to be developed or adapted.
The study, published in Nature Ecology and Evolution, involved more than 220 researchers, 163 mammal species and over 5,000 camera traps worldwide.Its findings revealed that wildlife react differently to human activity, depending on where the animals live and their position in the food web.
The main agency responsible for carrying out the act, the U.S. Fish and Wildlife Service, lists nearly 1,700 species endangered or threatened as of April 1. Nearly 1,400 species on the list have ...
Next, the Dragoi lab will combine foraging in a social context and record from two animals simultaneously while they cooperate to seek food as a reward. This is a daunting technical challenge but ...
Animals that are held in captivity for research might respond quite differently to a range of experimental treatments than a wild, free-living individual would. For example, environmental contaminants had different effects on wild and captive sea otters ( Levin et al ., 2007 ), and experimentally induced chronic stress caused a change in fecal ...
To protect Australia's iconic animals, scientists are experimenting with vaccine implants, probiotics, tree-planting drones and solar-powered tracking tags.
Things like roads, cars, lawns, and lawnmowers make life easier for people, but much tougher for wild animals. "Every day is an obstacle course with fatal consequences, potentially, for any slip up.
This includes governments and the research and advocacy communities that influence them. Our policy projects seek to incorporate wild animal welfare into institutional decision-making processes, benefiting wild animals either by changing policy in the short term or by normalizing wild animal welfare as a policy priority in the long term.
Infectious diseases impact the health of livestock, domestic animals, and wildlife and they also affect the life and health of humans. The growing importance of infectious diseases in animals is evidenced by the increased incidence and/or geographic range of some neglected or previously undetected diseases. Notably, this includes zoonotic diseases and those that have the potential to become ...
The aim of this article is to help advance this important research area by highlighting the central relationship between physiology and animal welfare and rectify its apparent oversight, as revealed by the current scientific literature on wild animals. Moreover, this article emphasizes the advantages of including physiological markers to assess ...
A heart attack will leave a permanent scar on a human heart, yet other animals, including zebrafish, can clear cardiac scar tissue and regrow damaged muscle as adults. Biologists sheds new light ...