Thank you for visiting nature.com. You are using a browser version with limited support for CSS. To obtain the best experience, we recommend you use a more up to date browser (or turn off compatibility mode in Internet Explorer). In the meantime, to ensure continued support, we are displaying the site without styles and JavaScript.
- View all journals

Coordination chemistry articles from across Nature Portfolio
Coordination chemistry is the study of compounds that have a central atom (often metallic) surrounded by molecules or anions, known as ligands. The ligands are attached to the central atom by dative bonds, also known as coordinate bonds, in which both electrons in the bond are supplied by the same atom on the ligand.
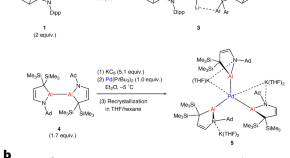
Palladium(0) complexes stabilized by anionic aluminium ligands
Palladium can be stabilized in the zero-oxidation state with one, two or three anionic aluminium ligands to produce mono-, di- or trianionic complexes, respectively. The palladium centres in the resulting complexes are highly negatively charged, and this electron richness enables the trianionic complex to undergo a hitherto hypothetical oxidative addition reaction with a diboron species.
Related Subjects
- Organometallic chemistry
Latest Research and Reviews
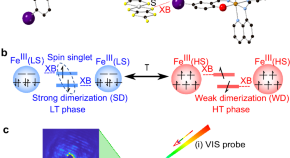
Direct observation of photoinduced sequential spin transition in a halogen-bonded hybrid system by complementary ultrafast optical and electron probes
Halogen bond is desired for tuning electronic properties by external stimuli. Here, authors reveal a sequential activation of spin crossover and dimer softening, unveiling a key transient state in a prototypical halogen-bonded material.
- Yifeng Jiang
- Stuart Hayes
- R. J. Dwayne Miller
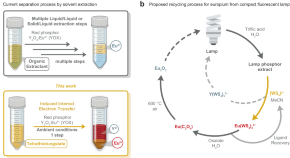
Recovery of europium from E-waste using redox active tetrathiotungstate ligands
Rare-earth elements (REEs) are vital to the modern economy, yet their mining poses both geopolitical and environmental challenges. Here, authors present a highly selective method for the recovery of europium from spent fluorescent lamps using redox active tetrathiotungstate ligands.
- Marie A. Perrin
- Paul Dutheil
- Victor Mougel
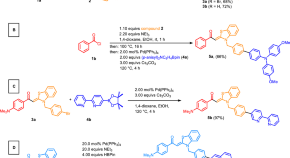
The complexometric behavior of selected aroyl- S , N -ketene acetals shows that they are more than AIEgens
- Lukas Biesen
- Thomas J. J. Müller
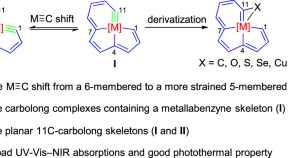
Syntheses and reactivities of strained fused-ring metallaaromatics containing planar eleven-carbon chains
7C-10C and 12C-carbolong complexes with planar ligand skeletons containing 7-10 and 12 carbon atoms have been previously reported but 11C-carbolong complexes with a planar carbon-chain ligand are elusive. Here, the authors prepare metallabenzyne-fused metallapentalenes and metallabenzene-fused metallapentalynes, both representing 11C-carbolong complexes with a planar carbon-chain ligand
- Haiping Xia
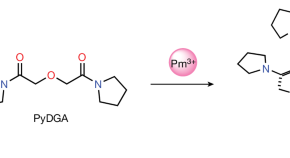
Observation of a promethium complex in solution
Stable chelation of the 147 Pm radionuclide in aqueous solution by the newly synthesized organic diglycolamide ligand is demonstrated and the resulting complex studied, showing accelerated shortening of bonds at the beginning of the lanthanide series.
- Darren M. Driscoll
- Frankie D. White
- Alexander S. Ivanov
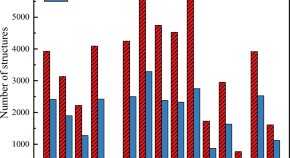
Insights into coordination and ligand trends of lanthanide complexes from the Cambridge Structural Database
- Shicheng Li
- Santa Jansone-Popova
- De-en Jiang
News and Comment
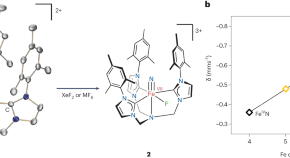
Lifting iron higher and higher
Biological and synthetic catalysts often utilize iron in high oxidation states (+IV and greater) to perform challenging molecular transformations. A coordination complex featuring an Fe(VII) ion has now been synthesized through sequential oxidations of nonheme iron–nitrido precursors.
- Adam T. Fiedler
- Laxmi Devkota
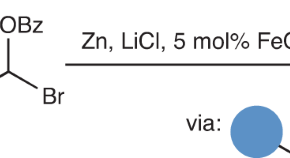
Cyclopropanes from iron carbenes
- Peter W. Seavill
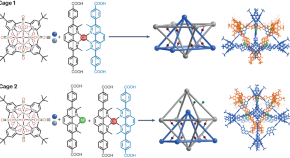
Picking an inter-locked cage
A metal templating approach can be used to generate homo- and heterointerlocked cage structures.
- Stephanie Greed
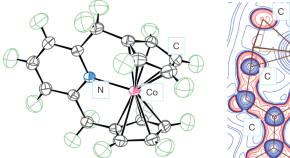
A 21-electron cobalt sandwich
- Joan Serrano-Plana
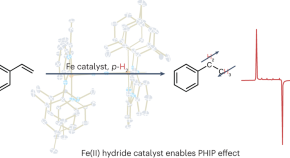
Benign hyperpolarization
- Davide Esposito
Quick links
- Explore articles by subject
- Guide to authors
- Editorial policies


An official website of the United States government
The .gov means it’s official. Federal government websites often end in .gov or .mil. Before sharing sensitive information, make sure you’re on a federal government site.
The site is secure. The https:// ensures that you are connecting to the official website and that any information you provide is encrypted and transmitted securely.
- Publications
- Account settings
Preview improvements coming to the PMC website in October 2024. Learn More or Try it out now .
- Advanced Search
- Journal List
- Int J Mol Sci

Application of Coordination Compounds with Transition Metal Ions in the Chemical Industry—A Review
Jacek malinowski.
1 Faculty of Chemistry, University of Gdańsk, Wita Stwosza 63, 80-308 Gdańsk, Poland; [email protected] (J.M.); lp.pw@79hcyzakinimod (D.Z.); [email protected] (D.J.); [email protected] (J.D.)
Dominika Zych
Dagmara jacewicz, barbara gawdzik.
2 Institute of Chemistry, Jan Kochanowski University, Świętokrzyska 15 G, 25-406 Kielce, Poland
Joanna Drzeżdżon
This publication presents the new trends and opportunities for further development of coordination compounds used in the chemical industry. The review describes the influence of various physicochemical factors regarding the coordination relationship (for example, steric hindrance, electron density, complex geometry, ligand), which condition technological processes. Coordination compounds are catalysts in technological processes used during organic synthesis, for example: Oxidation reactions, hydroformylation process, hydrogenation reaction, hydrocyanation process. In this article, we pointed out the possibilities of using complex compounds in catalysis, and we noticed what further research should be undertaken for this purpose.
1. Introduction
The use of complex compounds in processes employed in the broadly understood chemical industry is a very important aspect of the work of scientists around the world. Processes carried out on an industrial scale and conducted by scientists are enjoying the growing recognition of global corporations dealing in the production of various polymeric materials used in many aspects of our lives [ 1 , 2 , 3 , 4 ].
In order to understand the important function of coordination compounds in catalytic reactions during industrial processes carried out on an industrial scale, it is necessary to learn the exact mechanisms of the reactions carried out. Delving into the mechanism of an exemplary process will allow us to conclude that the most important changes occur in the coordination sphere of the comprehensive relationship. This means that many factors related to geometry, steric hindrance, or the used central atom and attached ligands of the complex compound affect the catalytic reaction.
In recent years, the focus has been on improving the efficiency and selectivity of processes. It has been confirmed that the selection of appropriate ligands in the metal coordination sphere allows a catalyst with properties that have been planned to be obtained. We can therefore conclude that the catalytic properties of the complex compound used depend to the greatest extent on the structure of the catalyst [ 5 , 6 ].
A very important aspect of work on chemical technologies is the smallest possible pollution of the environment, i.e., the application of the principles of green chemistry. Conducting reactions in organic solvents is replaced by supercritical liquids or ionic liquids. Developing the implement of biotechnological and organocatalytic methods allows environmentally friendly chemical technologies to be obtained [ 7 , 8 ].
In this publication, we present the latest methods of using coordination compounds in catalytic reactions used in the chemical industry. We present the influence of coordination compounds on the course of reactions taking place in organic synthesis (for example: Hydroformylation process, hydrogenation reaction, oxidation processes, olefin polymerization processes). We also describe the application of green chemistry principles in a catalysis with the participation of complex compounds.
2. Oxidation Processes
2.1. wacker process.
The Wacker process was developed in cooperation of two concerns, Wacker and Hoechst, and then published in 1959. The process is a well-known reaction for obtaining acetaldehyde by oxidation of ethene. The reaction is carried out in an aqueous environment using a homogeneous catalyst (PdCl 2 · CuCl 2 ). The synthesis was recognized by the chemical industry, due to the fact that it was an alternative to the hydroformylation reaction. The acetaldehyde obtained in the synthesis is widely used. It is mainly applied for production of acetic acid, acetic anhydride or chloroform. In addition, it can form synthetic resins in condensation reactions with phenols and amines. The Wacker process is shown in Figure 1 , in which stoichiometric reactions combine in one catalytic cycle. In the first stage, in an aqueous environment, it reacts with palladium(II) chloride, forming a stoichiometric amount of acetaldehyde, and palladium(II) is reduced to palladium(0). In the next stage, palladium(0) undergoes reoxidation in the presence of copper(II) compounds. Copper(I) is formed as the product of this reaction while in the last stage copper(I) is oxidized with oxygen to copper(II). Reaction 4 in Figure 1 represents the overall reaction of the process [ 9 , 10 ].

Reactions forming the catalytic cycle of the Wacker process [ 1 ].
According to research recommendations, the oxidation stage is actually catalyzed by [PdCl 4 ] 2− , which is formed under the conditions of water and Cl − ions. In the analyzed cycle, two chloride ligands are replaced by ethene and water. In the next stage, there is a nucleophilic attack of water on ethene in the complex, followed by substitution of the chloride ligand by another water molecule. β-elimination of a hydrogen atom leads to the formation of vinyl alcohol. This stage is followed by a series of processes leading to the production of acetaldehyde and palladium(II) hydride. Due to the fact that palladium(II) hydride is unstable, Pd(0) and HCl are formed as a result of the reduction reaction. The cycle is closed. Palladium(0) is oxidized to palladium(II) with CuCl 2 [ 11 , 12 ].
In [ 13 ] the researchers carried out quantum mechanical tests and they concluded that the anti-nucleophilic attack is the step determining the Wacker process under standard conditions. Unfortunately, experimental studies did not confirm the results obtained by calculation methods. Keith et al. report that the Wacker process is determined by a syn-nucleophilic attack [ 14 ].
The Wacker oxidation usually gives ketones as reaction products, but the literature reports that one can carry out the Wacker process that will give 99% selectivity in obtaining aldehydes, e.g., using 1,4-benzoquinone, t-BuOH, and PdCl 2 (MeCN) 2 [ 15 , 16 , 17 , 18 ]. Regarding the selectivity of the Wacker process, products with high selectivity are also obtained using styrene derivatives as substrates with the reaction carried out under mild conditions [ 19 ].
Studies conducted over the years proved that the Wacker process produces environmentally hazardous, chlorinated by-products, as well as harmful copper waste. According to the principles of green chemistry, solutions are being sought that affect the protection of ecosystems and the whole world. Researchers at the University of Pune proposed the Pd(0)/C system as a heterogeneous catalyst that can be recycled. This allowed the elimination of pure CuCl 2 . In addition, they used potassium bromate KBrO 3 as an oxidizing agent instead of molecular oxygen. By that, they developed a method using inexpensive substrates and achieved high process efficiency [ 20 ].
One may conclude that the by-products formed during the Wacker process are a major disadvantage of this cycle. Chlorine derivatives are environmental pollutants. Therefore, scientists should attempt to deactivate the resulting by-products.
2.2. Hydroperoxide Epoxidation Reaction of Olefins Catalyzed by Mo(VI) Complex
Epoxides are olefin oxides. Their structure contains a three-membered ring made of two carbon and oxygen atoms. They are the heterocyclic compounds. These compounds are characterized by high reactivity. For example, they undergo reactions that are accompanied by ring opening and closing followed by attachment of the nucleophile [ 21 , 22 ]. These reactions are used in the production of hypertension medications, antibiotics, and steroids. Hydrogen peroxides are the most common oxidants in the olefin epoxidation processes; H 2 O 2 is rarely availed because it poorly dissolves in hydrocarbons. The course of the process is catalyzed by metal oxides of groups 4–6, e.g., Ti, V, Mo, W. There are many methods of epoxide synthesis, but the most well-known is the propene epoxidation reaction. According to data, propene oxide production in the world is close to 6 million tons per year [ 12 ].
One of the epoxidation methods, which includes peroxide metal compounds, is the reaction of olefin epoxidation with hydroperoxide catalyzed by the Mo(VI) complex with a peroxide ligand. Olefin is attached to the active form of the catalyst, namely the ROO group. Olefin binds to the catalyst through an oxygen atom, which is a characteristic feature of this reaction mechanism. No bond is formed by the metal atom; however, molybdenum in the 6th degree of oxidation will have a positive effect on the breaking of the O–O bond and restoration of the catalyst [ 11 , 12 ].
The epoxy—propene oxide—formed in the reaction is commonly used for the production of polyether and polyhydric alcohols, ethers, or propylene glycols.
Studies on epoxidation using molybdenum(VI) compounds have contributed to the development of significant stereoselectivity and efficiency of conducted reactions. Unfortunately, the use of solvent-free epoxidation reactions remains to be explored.
2.3. Sharpless System for Asymmetric Epoxidation of Olefins with Hydroperoxide with Dimeric Titanium Complex as a Catalytically Active Form
The development of the chemistry of organometallic compounds contributed to the growing interest in the utilize of newly created systems. Sharpless conducted research on the use of organometallic catalysts in the asymmetric epoxidation reaction [ 23 ]. Allyl alcohol epoxidation is carried out with the participation of a system consisting of titanium(IV) isopropoxide, tartaric acid derivatives, and an oxidant in the form of tert-butyl hydroperoxide ( Figure 2 ). Ti(O- i Pr) 4 acting as a catalyst is a dimeric complex of titanium with tartaric ligands [ 21 , 24 ]. The tartaric acid ethyl ester used affects the stereochemistry of the reaction product. The ester interacting with the metal will form a complex. The resulting system undergoes the oxidation of hydrogen peroxide and the bond breaks on the appropriate side of the hydrogen bond depending on the chirality of the tartrate derivative [ 25 , 26 ].

Sharpless system diagram for asymmetric olefin epoxidation [ 21 ].
The problem in the Sharpless system for asymmetric epoxidation of olefins with hydroperoxide with the dimeric titanium complex as a catalytically active form is the use of chlorinated solvents. For this reason, this reaction creates many problems in industrial production.
2.4. Cyclohexyl Hydroperoxide Decomposition Reaction Catalyzed by Cobalt(II) and Cobalt(III) Complex Compounds
Improving the technology of obtaining polymer fibers is the subject of research in numerous research centers. Caprolactam, which is used for the production of polyamide fibers and plastics, is particularly important in great chemical synthesis. According to statistical data, caprolactam production capacity is 5.2 million tons per year. It is worth noting that the cyclohexanol–cyclohexanone mixture from hydrogenated benzene has a huge share in caprolactam production in Poland and worldwide. It is estimated that 90% of the world’s production of this raw material uses this technology [ 27 , 28 , 29 ]. In Poland, the leading production process for caprolactam is CYCLOPOL technology developed at the Industrial Chemistry Research Institute in cooperation with Zakłady Azotowe in Tarnów and Puławy. CYCLOPOL technology has also been implemented in other countries, i.e., Russia, India, Spain, Italy, and Slovakia. In the first stage of the process, the hydrogenation of benzene to cyclohexane occurs. The cyclohexane oxidation process takes place in a bubble reactor. It is carried out in the liquid phase at a temperature in the range 155–165 °C, under pressure from 0.8 to 1.05 MPa. The technology implies that air or air enriched with oxygen should be an oxidant. A mixture of cobalt and chromium catalysts that support cyclohexane oxidation to a mixture of cyclohexanol and cyclohexanone and decomposition of hydroperoxides is also used. The next stage is the distillation of a mixture of cyclohexanols and cyclohexanone, followed by their dehydrogenation [ 12 ]. The key step is the cyclohexane oxidation reaction. The various cobalt compounds, e.g., naphthenate, play the catalyst role in this step. An important reaction step is the decomposition of cyclohexyl hydroperoxide. The reaction takes place according to radical mechanism [ 4 ].
Cobalt(II) and cobalt(III) compounds are described in the literature as catalysts in this reaction. The mechanism of catalysis is described by the Haber–Weiss reaction [ 4 ].
Ongoing work on improving the cyclohexane oxidation reaction node at the Tarnów plant has resulted in significant technological changes. The new CYCLOPOL–bis process consists of two stages. The process of synthesis of cyclohexyl hydroperoxides was separated from the process of their selective decomposition. In addition, optimal aeration, pressure, and temperature parameters were selected for these separate oxidation phases. The modernized CYCLOPOL–bis technology has allowed production costs to be minimized, the quality of products to be improved, and the effects on the environment to become less toxic [ 12 ].
Research to improve the selectivity of decomposition reactions is still needed. It should be emphasized that the CYCLOPOL–bis technology is close to the principle of green chemistry.
3. The Hydrocyanation Reaction
Hydrocyanation is used in the industry in the production of adipic acid nitrile. It is the basic raw material for the production of nylon 6,6, the reaction of which consists of attaching HCN to the olefin. DuPont company processes possess an economic significance, where adipic acid nitrile is obtained in the process of butadiene hydrocyanation. The process uses the Ni(0) catalyst with phosphate ligands [ 20 ].
The process consists of two stages. The catalyst plays a key role in the whole process. At first, the oxidative attachment of HCN to the NiL 4 catalyst occurs and the hydrocyanide complex is formed. At this stage, the selection of a suitable ligand (L), e.g., a phosphorus ligand, is particularly important. Electronic and steric properties are taken into account, which have an impact on the course of individual stages of the hydrocyanation reaction. On the one hand, more basic ligands favor the addition of HCN. On the other hand, less basic ligands, which are characterized by high steric hindrance, are more popular [ 12 , 30 ]. Representative of this group is o-methylphenyl phosphate P(O-o-MeC 6 H 4 ) 3 , which facilitates the reductive elimination of the product (3-pentenenitrile). According to the research, steric effects in the case of phosphine and phosphite ligands have a significant impact on the stability of Ni(0) complexes [ 31 , 32 ]. IR analyses determining changes of carbonyl vibration frequency (νCO) in [Ni(CO) 3 L] (where L denotes a broad variety of phosphorus ligands) complexes have shown that the nature of the ligand π acceptor increases the stability of Ni(0) complexes [ 33 ]. The steric properties of the ligands are represented using the Tolman cone angle. For ligands with a small cone angle of approximately 109°, dissociation will not occur even if we use high dilution solutions [ 31 , 32 ]. An example of such a complex is [Ni[P(OEt) 3 ] 4 ]. 16-electron complexes, e.g., [Ni[P(O-o-tolyl) 3 ]], whose Tolman cone angle was 141°, and alkene complexes [Ni(alkene)L 2 ] were also examined. Subsequent studies have determined that the increase in metal–alkene bond strength is affected by the replacement of the H-alkene atom by a more electronegative CN. The electron effect is visible here, which results in higher stability of the acrylonitrile complex. Tolman also presented in his researches 1 H and 31 P NMR studies of the complex [HNi(CN)L 3 ] (where L is various phosphorus ligands) [ 34 , 35 ]. The addition of hydrogen cyanide to nickel(0) complexes is represented by the following reactions, where protonation is preceded by the dissociation of the ligand. The scientist pointed out that the addition of excess HCN may result in the formation of an undesirable complex [Ni(CN) 2 L 2 ] (where L is a phosphorus donor ligand), which deactivates the catalyst. For this reason, [Ni(CN) 2 L 2 ] is not active in the process of hydrocyanation [ 36 , 37 , 38 ].
Research concerns the HCN addition to butadiene at the first stage and the reaction taking place in the presence of a NiL 4 catalyst ( Figure 3 ). The reaction products are 3-pentenenitrile and 2-methyl-3-butenenitrile. The resulting isomer in branched form is not a substrate in the reaction of obtaining adipic acid nitrile; therefore, it is subjected to the process of isomerization to 3-pentenenitrile. The next stage takes place using the same catalyst with addition of Lewis acid. The Lewis acids used can be ZnCl 2 , ZnBr 2 , AlCl 3 , BPh 3 . It works with a free electron pair on the nitrogen of the CN group, which facilitates the creation of C–C connections. Isomerization of the double bond occurs and the 3-pentenenitrile is structured to an alkene (4-pentenitrile). The second CN group is joined in this reaction. Secondary by-products include 2-methylglutaronitrile (MGN), ethyl succinonitrile (ESN), and 2-pentenenitrile (AdN). Studies show that the hydrocyanation rate relative to isomerization is the highest for AlCl 3 and decreases in the order of AlCl 3 > ZnCl 2 > BPh 3 [ 12 , 29 , 30 , 38 ].

Mechanism of butadiene hydrocyanation [ 38 ].
In 2019 it was confirmed that hydrocyanation can run without cyanide [ 39 ]. The mentioned reaction was asymmetric olefin hydrocyanation. In the alkenic hydrocyanation process, the use of bident phosphorus-based ligands is of great importance, which contributes to increasing the stability of the products obtained during hydrocyanation [ 40 ]. In addition to nickel complexes, ruthenium(I) complexes for hydrocyanation are used [ 41 , 42 , 43 ].
The important issue regarding the hydrocyanation reaction is alkene isomerization. Low reaction yields are a disadvantage of hydrocyanation reactions. These issues remain to be improved.
4. Metathesis Reaction
The first studies on the metathesis reaction date back to the 1930s. Schneider and Frolich described the pyrolytic conversion of propene to ethene and butene, which took place without the use of a catalyst at a high temperature of about 852 °C [ 44 ]. Metathesis has been a major course in academic and industrial research for years. Banks and Baile’s research, conducted in 1964, on the alkylation of isoalkenes with olefin on heterogeneous catalysts [Mo(CO) 6 /Al 2 O 3 , WO 3 /SiO 2 , Re 2 O 7 /Al 2 O 3 ] at 160 °C turned out to be a breakthrough. These studies were aimed at obtaining fuels with a higher octane number, and also brought new information on the disproportion of linear olefins. Based on the developed technology, Philips Petroleum began production of high purity ethylene and but-2-ene from propylene [ 45 ]. Similar studies were conducted by Natta, who proved that the presence of homogeneous catalyst [TiCl 4 /AlEt 3 , MoCl 5 /AlEt 3 , WCl 6 /AlEt 3 ] cyclobutenes and cyclopentenes allows for the polymerization of the ring opening metathesis polymerization (ROMP) type already at low temperature. His work concerned the copolymerization of ethylene with both linear and cyclic olefins and the polymerization of cyclic alkenes (ring opening metathesis polymerization; ROMP). Catalytic systems based on metal oxides on a solid support were also known. Due to the low cost of their production, and thus their wide availability, they were used in the Shell higher olefin process (SHOP) and in further exploitation of advanced Shell technology (FEAST) [ 46 , 47 ].
At the same time, numerous studies were being conducted, each of which was characterized by the use of compounds containing multiple bonds. In addition, the reactions mentioned took place under the influence of catalysts. The research aimed to find out the exact reaction mechanism that could explain the experimental results obtained. Researchers tried to describe the individual reactions with understanding of the structure of the transition state. They strived to characterize the structure of the catalytic center and determine which homo- or heterogeneous catalysts affect the conversion of compounds containing multiple bonds.
The creator of the term “metathesis” is Calderon, who used the synthesized heterogeneous catalytic system WCl 6 /EtAlCl 2 /EtOH to synthesize but-2-ene and hex-3-ene from pent-2-ene [ 48 , 49 ].
The exact definition of metathesis was provided by the Nobel Prize winners in chemistry: Grubbs, Schrock, and Chauvin (2005). Metathesis is a catalytic reaction accompanied by an exchange of double bonds between carbon atoms [ 12 , 50 ]. This reaction can be applied to various chemical compounds, for example: Internal, terminal, and cyclic olefins, as well as dienes and podiums. Metathesis reactions usually do not require high temperatures and pressure. In addition, all atoms are used in metathesis processes, and the resulting by-products can be processed or reused. Therefore, the reactions are reversible and meet the requirements of green chemistry [ 51 ].
The olefin metathesis process is considered to be an especially important technology for the production and processing of olefins. Considering the type of substrate used, we distinguish the following types of metathesis [ 51 ]:

- Ring Closing Metathesis—RCM

- Ring Opening Metathesis Polymerization—ROMP
- Acyclic Diene Metathesis—ADMET
Chauvin worked on the metathesis process with his student, Herisson. The mechanism of metathesis proposed by these colleagues is shown in Figure 4 . They assumed that the metathesis reaction was catalyzed by alkylidene metal complexes. The first stage of the process is the production of metallycyclobutane II by reacting the R 1 -CH=CH 2 substrate with the complex I . The resulting complex degrades to the alkene, in this case, ethene, and to the corresponding metallocarbene. The resulting metallocarbene III reacts with the olefin R 2 -CH=CH 2 , and the subsequent decomposition of IV leads to the formation of carbene I . Carbene as an alkylidene complex is a proper reaction catalyst [ 52 , 53 ].

The mechanism of metathesis reaction.
Scientists in their research present homogeneous and heterogeneous catalytic systems. The metals used in the design of carbene complexes are transition metals with incompletely filled 3d, 4d, or 5d coatings [ 53 ]. Ruthenium, tungsten, molybdenum, and rhenium are most commonly applied. One of the first catalysts used in the described reaction was one-component tungsten carbide Ph 2 C=W(CO) 5 . The next step in the development of research on alkylidene catalysts was Schrock’s synthesis of a molybdenum complex ( Figure 5 ). This complex showed high catalytic activity but was unstable. It was one of the first complexes that could be used without the activators, e.g., Lewis acids. It is worth noting that it is applicable for the synthesis of sterically crowded compounds. Disadvantages of this catalyst were sensitivity to oxygen, moisture, and polar groups. Complex compounds based on ruthenium are of particular interest. They show high reactivity towards olefins (C–C bonds) and tolerance to the functional groups of the substrates [ 53 , 54 , 55 ]. In addition, they are stable and do not react negatively to oxygen and moisture that may be present in the reaction system. An example of such a complex is the first-generation Grubbs catalyst ( Figure 5 ). The catalyst synthesis is shown in Figure 5 . The resulting complex, in addition to the above-mentioned features, is easy to store. It is one of the most commonly used complexes in metathesis reactions due to the fact that it can act toward obtaining carbo- and heterocyclic ring compounds, as well as macrocyclic compounds [ 54 ]. In addition, it catalyzes the metathesis cyclization reaction of enines and the alkene–alkyne metathesis. It is often used in polymerization reactions: ADMET, ROMP, and living polymerization of norbornenes [ 54 ]. It does not catalyze cross metathesis with α, β-unsaturated compounds, which is its drawback. Catalysts that contain imidazole derivatives are also noteworthy. N-heterocyclyl carbenes are obtained by reacting a first-generation Grubbs catalyst with a compound that is a source of stable carbene, e.g., the imidazolidine salt ( Figure 6 ). In comparison to the first-generation catalyst, it takes part in reactions with α, β-unsaturated olefins, catalyzes ROMP cycloolefins with low ring stresses, and shows much higher activity.

Metathesis catalysts, from the left: ( A ) Schrock’s molybdenum complex, ( B ) first-generation Grubbs catalyst, ( C ) second-generation catalyst.

Grubbs catalyst preparation reaction.
Nickel complexes with chelate ligands have been used in metathesis reactions in a multistage SHOP process [ 54 , 55 ]. Initial material in this reaction is ethene. With the participation of a nickel catalyst, ethene is oligomerized and C 4 -C 40 olefins are formed. Light < C 6 and heavy > C 18 olefins in the isomerization process are converted into appropriate internal olefins. In the next stage of the metathesis process, olefins of medium length C 11 –C 14 are formed from them. The obtained internal olefins are used in the production of surfactants. In addition, the olefins subjected to a carbonylation process and hydrogenated to alcohols are the substrates in the synthesis of alkyl-benzenes. The separated C 6 –C 18 fraction is used for the production of plasticizers, detergents, and also lubricants. The oligomerization catalysts are the nickel complexes mentioned above. Chelate ligands are coordinated by oxygen and phosphorus atoms. Scientists pay particular attention to the hemilability of metal-coordinated ligands. Such ligands have the ability to open and close the ring, which is possible due to the different strength of coordination of atoms that act as donors. This property allows transitions between cis- and trans-forms, and the change in symmetry affects the selectivity of the reaction. Due to the selectivity, scientists are conducting numerous studies on the synthesis of catalysts, affecting the preparation of sterically crowded olefins and catalysts, which control the stereoselectivity of reactions [ 55 ].
The following complexes are olefin metathesis catalysts: Heterocyclic carbene-coordinated compounds of ruthenium, salamo-type bisoxime complexes of Co (II) and Ni (II), carboxylate, phenolate, hydroxycarboxylate, and catecholate derivatives of Ti (IV) [ 56 , 57 , 58 ]. It should also be noted that FeCl 3 also catalyzes the metathesis reaction [ 59 , 60 ].
In our view, the metathesis reaction provides many opportunities for the creation of various chemical compounds. It has application in refining industry and chemical studies. It is a powerful method of synthesis. For this reason, it is worth looking for new catalysts that meet the principles of green chemistry that can be used in metathesis.
5. Hydrosilylation Reaction
5.1. reduction pt(ii) to pt(0) in reaction with silane hsi(oet) 3.
Silicon compounds are widely used in the modern world. They are applied as additives to cleaning products, cosmetics, and even food products. In addition, they increasingly have found application in electronics and the automotive industry. For example, organosilicon polymers, polycarbosilanes, and polycarbosiloxanes are used as precursors of ceramic materials and are resistant to chemical agents. Some of these materials exhibit optoelectronic, photoconductive, or electroluminescent properties. The synthesis of silicon derivative compounds, necessary for the production of the above materials, is based on the hydrosilylation reaction. Hydrosilylation is the catalytic addition reaction of organic and inorganic hydrogen silanes to unsaturated compounds. Depending on the substrate used in the synthesis, new Si-C, Si-heteroatom bonds are formed. The most commonly used hydrosilylation catalysts are platinum(II) complexes; however, other transition metal coordination compounds are also known, e.g., Pd(II), Co(II), Ni(II), Ir(I), Rh(II), and Ru(I). Scientist reports inform about numerous homogeneous catalysts active in the hydrosilylation reaction. They are [PtCl 2 (cod)], [Pt(CH 2 =CHSiMe 2 ) 2 O 3 ], [RhCl(PPh 3 ) 3 ], [Pd 2 (dba) 3 ], where cod—cyclooctadiene, dba—dibenzoylacetone [ 61 ].
As an example of the reaction of reducing platinum(II) to platinum(0) is the reaction of [PtCl 2 (cod)] with (EtO) 3 SiH ( Figure 7 ). The metal colloid formed in the reaction can also be used in the hydrosilylation process.

Example of the reduction of platinum(II) to platinum(0).
It should be noted that the hydroformylation reaction is commonly used in the industry for siloxane and silanes production. Chemoselectivity of hydroformylation means that its applicability for the synthesis of new compounds is high. Currently, hydroformylation catalysts are not based on platinum due to the reduction of catalyst costs. The use of new catalysts in hydroformylation opens up the possibility of designing new transition metal compounds with catalytic properties in this process.
5.2. Karstedt’s Catalyst
Platinum complexes are most commonly used in hydrosilylation of carbon–carbon double bonds. Karstedt’s catalyst was described in 1973 as a compound of platinum(0) with divinylsilaxane ligands. It is formed in the reaction of divinyltetramethyldisiloxane with chloroplatinic acid, and it is applicable in numerous reactions due to its properties, i.e., high catalytic activity and good solubility in polysiloxane systems ( Figure 8 ) [ 61 , 62 ].

Karstedt’s catalyst.
Therefore, the high activity and selectivity of Karstedt’s catalyst causes it to be successfully used in industry. It will be difficult to find a replacement for this hydroformylation catalyst.
5.3. Hydrosilylation Mechanism Proposed by Chalk and Harrod and Modified Mechanism of Chal–Harrod—Catalyzed by Rhodium Complex Compounds
Reactions entering the cycle of the hydrosilylation process may take place with the participation of free radicals or in the presence of catalysts in the form of transition metal complex compounds. It is worth paying attention to the mechanisms of the hydrosilylation process of carbon–carbon multiple bonds in the presence of transition metal complexes. The use of transition metal complexes as catalysts affects the selectivity and speed of individual reactions. The hydrosilylation process is widely used in the synthesis of organosilicon compounds. In the 1960s, Chalk and Harrod introduced the first concept of a hydrosilylation mechanism [ 61 ]. In their research, they used hexachloroplatinic acid H 2 [PtCl 6 ] as a homogeneous reaction catalyst. In the first stage, silane is attached to the catalyst metal. The configuration of the transition metal used in the catalyst is usually d 8 or d 10 [ 61 ]. The second stage leads to a stable alkene complex. Olefin coordination will only occur when the electron cloud transfer from the double bond to the hybridized metal orbit occurs. It is important to use excess substrate (olefin). As a result of the oxidative addition of HSiR 3 to the complex, an M-H and M-Si bond is formed. The rearrangement of π–σ is considered a key stage. The migratory insertion of olefin for M–H binding generates a silyl–alkyl complex. The reaction product is formed by reducing the elimination of the alkyl complex [ 61 , 62 , 63 ].
Research on the formation of unsaturated products in the hydrosilylation process was also undertaken. The explanation for this problem is the modified Chalk–Harrod mechanism, catalyzed by rhodium complex compounds. Although the product obtained in both processes is the same and does not differ in any way, the process itself changes. This is visible at the key stage which is alkene insertion. In the new process, it occurs to the M–Si bond, not to the M–H bond. The final stage proceeds in the same way by the elimination of alkenylsilane. Both processes are shown in the diagram ( Figure 9 ) [ 61 , 62 , 63 ].

Hydrosilylation mechanism and modified mechanism proposed by Chalk and Harrod [ 63 ].
The hydrosilylation of alkenes with tertiary silanes can run without the use of a catalytic activator but with the use of the catalyst itself, i.e., cobalt(II) amide coordination compounds [ 64 , 65 , 66 , 67 ]. It is worth noting that alkene hydrosilylation can be successfully catalyzed by earth-abundant transition metal compounds [ 68 ].
The regioselectivity and stereoselectivity of the hydroformylation reaction depends on many factors e.g., solvent, catalyst, or temperature. Thus, it should be noted that the characteristic of the actual structure obtained product is difficult.
6. Hydroformylation Reaction
Hydroformylation is the reaction of olefins with hydrogen and carbon monoxide(II), where the products are straight chain (n) and branched chain (iso) aldehydes. This process occurs under the influence of the catalyst present during the reaction [ 69 , 70 ]. The products obtained in the form of aldehydes can be converted into alcohols, carboxylic acids, acetals, diols, or aldols under appropriate conditions. Most often, however, the intention of the reaction is to obtain an unbranched chain aldehyde. The catalytic system is most often characterized by the n/iso ratio. As we can see, the higher the numerical value of the ratio, the better the catalytic system used during the hydroformylation. The hydroformylation reaction scheme is shown below.
R-CH=CH 2 + CO + H 2 → R-CH 2 -CH 2 -CHO + R-CH(CH 3 )-CHO
The hydroformylation process was discovered by German chemist, Roelen, in 1938. He made the discovery during the Fischer–Tropsch reaction [ 71 ]. The first catalyst used during the hydroformylation reaction was hydridotetracarbonyl—[HCo(CO) 4 ]. Then, the addition of phosphines to cobalt catalysts was applied, which resulted in a higher n/iso ratio and the hydroformylation process was carried out at a lower pressure and temperature.
A great breakthrough in the search for catalytic systems was made thanks to the work of the English Nobel Prize winner Sir Geoffrey Wilkinson. They allowed the discovery of compounds based on rhodium atoms that showed a thousand-fold higher catalytic activity in the hydroformylation reaction compared to cobalt catalysts [ 72 , 73 ].
Rhodium compounds, despite a higher price, are commonly used as catalysts for hydroformylation reactions. Union Carbide Davy Powergass Johnson–Matthey LPO—a British chemical company—uses a hydroformylation process using a precursor of formula [(acac)Rh(CO) 2 ] and a modifying ligand, phosphine (PPh 3 ). The addition of approximately 10% by weight PPh 3 produces the active catalyst form—[(acac)Rh(CO)(PPh 3 )]. The hydroformylation process is carried out under the following conditions: At a temperature from 60 °C to 120 °C, pressure in the range of 10–50 bar. This process has a n/iso ratio of around 1:5. The hydroformylation process in Kędzierzyn–Koźle at Zakłady Azotowe is used in Poland [ 74 , 75 ].
Kuntz’s discovery of the industrial conduct of the hydroformylation process and its commercialization by Ruhrchemie/Rhone-Poulenc is based on the use of a two-phase catalytic system based on a rhodium complex compound. Tris(3-sulfonylphenyl)phosphine sodium (TPPS) was used in the hydroformylation process ( Figure 10 ).

Simplified formula of sodium salt (tris(3-sulfonylphenyl)phosphine).
The use of the aforementioned catalyst system allows easy separation of the catalyst from the desired products (aldehydes) because it is in the polar phase. Therefore, the catalyst is used in a continuous process. Conducting the hydroformylation process using a two-phase catalytic system allows for high selectivity and activity for the production of a linear product to be maintained [ 76 ].
The electron and steric properties of ligands attached to the rhodium atom influence the selectivity of the obtained product in the hydroformylation reaction. A larger amount of the linear product in the olefin hydroformylation reaction is obtained by using ligands with weaker basic properties—for example, pyrrolylphosphines or phosphites [ 77 , 78 , 79 ].
The steric properties of the ligands are evaluated based on the size of the conic angle, while for chelate ligands they are based on the grip angle, which determines the value of the angle between the P–M–P bonds present in the complex with the ligand. Studies on the impact of the grip angle on the selectivity of the reaction have shown that the equatorial–equatorial location of donor atoms in chelating ligands found in rhodium atom-based catalyst systems results in obtaining the best n/iso values. This means that the geometry of the respective rhodium complexes, in this case, for grip angles, is about 120°, exhibiting the geometry of the trigonal bipyramid.
The newest reports from scientists inform that the use of carbene and phosphorus ligand catalysts simultaneously attached to the rhodium atom in the coordination sphere results in obtaining a much better n/iso ratio. [RhH(NHC)(CO)P(OPh) 3 ) 2 ], where NHC denotes heterocyclic carbene, is an examples of such a catalyst [ 80 , 81 , 82 , 83 ].
The catalysis of the hydroformylation process is constantly evolving with regard to the use of rhodium compounds as catalysts [ 84 ]. Even a rhodium single-atom exhibits catalytic activity in hydroformylation similar to the RhCl(PPh 3 ) 3 complex [ 85 ]. Rhodium particles also have catalytic properties in hydroformylation [ 86 , 87 ]. In 2018 it was published that Fe(II) has the ability to catalyze hydroformylation of alkenes under mild conditions [ 88 ].
The most important advantage of the hydroformylation reaction is the ease of separation of the resulting product and catalyst. It is very important when receiving new materials.
7. Carbonylation Reaction
The carbonylation reaction is the process of attaching a carbon monoxide(II) molecule to an organic compound [ 89 , 90 , 91 ]. Most often, this process is used to produce acetic acid from methanol or an anhydrous derivative of the acid from the ester—methyl acetate. This reaction is carried out in the presence of transition metals, specifically metals in group 9, cobalt, rhodium, and iridium are used primarily. In addition, an iodide co-catalyst is needed during the process to activate methanol. This reaction produces acetyl iodide, which in subsequent hydrolysis produces a mixture of acetic acid and hydroiodic acid.
BASF carried out the first commercially conducted process of carbonylation of methyl alcohol with a cobalt catalyst. Then, industrial scale carbonylation of methanol was started by Monstanto, which applied a rhodium catalyst. The conditions operated by Monstanto compared to BASF differed in the use of lower carbon monoxide pressure and temperature. This process was also carried out with much better selectivity for methyl alcohol.
Studies on the mechanism of methanol carbonylation were conducted very thoroughly. Maitlis’ group examined the reaction using infrared spectroscopy and found that the mechanism of methanol carbonylation contains two catalytic cycles. The first cycle, rhodium, involves organometallic compounds and an iodide cycle containing organic reactions. The catalyst for this carbonylation reaction was the rhodium complex compound, cis-[Rh(CO) 2 I 2 ] − [ 92 ].
The methanol carbonylation process proposed by BP Chemicals, called the Cativa process, was carried out using an alternative iridium catalyst. The main difference of this process compared to the mechanism proposed by Monstanto is that it has about 100 times faster oxidation caused by the addition of CH 3 I to [Ir(CO) 2 I 2 ] − than to [Rh(CO) 2 I 2 ] − .
However, a different type of catalyst is used when carbonylation of higher alcohols is carried out. This is caused by too slow a reaction using a rhodium catalyst. Therefore, palladium catalysts are applied that simultaneously also catalyze the olefin hydroxycarbonylation.
The palladium complex compound-PdCl 2 (PPh 3 ) 2 is used in the carbonylation reaction, where the product is the known anti-inflammatory drug, ibuprofen. This synthesis is carried out by Boots–Hoechst–Celanese. Below, Figure 11 shows a simplified formula of this catalyst.

Simplified formula of the palladium complex used during ibuprofen synthesis.
Naproxen is another very well-known remedy in which production palladium complexes are used as catalysts. This drug belongs to non-steroidal inflammatory drugs, which are obtained in a multistage synthesis. The palladium complex is a catalyst during stage 2—Heck reaction, and during stage 3—hydroxycarbonylation.
Both ibuprofen and naproxen synthesis can be carried out in the presence of chiral phosphine. Reactions carried out in ionic liquids, using a ruthenium coordination compound as a catalyst, and with the introduction of a chiral phosphine allows us to obtain the appropriate optical isomer. Below, Figure 12 shows the catalyst used to obtain (S)-ibuprofen [ 93 ].

Ruthenium catalyst from S-BINAP used for the synthesis of S-ibuprofen.
Hydroaminocarbonylation of olefins, aminocarbonylation of aryl iodides, and oxidative carbonylation of amines occurs with the application of bulk Pd catalyst without the use of organic ligands [ 94 , 95 ]. Iridium and immobilized-rhodium catalyze the methanol carbonylation process, leading to the formation acetic acid [ 96 ]. An interesting fact is that membranes, e.g., chitosan, polyamide, polyvinyl alcohol, are used to catalyze carbonylation reactions [ 97 ]. Membranes take part in the product separation of carbonylation. In 2019 K 2 [Fe(CO) 4 ] as a catalyst promoting the formal insertion of CO was published [ 98 ].
Therefore, in the carbonylation reaction, iron(II) and platinum(II) complexes should be tested as catalysts. Palladium(II) complexes satisfactorily perform the role of catalyst carbonylation reaction; therefore, due to the similarity, analogous platinum(II) complexes should be tested.
8. Olefin Polymerization
Polymerization of olefins is a process that is used commercially around the world on a very large scale in the chemical industry. This is evidenced by the fact that the sum of the annual production of polyethylene and polypropylene is about 15 million tons. Another important aspect of using complex compounds such as olefin polymerization catalysts is the fact that it is economically cheap and environmentally friendly [ 99 ].
The newest version of the Ziegler–Natta catalysts used in the olefin polymerization process is the deposition of titanium(IV) tetrachloride on magnesium chloride. The MgCl 2 , the carrier, has a grain diameter of about 50 µm. A carrier with such grain diameter characteristics is obtained by precipitation from a soluble precursor solution with the addition of a Lewis base or by mechanical milling. The second stage is the addition of phthalic esters or silyl ethers. Magnesium(II) ions and titanium(III) ions have similar ionic rays, which make it possible to obtain a third-generation catalyst. This synthesis consists of adding titanium(I) tetrachloride to a solution of alkoxide, sulfide, or carboxylate containing magnesium(II) ions. Then the addition of TiCl 4 and phthalates activates the catalyst [ 100 ].
Atom transfer radical polymerization (ATRP) is a technique that allows the production of polymers and co-polymers used commercially around the world. Polish chemist Matyjaszewski, working in the United States, discovered and developed this method of polymerization. The ATRP technique is cheap and does not pollute the environment in the production of polymeric materials on an industrial scale.
The application of radical transfer polymerization techniques allows for polymers with the appropriate structure and morphology to be obtained. Thanks to such control possibilities of the process being carried out, its application in various industrial technologies is becoming wider.
Copper and iron coordination compounds are used as catalysts during radical atomization polymerization. Application of copper compounds during the process determines the necessity of the presence of reducing agents. The polymerization process carried out in water can be in homogeneous conditions or heterogeneous. Below, Figure 13 shows an example of a complex which is a catalyst for the ATPR process.

An example of a copper complex used as a catalyst in atom transfer radical polymerization.
The technique of radical polymerization with atom transfer allows for the production of polymers that are components of moisturizing agents, surfactants, paints, and surfactants. It is also used in the synthesis of polar thermoplastic elastomers. It also allows the improvement of hydrophilic, antibacterial, or conductivity properties in the polymer materials produced.
Radical polymerization using organometallic compounds (OMRP) is another technique used in polymerization. This method was initially proposed for the polymerization of acrylates. Acrylic acid, vinyl acetate, or other substrates are the monomers. Titanium, cobalt, iron, and chromium compounds have found application in this technique as catalysts. Figure 14 shows examples of ruthenium complexes used during OMRP [ 101 , 102 ].

Titanium compounds used as catalysts in radical polymerization using organometallic compounds.
In our view, olefin polymerization is a wide field for research, because nowadays there are reports of ever-increasing catalysts of this reaction being non-metallocene transition metal complexes [ 103 , 104 , 105 , 106 , 107 , 108 ]. The new complex compounds have higher values of catalytic activities than those traditionally used, and their synthesis is simple and cheap. New catalysts are chromium(III), oxovanadium(IV), and cobalt(II) complexes.
Late transition-metal catalysts are used in olefin polymerization. However, one should bear in mind that most of them cause the formation of amorphous and atactic polymers [ 109 , 110 ]. [Cu(2,3-pydc)(bpp)]·2.5H 2 O, [Zn(2,3-pydc)(bpp)]·2.5H 2 O, and [Cd(2,3-pydc)(bpp)(H 2 O)]·3H 2 O (2,3-pydc denotes pyridine-2,3-dicarboxyliate, bpp = 1,3-bis(4-pyridyl)propane) are metal–organic frameworks which are applied in heterogeneous catalysis [ 111 ]. Tridentate ligands based on N-, P-, and S-donors are used to synthesize the chromium(III) catalyst for ethylene trimerization and tetramerization [ 112 , 113 ].
One may conclude that this field must be developed because new catalysts can successfully replace traditionally used ones, which, unlike new catalysts, unfortunately did not meet the principles of green chemistry.
9. Hydrogenation Reaction
The hydrogenation reaction is a very important organic chemistry reaction. Hydrogen, one of the most important elements in the chemical industry, is used in almost 4% of hydrogenate carbon and hydrogen compounds. The mechanism of this reaction requires the presence of a catalyst, which is most often transition metal compounds. This process is carried out to create new bonds between carbon and hydrogen [ 114 , 115 ].
Heterogeneous catalysts are most often used during the hydrogenation reaction. The reason for their application is the ease of separation from organic products. However, the exploit of heterogeneous catalysts during the hydrogenation reaction is not possible in the asymmetric hydrogenation reaction. In addition, homogeneous catalysts, i.e., those made of complex compounds that are based on transition metals, increase chemoselectivity and regioselectivity of the reaction.
If we use a metal complex as a catalyst during the hydrogenation reaction, this gives the opportunity to create a spatial and electronic structure of the compound. This can be achieved by choosing the right ligand. An example of the best-known hydrogenation reaction catalyst is the Wilkinson catalyst—[RhCl(PPh 3 ) 3 ] ( Figure 15 ).

Simplified formula of Wilkinson’s catalyst.
A very important enantioselective synthesis, during which the L-3,4-dihydroxylphenylalanine compound is obtained (L-DOPA), in one of the stages uses an asymmetric hydrogenation reaction. L-DOPA is a medicine applied during therapy against Parkinson’s disease. The need to obtain the appropriate product enantiomer informs us that the catalytic system of the hydrogenation mechanism must contain a chiral form of phosphine. During the synthesis reaction of L-3,4-dihydroxylphenylalanine, the catalytic system of the rhodium complex with 1,2-bis[(2-methoxyphenyl)phenylphosphine]ethane (DIPAMP) is used. Figure 16 shows a simplified formula of the chiral form of phosphine, which is a ligand in the rhodium complex [ 116 ].

Simplified formula of the chiral form of phosphine-1,2-bis[(2-methoxyphenyl)phenylphosphine] ethane.
It can be concluded that the use of phosphines as ligands in catalysts creates many opportunities to design new complexes as catalysts. Hydrogenation of esters and amides are catalyzed by these types of compounds. It is worth trying to synthesize new complexes containing other organophosphorus compounds as ligands, as well as trying to check the catalytic activity of phosphine-containing dual-core complexes.
10. Catalysis and Green Chemistry
10.1. ionic liquids—catalytic reactions.
In modern catalysis, particular attention is paid to the principles of green chemistry. Technological processes must be as environmentally friendly as possible. Ethylammonium nitrate is a chemical compound recognized as one of the first ionic liquids. This chemical compound was obtained in 1914 by Walden as a result of the neutralization of ethylamine with the help of concentrated nitric acid(V) [ 117 ]. Ionic liquids are characterized by very low vapor pressure and low melting points. The low volatility of ionic liquids is a property that caused these chemicals to be classified as green solvents. The group of compounds called ionic liquids includes: Pyridinium, tetraalkylammonium, imidazolium, and phosphonium salts. Ionic liquids can be used as solvents friendly to the environment because it does not cause environmental load. The biodegradability and ecotoxicity of ionic liquids are currently studied. Ionic liquids are involved in the transport of metals and chemical compounds in soil [ 118 ].
Imidazolium salts can be used in two-phase systems. For this reason, they are the most commonly applied ionic liquids as a catalytic reaction medium. Ionic liquids are implemented in the technological process of obtaining S-Naproxen and S-Ibuprofen anti-inflammatory drugs. Asymmetric hydrogenation is catalyzed by ruthenium complexes with S-Binap chiral phosphine carried out in systems containing ionic liquid and alcohol, e.g., methanol.
Pyridinium ionic liquids are a very good medium for the methoxycarbonylation reaction of iodobenzene catalyzed by [PdCl 2 (cod)] or Pd(0)/PVP [ 119 ]. In the Sonogashira reaction, ionic liquids were used, and thus effective catalyst recycling was possible [PdCl 2 (P(OPh) 3 ) 2 ] [ 120 ]. Processes based on the Sonogashira reaction that applies in industries are the synthesis of alkyl derivatives. These types of reactions are used in the pharmaceutical industry. In this type of reaction tetraalkylphosphonium salts are the best reaction medium. The resulting product is separated from the ionic liquid containing the catalyst using hexane extraction. By-products are removed by washing with water.
Palladium precursors with triphenylphosphine in imidazolium ionic liquids are used in the Suzuki reaction to which bromobenzene undergoes [ 121 ]. In the Suzuki reaction, the carbene complex is the active form of the catalyst. The formation of carbene complexes is characteristic of ionic liquids with particular regard to imidazolium halides. Complex compounds of ruthenium(I) and palladium(II) undergo such reactions. During the reaction, the C(2) carbon of the imidazolium ring is deprotonated and an M–C bond is formed [ 122 , 123 ].
Carbene complexes are formed in situ if the ionic liquid is a solvent in the catalytic reaction. The reactivity of carbene complexes often differs from the catalyst precursor. For this reason, there are cases where the ionic liquid is an inhibitor of catalytic activity [ 123 ]. Iodobenzene methoxycarbonylation is an example of such a reaction. It can be concluded that ionic liquids are not only solvents, but are also active components of the reaction system. Ionic liquids take part in the formation of [IL] 2 [PdX 4 ], where IL is the cation of the ionic liquid and X is the halide. [IL] 2 [PdX 4 ] complex compounds are more active in the Suzuki reaction than in the [PdCl 2 (cod)] complex [ 124 , 125 ].
C–C coupling reactions catalyzed by palladium compounds in an ammonium salt environment, i.e., [Bu 4 N]Br, show that the use of ionic liquids increases the efficiency of the reaction [ 126 ].
The application of catalysts which are coordination compounds containing ionic liquids (as ligands) was implemented in the technological process of obtaining S-naproxen and S-ibuprofen. Therefore, we conclude that it is necessary to examine the influence of geometric isomerism of catalysts on their catalytic activity and the type of reaction product obtained.
10.2. Catalysts of Organic Reactions—Metal Nanoparticles
Metal nanoparticles have diameters in the range from 1 to 50 nm. The size of the nanotube has a very large impact on their properties. First information on the use of metal nanoparticles of Pd in Heck’s reaction comes from the 90s [ 127 ]. This concerned reactions between aryl bromides and styrene, or butyl acrylate and iodobenzene [ 127 ]. Metal nanoparticles obtained by reducing metal chloride salts in the presence of tetra-N-alkylammonium cations are electrostatically stabilized [ 127 ]. The application of nanoparticles and metal complexes in various catalytic reactions is presented in Figure 17 .

The application of exemplary metal nanoparticles in various catalytic reactions.
Metal nanoparticles have some features of both homogeneous and heterogeneous catalysts. Nanoparticles can be a source of soluble metal complexes formed during a given reaction.
The complex compound undergoing a reduction reaction generates nanoparticles ( Figure 18 ). Then the nanoparticles form a metallic structure because they agglomerate. The reverse process is also possible. This happens in the case of catalytic reactions during which nanoparticles are dissolved, which in turn contributes to the formation of complex compounds. There are known cases of equilibrium between nanoparticles and palladium complexes in the catalytic system. The equilibrium is particularly common when phosphorus ligands are present in the catalytic system [ 128 ]. Metal nanoparticles are formed as a result of a reduction of complex compounds. Reduction may occur during the catalytic reaction, but it is also possible before catalysis [ 129 ].

Transformations of palladium catalysts from monomolecular to nanoparticles. This figure is adapted from [ 129 ].
Among the nanoparticles, monometallic nanoparticles are the most popular. However, bimetallic nanoparticles are becoming the subject of more and more frequent scientific research. Cheaper base metals are part of the inner sphere of bimetallic nanoparticles [ 130 ]. The base metals are covered with a layer of precious metals, e.g., platinum, palladium, and ruthenium.
Catalysis with palladium and copper nanoparticles is applied to form C–C and C–S bonds [ 131 ]. The palladium nanoparticles cause coupling of vicinal–diiodoalkenes and acrylic esters and nitriles. The copper nanoparticles are used in chemoselective reduction of N-aromatic compounds.
It can be stated that the future research approach is the application of metal nanoparticles to identify catalytic sites [ 132 ]. The identification of catalytic sites for oxygen reduction reactions is extremely important when designing new highly active catalysts containing base metals.
11. Conclusions
This article describes many chemical technology processes that involve coordination compounds as catalysts. Many organic synthesis processes in the chemical industry, including the olefin polymerization process and atom transfer radical polymerization (ATRP) process, are used to produce polymer materials. The resulting products are used in many areas of our lives in the pharmaceutical industry—for the synthesis of drugs, polyethylene production, or for the synthesis of complex organic compounds. A very important aspect in chemical technologies is the widest possible application of the principles of green chemistry—in the case of catalytic reactions involving coordination compounds, biotechnological, or organocatalytic methods are applied. An example of the described principle can be use of ultrasound to better dissolve solutions or microwave radiation for heating.
The main conclusions of this review are following:
- PdCl 2 taking part in the Wacker process forming a stoichiometric amount of acetaldehyde;
- Modernized CYCLOPOL-bis technology for caprolactam production allows for production costs to be minimized and the quality of products to be improved;
- Ni complexes with bidentate chelate ligands applied as catalysts in SHOP allow a separated C 6 –C 18 fraction to be obtained, which is used for the production of plasticizers and detergents;
- [PtCl 2 (cod)], [Pt(CH 2 =CHSiMe 2 ) 2 O 3 ], [RhCl(PPh 3 ) 3 ], [Pd 2 (dba) 3 ] are the hydrosilylation catalysts;
- Cu(I) and Fe(II) complexes with ligands such as halides, 2,2′-bipyridine, or tris(2-pyridylmethyl)amine are the catalysts in ATRP;
- [PdCl 2 (cod)] and Pd(0)/PVP are catalysts for the methoxycarbonylation reaction;
- K 2 [Fe(CO) 4 ] catalyse carbonylation;
- [Cu(2,3-pydc)(bpp)]·2.5H 2 O, [Zn(2,3-pydc)(bpp)]·2.5H 2 O, and [Cd(2,3-pydc) (bpp)(H 2 O)]·3H 2 O are metal–organic frameworks which are applied in heterogeneous catalysis;
- [IL] 2 [PdX 4 ] complex is more active than [PdCl 2 (cod)] in the Suzuki reaction.
In recent years, the development of research on the use of complex noble metal compounds as photoredox catalysts has been observed. This trend will continue for a long time because photoredox catalysis is a very useful method for activating small molecules.
Acknowledgments
Supported by the Foundation for Polish Science (FNP).
Author Contributions
Writing—original draft preparation: J.M., D.Z., J.D., and D.J.; writing—review and editing: J.M., D.Z., J.D., D.J., and B.G.; funding acquisition: J.D. and B.G. All authors have read and agreed to the published version of the manuscript.
This research was funded by National Science Centre, Poland under grant number 2015/19/N/ST5/00276.
Conflicts of Interest
The authors declare no conflict of interest. The funders had no role in the design of the study; in the collection, analyses, or interpretation of data; in the writing of the manuscript; or in the decision to publish the results.
Coordination Compounds (Chelates) in Analytical Chemistry: Solutions, Sorbents, and Nanoplatforms
- Published: 23 September 2022
- Volume 48 , pages 622–630, ( 2022 )
Cite this article
- S. N. Shtykov 1
145 Accesses
Explore all metrics
Brief characterization is given for periods of the hundred-year history of application of coordination compounds (chelates) based on chromophoric organic reagents (ORs) for the spectrophotometric and luminescent determination of metal ions. Four periods can be distinguished: detection of the efficacy, synthesis of the first ORs for metal determination, and development of the theoretical fundamentals for their action; period of targeted synthesis of ORs, systematic research of the coordination of metal compounds to ORs, and extensive use in chemical analysis; period of modification of the ORs in solution and on the sorbent surface; and period of application of liquid and solid nanoplatforms and supramolecular processes for enhancing the properties of coordination compounds of metals with organic analytical reagents. The achievements and application areas for each period are given.
This is a preview of subscription content, log in via an institution to check access.

Access this article
Price includes VAT (Russian Federation)
Instant access to the full article PDF.
Rent this article via DeepDyve
Institutional subscriptions
Similar content being viewed by others
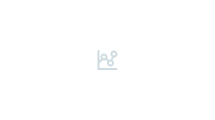
Polyoxometalates in Analytical Sciences
Fluorescence studies of metal complexes in synergistic extraction systems combining dibutyl phosphoric acid and tri-n-butyl phosphate.
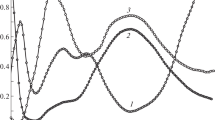
Comparative Study of the Formation of Heterometallic Diethylenetriaminpentaacetates of Cobalt(II), Nickel(II), and Copper(II) in Aqueous Solutions
Pinto, I.S.S., Neto, I.F.F., and Soares, H.M.V.M., Environ. Sci. Pollut. Res ., 2014, vol. 21, p. 11893. https://doi.org/10.1007/s11356-014-2592-6
Article CAS Google Scholar
Kryvoruchko, A., Kornilovich, B., and Yurlova, L., Desalination , 2002, vol. 144, p. 243. https://doi.org/10.1016/S0011-9164(02)00319-3
Arwidsson, Z., Elgh-Dalgren, K., Von Kronhelm, T., et al, J. Hazard. Mater ., 2010, vol. 173, p. 697. https://doi.org/10.1016/j.jhazmat.2009.08.141
Article CAS PubMed Google Scholar
Begum, Z.A., Rahman, I.M.M., Sawai, H., et al., Water Air Soil Pollut ., 2013, vol. 224, p. 1381. https://doi.org/10.1007/s11270-012-1381-4
Kontoghiorghes, G.J., Int. J. Mol. Sci ., 2020, vol. 21, p. 2499. https://doi.org/10.3390/ijms21072499
Article PubMed Central Google Scholar
Hu, A. and Wilson, J.J., Acc. Chem. Res. , 2022, vol. 55, no. 6, p. 904. https://doi.org/10.1021/acs.accounts.2c00003
Article CAS PubMed PubMed Central Google Scholar
Timerbaev, A.R., Hartinger, C.G., Aleksenko, S.S., and Keppler, B.K., Chem. Rev. , 2006, vol. 106, p. 2224. https://doi.org/10.1021/cr040704h
Kontoghiorghes, G.J. and Kontoghiorghe, C.N., Cells , 2020, vol. 9, no. 6, p. 1456. https://doi.org/10.3390/cells9061456
Article CAS PubMed Central Google Scholar
Sodhi, R.K. and Paul, S., Cancer Therapy Oncol. Int. J. , 2019, vol. 14, no. 2, p. 555883. https://doi.org/10.19080/CTOIJ.2019.14.555883
Sun, X., Sarteshnizi, R.A., Boachie, R.T., et al., Foods , 2020, vol. 9, p. 1402. https://doi.org/10.3390/foods9101402
Wu, W., Yang, Y., Sun, N., et al., Food Res. Int ., 2020, vol. 131, p. 108976. https://doi.org/10.1016/j.foodres.2020.108976
Byrne, L., Hynes, M.J., Connolly, C.D., and Murphy, R.A., Animals , 2021, vol. 11, no. 6, p. 1730. https://doi.org/10.3390/ani11061730
Li, P. and Li, H., Coord. Chem. Rev. , 2021, vol. 441, p. 213988. https://doi.org/10.1016/j.ccr.2021.213988
Inczedy, J., Analytical Applications of Complex Equilibria , Budapest: Akademiai Kiado, 1976.
Google Scholar
Marszenko, Z. and Balcerzak, M., Spektrofotometryczne metody w analizie nieorganicznej , Warszawa: Wydawnictwo Naukowe PWN, 1998.
Savvin, S.B., Shtykov, S.N., and Mikhailova, A.V., Russ. Chem. Rev. , 2006, vol. 75, no. 4, p. 341. https://doi.org/10.1070/RC2006v075n04ABEH001189
Seiny, N.R., Coulibaly, M., Yao, A.N., et al., Int. J. Electrochem. Sci ., 2016, vol. 11, p. 5342. https://doi.org/10.20964/2016.06.61
Zolotov, Yu.A., Ivanov, V.M., and Amelin, V.G., Chemical Test Methods of Analysis , Oxford: Elsevier, 2002.
Zolotov, Yu.A. Ekstraktsiya v neorganicheskom analize (Extraction in Inorganic Analysis), Moscow: MGU, 1988.
Timerbaev, A.R., Petrukhin, O.M., Alimarin, I.P., and Bol’shova, T.A., Talanta , 1991, vol. 38, no. 5, p. 467. https://doi.org/10.1016/0039-9140(91)80165-V
Slizhov, Yu.G. and Gavrilenko, M.A., Primenenie vnutrikompleksnykh soedinenii v gazovoi khromatografii (Application of Chelates in Gas Chromatography), Tomsk: Tomsk University, 2000.
Kel’tsieva, O.A., Gladilovich, V.D., and Podol’skaya, E.P., Nauchnoe Priborostroenie , 2013, vol. 23, no. 1, p. 74.
Zolotov, Yu.A., Tsisin, G.I., Morosanova, E.I., and Dmitrienko, S.G., Russ. Chem. Rev ., 2005, vol. 74, p. 37. https://doi.org/10.1070/RC2005v074n01ABEH000845
Tsizin, G.I. and Statkus, M.A., Sorbtsionnoe kontsentrirovanie mikrokomponentov v dinamicheskikh usloviyakh (Sorption Preconcentration of Trace Components under Dynamic Conditions), Moscow: Lenand, 2016.
Neudachina, L.K., Pestov, A.V., Baranova, N.V., and Startsev, V.A., Analitika i Control , 2011, vol. 15, no. 2, p. 238.
Savvin, S.B. and Strel’nikova, E.B., Zh. Anal. Khim. , 1983, vol. 38, no. 4, p.727.
CAS Google Scholar
Kul’berg, L.M., Organicheskie reaktivy v analiticheskoi khimii (Organic Reagents in Analytical Chemistry). Moscow: Goskhimizdat, 1950.
Feigl, F., Chemistry of Specific Selective and Sensitive Reactions , New York: Academic, 1949.
Berg, R. Primenenie 8-oksikhinolina v analiticheskoi kh-imii (Application of 8-Hydroxyquinoline in Analytical Chemistry), Moscow: Goskhimizdat, 1937.
Voznesenskii, S.A., Vnutrikompleksnye soedineniya i ikh znachenie dlya analiticheskoi khimii (Chelates and Their Significance for Analytical Chemistry), Moscow: GONTI, 1938.
Tananaev, N.A. Kapel’nyi metod. Kachestvennyi analiz neorganicheskikh soedinenii kapel’nym metodom (Drop Analysis. Qualitative Drop Analysis of Inorganic Compounds), Moscow: Goskhimizdat, 1954.
Kuznetsov, V.I., Usp. Khim. , 1952, vol. 21, no. 2, p. 175.
Savvin, S.B., Organicheskie reagenty gruppy arsenazo III (Organic Reagents of Arsenazo III Group), Moscow: Atomizdat, 1971.
Ivanov, V.M., Geterotsiklicheskie azotsoderzhashchie azosoedineniya (Heterocyclic Nitrogen-Containing Azo Compounds), Moscow: Nauka, 1982.
Umland, F., Janssen, A., Thierig, D., and Wünsch, G., Theorie und praktische anwendung von komplexbildnern , Frankfurt am Main: Academische Verlagsgesellschaft, 1971.
Holzbecher, Z., Divis, L., Kral, M., et al., Organická činidla v anorganické analyze , Praha: SNLT–Nakladatelstvi Technicke Literatury, 1975.
Nazarenko, V.A. and Antonovich, V.P., Trioksifluorony (Trihydroxyfluorones), Moscow: Nauka, 1973.
Perrin, D.D., Organic Complexing Reagents: Structure, Behavior, and Application to Inorganic Analysis , Sydney: Interscience, 1964.
Burger, K., Organic Reagents in Metal Analysis , Budapest: Akademiai Kiado, 1973.
Sandell, E.B. and Onishi, H., Photometric Determination of Traces of Metals , New York: Wiley, 1978, Pt. 1.
Upor, E., Mohai, M., and Novak, G.Y., Photometric Methods in Inorganic Trace Analysis , Budapest: Akadémiai Kiadó, 1985.
Pribil, R. Applications of EDTA and Related Compounds , Oxford: Pergamon, 1972.
Zolotov, Yu.A., Rossiiskii vklad v analiticheskuyu k-himiyu (Russian Contribution to Analytical Chemistry), Moscow: PRESS-BOOK.RU, 2017, p. 116.
Marov, I.N. and Kostromina, N.A., EPR i YaMR v k-himii koordinatsionnykh soedinenii (ESR and NMR in the Chemistry of Coordination Compounds), Moscow: Nauka, 1979.
Fedorov, L.A., Spektroskopiya YaMR organicheskikh analiticheskikh reagentov i ikh kompleksov s ionami metallov (NMR Spectroscopy of Organic Analytical Reagents and Their Complexes with Metal Ions), Moscow: Nauka, 1987.
Fedorov, L.A. and Ermakov, A.N., Spektroskopiya YaMR v neorganicheskom analize (NMR Spectroscopy in Inorganic Analysis), Moscow: Nauka, 1989.
Savvin, S.B. and Kuzin, E.L., Elektronnye spektry i struktura organicheskikh reagentov (Electronic Spectra and Structure of Organic Reagents), Moscow: Nauka, 1974.
Sommer, L., Ackermann, G., Thorburn Burns, D., and Savvin, S.B., Pure Appl. Chem. , 1990, vol. 62, p. 2147. https://doi.org/10.1515/iupac.62.0024
Sommer, L., Ackermann, G., and Thorburn Burns, D., Pure Appl. Chem ., 1990, vol. 62, p. 2323. https://doi.org/10.1351/pac199062122323
Korenman, I.M., Organicheskie reagenty v neorganicheskom analize (Organic Reagents in Inorganic Analysis), Moscow: Khimiya, 1980.
Dictionary of Analytical Reagents , Townshend, A., Ed., New York: CRC, 1993.
Vinogradov, A.V. and Elinson, S.V. 8-Oksikhinolin (8‑Hydroxyquinoline), Moscow: Nauka, 1979.
Peshkova, V.M., Savostina, V.M., and Ivanova, E.K, Oksimy (Oximes), Moscow: Nauka, 1977.
Peshkova, V.M. and Mel’chakova, N.V., β -Diketony (β‑Diketones), Moscow: Nauka, 1986.
Pilipenko, A.T. and Zul’figarov, O.S., Gidroksamovye kisloty (Hydroxamic Acids), Moscow: Nauka, 1989.
Zolotov, Yu.A., Ekstraktsiya vnutrikompleksnykh soedinenii (Extraction of Chelates), Moscow: Nauka, 1968.
Bozhevol’nov, E.A., Lyuminestsentnyi analiz neorganicheskikh veshchestv (Luminescence Analysis of Inorganic Compounds), Moscow: Khimiya, 1966.
Stolyarov, K.P. and Grigor’ev, N.N., Vvedenie v lyuminestsentnyi analiz neorganicheskikh veshchestv (Introduction to Luminescence Analysis of Inorganic Compounds), Leningrad: Khimiya, 1967.
Chernova, R.K., Petrova, I.K., and Kudryavtseva, L.M., Organicheskie reagenty v fluorimetricheskom analize neorganicheskikh ionov (Organic Reagents in the Fluorimetric Analysis of Inorganic Ions), Saratov: Saratov. Univ., 1982.
Burgess, J., Pure Appl. Chem. , 1991, vol. 63, no. 12, p. 1677. https://doi.org/10.1351/pac199163121677
Kuznetsov, V.V., Zh. Anal. Khim ., 1990, vol. 45, no. 9, p. 1704.
Savvin, S.B., Petrova, T.B., and Dzherayan, T.G., Zh. Anal. Khim., 1980, vol. 35, no. 8, p. 1485.
Savvin, S.B. and Petrova, T.V., Zh. Anal. Khim., 1969, vol. 24, no. 2, p. 177.
Dedkova, V.P. and Savvin, S.B., Zh. Anal. Khim., 1993, vol. 48, no. 4, p. 624.
Fialkov, Yu.A., Rastvoritel’ kak sredstvo upravleniya khimicheskim protsessom (Solvent as a Means for Control of a Chemical Reaction), Leningrad: Khimiya, 1990.
Tananaiko, M.M. and Pilipenko A.T., Raznoligandnye i raznometall’nye kompleksy i ikh primenenie v analiticheskoi khimii (Different-Ligand and Different-Metal Complexes and Their Use in Analytical Chemistry), Moscow: Khimiya, 1983.
Lukachina, V.V., Ligand-ligandnoe vzaimodeistvie i ustoichivost’ raznoligandnykh kompleksov (Ligand–Ligand Interaction and Stability of Different-Ligand Complexes), Kiev: Nauk. dumka, 1988.
Kuznetsov, V.V., Russ. Chem. Rev. , 1986, vol. 55, no. 9, p. 797. https://doi.org/10.1070/RC1986v055n09ABEH003223
Article Google Scholar
Savvin, S.B. and Mikhailova, A.V., Zh. Anal. Khim., 1996, vol. 51, no. 1, p. 49.
Savvin, S.B., Chernova, R.K., and Shtykov, S.N., Poverkhnostno-aktivnye veshchestva (Surfactants), Moscow: Nauka, 1991.
Mikhailova, A.V. and Savvin, S.B., Dokl. Akad. Nauk. , 1997, vol. 355, no. 4, p. 501.
Savvin, S.B., Marov, I.N., Chernova, R.K., et al., Zh. Anal. Khim., 1981, vol. 36, no. 5, p. 850.
Chernova, R.K., Amelin, V.G., and Shtykov, S.N., Zh . Fiz. Khim ., 1983, vol. 57, no. 6, p. 1482.
Tsepulin, V.V., Kartsev, V.N., Amelin, V.G., et al., Zh. Fiz. Khim. , 1986, vol. 60, no. 1, p. 232.
Savvin, S.B., Chernova, R.K., Belousova, V.V., et al., Zh. Anal. Khim., 1978, vol. 33, no. 8, p. 1473.
Chernova, R.K. and Shtykov, S.N., Fresenius Z. Anal. Chem ., 1989, vol. 335, p. 111.
Chernova, R.K., Shtykov, S.N., Beloliptseva, G.M., et al., Zh. Anal. Khim., 1984, vol. 39, no. 6, p. 1019.
Zaporozhets, O.A., Gaver, O.M., and Sukhan, V.V., Russ. Chem. Rev. , 1997, vol. 66, no. 7, p. 637.
Kuz’min, N.M. and Zolotov, Yu.A., Kontsentrirovanie sledov elementov (Preconcentration of Traces of Elements), Moscow: Nauka, 1988.
Zolotov, Yu.A., Tsizin, G.I., Morosanova, E.I., and Dmitrienko, S.G., Sorbtsionnoe kontsentrirovanie mikrokomponentov iz rastvorov: primenenie v neorganicheskom analize (Sorption Preconcentration of Trace Components from Solutions: Application in Inorganic Analysis), Moscow: Nauka, 2007.
Myasoedova, G.V. and Savvin, S.B., Khelatoobrazuyushchie sorbenty (Chelating Sorbents), Moscow: Nauka, 1984.
Khimiya privitykh poverkhnostnykh soedinenii (Chemistry of Grafted Surface Compounds), Lisichkin, G.V., Ed., Moscow: Fizmatlit, 2003.
Mujahid, A., Lieberzeit, P.A., and Dickert, F.L., Materials , 2010, vol. 3, p. 2196. https://doi.org/10.3390/ma3042196
Holthoff, E.L. and Bright, F.V., Acc. Chem. Res. , 2007, vol. 40, p. 756. https://doi.org/10.1021/ar700087t
Hering, R., Chelatbildende Ionenaustauscher , Berlin: Academie, 1967.
Amelin, V.G., J. Anal. Chem., 2000, vol. 55, no. 9, p. 808.
Ostrovskaya, V.M., Zh. Anal. Khim., 1977, vol. 32, no. 9, p. 1820.
Amelin, V.G. and Ivanov, V.M., J. Anal. Chem., 2000, vol. 55, no. 4, p. 367.
Amelin, V.G., Zh. Anal. Khim., 1999, vol. 54, no. 10, p. 1088.
Chernova, R.K., Shtykov, S.N., Agranovskaya, L.A., and Bubelo, V.D., USSR Inventor’s Certificate no. 1555667, Byull. Izobret. , 1990, no. 13.
Gur’eva, R.F. and Savvin, S.B., Zh. Anal. Khim., 1997, vol. 52, no. 3, p. 247.
Dedkova, V.P., Shvoeva O.P., and Savvin, S.B., Zavodsk. Lab. Diagnostika Mater. , 1998, vol. 64, no. 12, p. 7.
Dmitrienko, S.G. and Zolotov, Yu.A., Russ. Chem. Rev. , 2002, vol. 71, no. 2, p. 159.
Dmitrienko, S.G. and Apyari, V.V., Penopoliuretany: Sorbtsionnye svoistva i primenenie v khimicheskom analize (Polyurethane Foams: Sorption Properties and Application in Chemical Analysis), Moscow: Krasand, 2010.
Shtykov, S.N., Nanoob’’ekty i nanotekhnologii v khimicheskom analize (Nanoobjects and Nanotechnologies in Chemical Analysis), Shtykov, S.N., Ed., Moscow: Nauka, 2015.
Shtykov, S.N., in Nanoanalytics: Nanoobjects and Nanotechnologies in Analytical Chemistry , Shtykov, S.N., Ed., Berlin: Germany, De Gruyter, 2018, p. 32. https://doi.org/10.1515/9783110542011-001
Analiticheskaya khimiya. Instrumental’nye metody analiza (Analytical Chemistry. Instrumental Analysis), Ishchenko, A.A., Ed., Moscow: Fizmatlit, 2020, vol. 3, pt. 2.
Shtykov, S.N., J. Anal. Chem., 2000, vol. 55, no. 7, p. 608.
Shtykov, S.N., J. Anal. Chem., 2002, vol. 57, no. 10, p. 859.
Shtykov, S.N., Sumina, E.G., and Malova, M.I., Zh. Anal. Khim., 1997, vol. 52, no. 7, p. 707.
Shtykov, S.N. and Parshina, E.V., Zh. Anal. Khim., 1995, vol. 50, no. 7, p. 740.
Smirnova, T.D., Shtykov, S.N., and Zhelobitskaya, E.A., Phys. Sci. Rev ., 2019, vol. 4, no. 3, p. 20189981. https://doi.org/10.1515/psr-2018-9981
Shtykov, S.N. and Sumina, E.G., Zh. Anal. Khim., 1997, vol. 52, no. 7, p. 697.
Shtykov, S.N., Amelin, V.G., Sorokin, N.N., and Chernova, R.K., Zh. Fiz. Khim. , 1986, vol. 60, no. 2, p. 345.
Shtykov, S.N., Okunev, A.V., and Safarova, M.I., J. Anal. Chem., 2003, vol. 58, no. 11, p. 1031.
Burmistrova, N.A., Mushtakova, S.P., Shtykov, S.N., et al., Russ. Chem. Bull ., 2000, no. 8, p. 1386.
Shtykov, S.N. and Goryacheva, I.Yu., Opt. Spektrosk. , 1997, vol. 83, no. 4, p. 698.
Shtykov, S.N., Lyuminestsentnyi analiz (Luminescence Analysis), Romanovska, G.I., Ed., Moscow: Nauka, 2015.
Egorova, A.V., Skripinets, Yu.V., Aleksandrova, D.I., and Antonovich, V.P., Metody i Ob’’ekty Khim. Anal ., 2010, vol. 5, no. 4, p. 180.
Devterova, J., Kirillov, K., Nikolaev, A., et al., Materials , 2022, vol. 15, p. 1127. https://doi.org/10.3390/ma15031127
Kim, Y.J., Yang, Y.S., Ha, S.C., et al., Sens. Actuators , 2005, vol. 106, p. 189. https://doi.org/10.1016/j.snb.2004.05.056
Chew, A.K., Dallin, B.C., and van Lehn, R.C., ACS Nano , 2021, vol. 15, no. 3, p. 4534. https://doi.org/10.1021/acsnano.0c08623
Apyari, V.V., Arkhipova, V.V., Dmitrienko, S.G., and Zolotov, Yu.A., J. Anal. Chem., 2014, vol. 69, no. 1, p. 1.
Beloglazkina, E.K., Majouga, A.G., Romashkina, R.B., et al., Russ. Chem. Rev. , 2012, vol. 81, no. 1, p. 65. https://doi.org/10.1070/RC2012v081n01ABEH004158
Terenteva, E.A., Apyari, V.V., Kochuk, E.V., et al., J. Anal. Chem., 2017, vol. 72, no. 11, p. 1138.
Obare, S.O., Hollowell, R.E., and Murphy, C.J., Langmuir , 2002, vol. 18, p. 10407. https://doi.org/10.1021/la0260335
Beer, P.D., Cormode, D.P., and Davis, J.J., Chem. Commun ., 2004, no. 4, p. 414. https://doi.org/10.1039/B313658B
Dang, Y.Q., Li, H.W., Wang, B., et al., ACS Appl. Mater. Interfaces , 2009, vol. 1, no. 7, p. 1533. https://doi.org/10.1021/am9001953
Darbha, G.K., Singh, A.K., Rai, U.S., et al., J. Am. Chem. Soc. , 2008, vol. 130, p. 8038. https://doi.org/10.1021/ja801412b
Chansuvarn, W. and Imyim, A., Microchim. Acta , 2012, vol. 176, p. 574. https://doi.org/10.1007/s00604-001-0691-3
Download references
This study was supported by the Russian Science Foundation (project no. 21-13-00267).
Author information
Authors and affiliations.
Institute of Chemistry, Saratov National Research State University named after N.G. Chernyshevsky, Saratov, Russia
S. N. Shtykov
You can also search for this author in PubMed Google Scholar
Corresponding author
Correspondence to S. N. Shtykov .
Ethics declarations
The author declares that he has no conflicts of interest.
Additional information
Dedicated to Academician Yu.A. Zolotov in the year of his 90th birthday
Translated by Z. Svitanko
Rights and permissions
Reprints and permissions
About this article
Shtykov, S.N. Coordination Compounds (Chelates) in Analytical Chemistry: Solutions, Sorbents, and Nanoplatforms. Russ J Coord Chem 48 , 622–630 (2022). https://doi.org/10.1134/S1070328422100062
Download citation
Received : 21 April 2022
Revised : 05 May 2022
Accepted : 06 May 2022
Published : 23 September 2022
Issue Date : October 2022
DOI : https://doi.org/10.1134/S1070328422100062
Share this article
Anyone you share the following link with will be able to read this content:
Sorry, a shareable link is not currently available for this article.
Provided by the Springer Nature SharedIt content-sharing initiative
- metal chelates
- applications
- analytic chemistry
- Find a journal
- Publish with us
- Track your research

RSC Advances
Exploring the latest trends in chemistry, structure, coordination, and diverse applications of 1-acyl-3-substituted thioureas: a comprehensive review.

* Corresponding authors
a Department of Chemistry, Quaid-i-Azam University, Islamabad, Pakistan E-mail: [email protected]
b Departamento de Química, CEQUINOR (UNLP, CONICET-CCT La Plata), Facultad de Ciencias Exactas, Universidad Nacional de La Plata, Bv. 120 1465, La Plata 1900, Argentina
Acyl thioureas represent a privileged moiety with vast potential applicability across diverse fields, making them the subject of extensive research efforts. The inherent flexibility of thiourea facilitates the synthesis of a wide range of core structures with diverse functionalities and properties. The distinctive presence of hard and soft donor sites renders acyl thioureas inclined to act as versatile ligands, thereby engendering a diverse array of metal complexes incorporating acyl thiourea as a pivotal ligand. Extensive investigations into the synthesized acyl thioureas and their derivatives have culminated in the elucidation of their substantial potential across a spectrum of applications, spanning biological activities, materials chemistry, catalysis, and beyond. This literature review represents a continuation of our ongoing endeavor to compile comprehensive data on research endeavors concerning acyl thioureas over the past two years.
Article information

Download Citation
Permissions.

S. A. Ullah, A. Saeed, M. Azeem, M. B. Haider and M. F. Erben, RSC Adv. , 2024, 14 , 18011 DOI: 10.1039/D4RA02567A
This article is licensed under a Creative Commons Attribution 3.0 Unported Licence . You can use material from this article in other publications without requesting further permissions from the RSC, provided that the correct acknowledgement is given.
Read more about how to correctly acknowledge RSC content .
Social activity
Search articles by author, advertisements.
Academia.edu no longer supports Internet Explorer.
To browse Academia.edu and the wider internet faster and more securely, please take a few seconds to upgrade your browser .
Enter the email address you signed up with and we'll email you a reset link.
- We're Hiring!
- Help Center
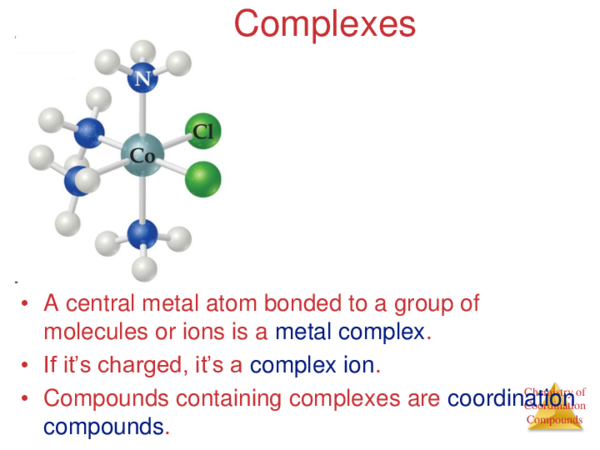
Chemistry of Coordination Compounds

Related Papers
Pakistan Everafter
A Lewis base (e-donor) donates a pair of electrons to a Lewis acid (e-acceptor) forming a coordinate covalent bond.
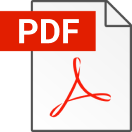
Laboratory Introduction to Bio-inorganic Chemistry
Eiichiro Ochiai
Dripta Sanyal
Kamran Mahmudov
This review describes how non-covalent interactions, such as hydrogen (inter-and intramolecular, resonance-and charge-assisted hydrogen bonding), halogen, chalcogen and pnicogen bonds, p-interactions, as well as other types of weak forces can influence and control the reactions in the synthesis, e.g., of metal-containing supramolecular networks, providing a class of highly directional stabilizing contacts that can be exploited in the design of coordination compounds. The selected recent examples show that both inter-and intramolecular non-covalent interactions can influence the formation of coordination compounds. This overview of non-covalent interactions applied for synthesis and design of coordination compounds also contributes to an improved understanding of their behavior in crystal engineering, catalysis, etc. It provides a practical consultation for synthetic chemists that are interested in exploring and further developing non-covalent interactions assisted synthesis and design of coordination compounds.
احمد حسن عبدالسلا م نور الدين
Editor CSRL
This mini-review discusses the different types of ligands, polytopic ligands and metal organic ligands (MOLs) of 21 years with particular focus on the one pot synthesis of different homo and hetero polynuclear complexes using MOLs. Amongt the different methods used for the synthesis of polynuclear complexes the complexes used as ligand is the most successful method. The properties of complexes originating due to metal ions may get enhanced in polynuclear complexes. Moreover, the interaction between the metal ions in such complexes may give rise to new properties. The tailor made polynuclear complexes synthesized by suitable methods with expected structure and properties can be applied in different fields.
Journal of Coordination Chemistry
Alexander Sadimenko
Max Kopylovich , Kamran Mahmudov
In this chapter we have illustrated the significance of the halogen bonds as a driven force of reaction in the synthesis and design of coordination compounds. Additionally, cooperation of halogen, hydrogen, and coordination bonds in the halogen bond‐assisted syntheses, some parameters of halogen bonds and their relations to properties of the metal ions were discussed.
Bulletin of Japan Society of Coordination Chemistry
Pierre Braunstein
This Account will focus on some recent aspects of the research performed in the author’s laboratory. Selected examples of complexes in which the ligands play an essential role in determining the molecular properties will be examined. Concerning the applications of these complexes, we will limit ourselves to some aspects related to the catalytic oligomerization of ethylene. In the search for such catalysts, we found that deprotonation of N,OH ligands chelated to Ni(II) with NaH can give rise to a range of heterometallic Na-Ni polynuclear complexes. In addition to their catalytic relevance, their magnetic properties were also investigated and have revealed in some cases Single Molecule Magnet behaviour. The diverse functions that co-catalysts may exert will be illustrated by the characterization of Cr(III) complexes obtained by reaction of fac-[Cr(NpyPNpy)Cl3] with typical co-catalysts such as MAO, EtAlCl2, AlMe3 and AlEt3. We will also discuss some polynuclear and cluster chemistry w...
RELATED PAPERS
AIMAN HAKIM MOHAMED ZULMAZI
ENCARNACIÓN MANRESA-MARHUENDA
Simbiótica. Revista Eletrônica
Simbiótica Revista Eletrônica
Advanced Powder Technology
Saeid Azizian
Nature Communications
Franco Pirajno
Juha-Matti Katajajuuri
Kris Francisco
Nano Letters
Maciej Paszewski
European journal of public health
Giulia Silvestrini
Lymphoma and Chronic Lymphocytic Leukemias
Majalah Bahana Penerbit ANDI Offset
Adi S U H E N R A Sigiro
Ethnography and Education
Pat Thomson
Molecular and Biochemical Parasitology
Arturo Rojo-Domínguez
Revista Caminhos de Geografia
Adriana Salay Leme , Livia Cangiano Antipon
International Journal of Molecular Sciences
Vilma Ratautaite
Progress in Environmental Geography
Manuel Prieto
Francisco Concepcion
Russell Karow
Acta Societatis Botanicorum Poloniae
Małgorzata Garnczarska
Surgery for Obesity and Related Diseases
Farah Husain
Age and Ageing
Ngaire Kerse
RELATED TOPICS
- We're Hiring!
- Help Center
- Find new research papers in:
- Health Sciences
- Earth Sciences
- Cognitive Science
- Mathematics
- Computer Science
- Academia ©2024
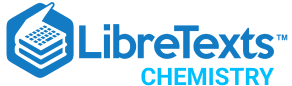
- school Campus Bookshelves
- menu_book Bookshelves
- perm_media Learning Objects
- login Login
- how_to_reg Request Instructor Account
- hub Instructor Commons
Margin Size
- Download Page (PDF)
- Download Full Book (PDF)
- Periodic Table
- Physics Constants
- Scientific Calculator
- Reference & Cite
- Tools expand_more
- Readability
selected template will load here
This action is not available.
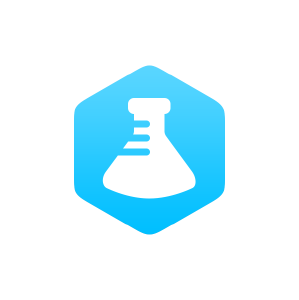
24.1: Werner’s Theory of Coordination Compounds
- Last updated
- Save as PDF
- Page ID 24350

\( \newcommand{\vecs}[1]{\overset { \scriptstyle \rightharpoonup} {\mathbf{#1}} } \)
\( \newcommand{\vecd}[1]{\overset{-\!-\!\rightharpoonup}{\vphantom{a}\smash {#1}}} \)
\( \newcommand{\id}{\mathrm{id}}\) \( \newcommand{\Span}{\mathrm{span}}\)
( \newcommand{\kernel}{\mathrm{null}\,}\) \( \newcommand{\range}{\mathrm{range}\,}\)
\( \newcommand{\RealPart}{\mathrm{Re}}\) \( \newcommand{\ImaginaryPart}{\mathrm{Im}}\)
\( \newcommand{\Argument}{\mathrm{Arg}}\) \( \newcommand{\norm}[1]{\| #1 \|}\)
\( \newcommand{\inner}[2]{\langle #1, #2 \rangle}\)
\( \newcommand{\Span}{\mathrm{span}}\)
\( \newcommand{\id}{\mathrm{id}}\)
\( \newcommand{\kernel}{\mathrm{null}\,}\)
\( \newcommand{\range}{\mathrm{range}\,}\)
\( \newcommand{\RealPart}{\mathrm{Re}}\)
\( \newcommand{\ImaginaryPart}{\mathrm{Im}}\)
\( \newcommand{\Argument}{\mathrm{Arg}}\)
\( \newcommand{\norm}[1]{\| #1 \|}\)
\( \newcommand{\Span}{\mathrm{span}}\) \( \newcommand{\AA}{\unicode[.8,0]{x212B}}\)
\( \newcommand{\vectorA}[1]{\vec{#1}} % arrow\)
\( \newcommand{\vectorAt}[1]{\vec{\text{#1}}} % arrow\)
\( \newcommand{\vectorB}[1]{\overset { \scriptstyle \rightharpoonup} {\mathbf{#1}} } \)
\( \newcommand{\vectorC}[1]{\textbf{#1}} \)
\( \newcommand{\vectorD}[1]{\overrightarrow{#1}} \)
\( \newcommand{\vectorDt}[1]{\overrightarrow{\text{#1}}} \)
\( \newcommand{\vectE}[1]{\overset{-\!-\!\rightharpoonup}{\vphantom{a}\smash{\mathbf {#1}}}} \)
Learning Objectives
- To know the most common structures observed for metal complexes.
- To predict the relative stabilities of metal complexes with different ligands
One of the most important properties of metallic elements is their ability to act as Lewis acids that form complexes with a variety of Lewis bases. A metal complex consists of a central metal atom or ion that is bonded to one or more ligands (from the Latin ligare, meaning “to bind”), which are ions or molecules that contain one or more pairs of electrons that can be shared with the metal. Metal complexes can be neutral, such as Co(NH 3 ) 3 Cl 3 ; positively charged, such as [Nd(H 2 O) 9 ] 3+ ; or negatively charged, such as [UF 8 ] 4− . Electrically charged metal complexes are sometimes called complex ions . A coordination compound contains one or more metal complexes.
Coordination compounds are important for at least three reasons. First, most of the elements in the periodic table are metals, and almost all metals form complexes, so metal complexes are a feature of the chemistry of more than half the elements. Second, many industrial catalysts are metal complexes, and such catalysts are steadily becoming more important as a way to control reactivity. For example, a mixture of a titanium complex and an organometallic compound of aluminum is the catalyst used to produce most of the polyethylene and polypropylene “plastic” items we use every day. Finally, transition-metal complexes are essential in biochemistry. Examples include hemoglobin, an iron complex that transports oxygen in our blood; cytochromes, iron complexes that transfer electrons in our cells; and complexes of Fe, Zn, Cu, and Mo that are crucial components of certain enzymes, the catalysts for all biological reactions.
History of the Coordination Compounds
Coordination compounds have been known and used since antiquity; probably the oldest is the deep blue pigment called Prussian blue: \(\ce{KFe2(CN)6}\). The chemical nature of these substances, however, was unclear for a number of reasons. For example, many compounds called “double salts” were known, such as \(\ce{AlF3·3KF}\), \(\ce{Fe(CN)2·4KCN}\), and \(\ce{ZnCl2·2CsCl}\), which were combinations of simple salts in fixed and apparently arbitrary ratios. Why should \(\ce{AlF3·3KF}\) exist but not \(\ce{AlF3·4KF}\) or \(\ce{AlF3·2KF}\)? And why should a 3:1 KF:AlF3 mixture have different chemical and physical properties than either of its components? Similarly, adducts of metal salts with neutral molecules such as ammonia were also known—for example, \(\ce{CoCl3·6NH3}\), which was first prepared sometime before 1798. Like the double salts, the compositions of these adducts exhibited fixed and apparently arbitrary ratios of the components. For example, \(\ce{CoCl3·6NH3}\), \(\ce{CoCl3·5NH3}\), \(\ce{CoCl3·4NH3}\), and \(\ce{CoCl3·3NH3}\) were all known and had very different properties, but despite all attempts, chemists could not prepare \(\ce{CoCl3·2NH3}\) or \(\ce{CoCl3·NH3}\).
Although the chemical composition of such compounds was readily established by existing analytical methods, their chemical nature was puzzling and highly controversial. The major problem was that what we now call valence (i.e., the oxidation state) and coordination number were thought to be identical. As a result, highly implausible (to modern eyes at least) structures were proposed for such compounds, including the “Chattanooga choo-choo” model for CoCl 3 ·4NH 3 shown here.
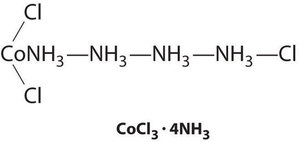
The modern theory of coordination chemistry is based largely on the work of Alfred Werner (1866–1919; Nobel Prize in Chemistry in 1913). In a series of careful experiments carried out in the late 1880s and early 1890s, he examined the properties of several series of metal halide complexes with ammonia. For example, five different “adducts” of ammonia with PtCl 4 were known at the time: PtCl 4 ·nNH 3 (n = 2–6). Some of Werner’s original data on these compounds are shown in Table \(\PageIndex{1}\). The electrical conductivity of aqueous solutions of these compounds was roughly proportional to the number of ions formed per mole, while the number of chloride ions that could be precipitated as AgCl after adding Ag + (aq) was a measure of the number of “free” chloride ions present. For example, Werner’s data on PtCl 4 ·6NH 3 in Table \(\PageIndex{1}\) showed that all the chloride ions were present as free chloride. In contrast, PtCl 4 ·2NH 3 was a neutral molecule that contained no free chloride ions.
Alfred Werner (1866–1919)
Werner, the son of a factory worker, was born in Alsace. He developed an interest in chemistry at an early age, and he did his first independent research experiments at age 18. While doing his military service in southern Germany, he attended a series of chemistry lectures, and he subsequently received his PhD at the University of Zurich in Switzerland, where he was appointed professor of chemistry at age 29. He won the Nobel Prize in Chemistry in 1913 for his work on coordination compounds, which he performed as a graduate student and first presented at age 26. Apparently, Werner was so obsessed with solving the riddle of the structure of coordination compounds that his brain continued to work on the problem even while he was asleep. In 1891, when he was only 25, he woke up in the middle of the night and, in only a few hours, had laid the foundation for modern coordination chemistry.
These data led Werner to postulate that metal ions have two different kinds of valence: (1) a primary valence ( oxidation state ) that corresponds to the positive charge on the metal ion and (2) a secondary valence ( coordination number ) that is the total number of ligand-metal bonds bound to the metal ion. If \(\ce{Pt}\) had a primary valence of 4 and a secondary valence of 6, Werner could explain the properties of the \(\ce{PtCl4·NH3}\) adducts by the following reactions, where the metal complex is enclosed in square brackets:
\[\begin{align*} \mathrm{[Pt(NH_3)_6]Cl_4} &\rightarrow \mathrm{[Pt(NH_3)_6]^{4+}(aq)+4Cl^-(aq)} \\[4pt] \mathrm{[Pt(NH_3)_5Cl]Cl_3} &\rightarrow \mathrm{[Pt(NH_3)_5Cl]^{3+}(aq) +3Cl^-(aq)}\\[4pt] \mathrm{[Pt(NH_3)_4Cl_2]Cl_2} &\rightarrow \mathrm{[Pt(NH_3)_4Cl_2]^{2+}(aq) +2Cl^-(aq)}\\[4pt] \mathrm{[Pt(NH_3)_3Cl_3]Cl} &\rightarrow \mathrm{[Pt(NH_3)_3Cl_3]^+(aq) + Cl^-(aq)}\\[4pt] \mathrm{[Pt(NH_3)_2Cl_4]} &\rightarrow \mathrm{[Pt(NH_3)_2Cl_4]^0(aq)} \end{align*} \label{23.9}\]
Further work showed that the two missing members of the series—[Pt(NH 3 )Cl 5 ] − and [PtCl 6 ] 2− —could be prepared as their mono- and dipotassium salts, respectively. Similar studies established coordination numbers of 6 for Co 3 + and Cr 3 + and 4 for Pt 2 + and Pd 2 + .
Werner’s studies on the analogous Co 3 + complexes also allowed him to propose a structural model for metal complexes with a coordination number of 6. Thus he found that [Co(NH 3 ) 6 ]Cl 3 (yellow) and [Co(NH 3 ) 5 Cl]Cl 2 (purple) were 1:3 and 1:2 electrolytes. Unexpectedly, however, two different [Co(NH 3 ) 4 Cl 2 ]Cl compounds were known: one was red, and the other was green (Figure \(\PageIndex{1a}\)). Because both compounds had the same chemical composition and the same number of groups of the same kind attached to the same metal, there had to be something different about the arrangement of the ligands around the metal ion. Werner’s key insight was that the six ligands in [Co(NH 3 ) 4 Cl 2 ]Cl had to be arranged at the vertices of an octahedron because that was the only structure consistent with the existence of two, and only two, arrangements of ligands (Figure \(\PageIndex{1b}\). His conclusion was corroborated by the existence of only two different forms of the next compound in the series: Co(NH 3 ) 3 Cl 3 .
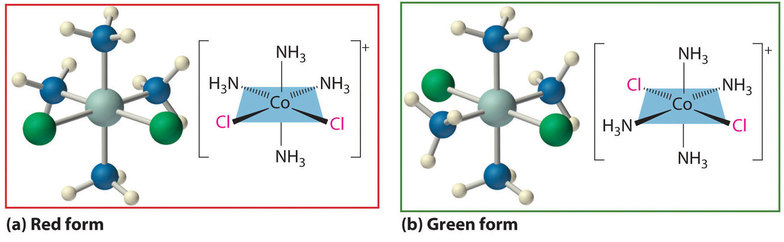
Example \(\PageIndex{1}\)
In Werner’s time, many complexes of the general formula MA 4 B 2 were known, but no more than two different compounds with the same composition had been prepared for any metal. To confirm Werner’s reasoning, calculate the maximum number of different structures that are possible for six-coordinate MA 4 B 2 complexes with each of the three most symmetrical possible structures: a hexagon, a trigonal prism, and an octahedron. What does the fact that no more than two forms of any MA 4 B 2 complex were known tell you about the three-dimensional structures of these complexes?
Given : three possible structures and the number of different forms known for MA 4 B 2 complexes
Asked for : number of different arrangements of ligands for MA 4 B 2 complex for each structure
Sketch each structure, place a B ligand at one vertex, and see how many different positions are available for the second B ligand.
The three regular six-coordinate structures are shown here, with each coordination position numbered so that we can keep track of the different arrangements of ligands. For each structure, all vertices are equivalent. We begin with a symmetrical MA 6 complex and simply replace two of the A ligands in each structure to give an MA 4 B 2 complex:
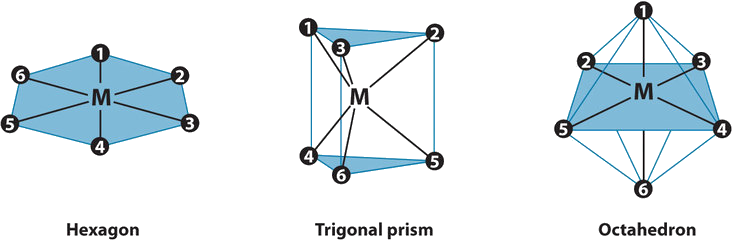
For the hexagon, we place the first B ligand at position 1. There are now three possible places for the second B ligand: at position 2 (or 6), position 3 (or 5), or position 4. These are the only possible arrangements. The (1, 2) and (1, 6) arrangements are chemically identical because the two B ligands are adjacent to each other. The (1, 3) and (1, 5) arrangements are also identical because in both cases the two B ligands are separated by an A ligand.
Turning to the trigonal prism, we place the first B ligand at position 1. Again, there are three possible choices for the second B ligand: at position 2 or 3 on the same triangular face, position 4 (on the other triangular face but adjacent to 1), or position 5 or 6 (on the other triangular face but not adjacent to 1). The (1, 2) and (1, 3) arrangements are chemically identical, as are the (1, 5) and (1, 6) arrangements.
In the octahedron, however, if we place the first B ligand at position 1, then we have only two choices for the second B ligand: at position 2 (or 3 or 4 or 5) or position 6. In the latter, the two B ligands are at opposite vertices of the octahedron, with the metal lying directly between them. Although there are four possible arrangements for the former, they are chemically identical because in all cases the two B ligands are adjacent to each other.
The number of possible MA 4 B 2 arrangements for the three geometries is thus: hexagon, 3; trigonal prism, 3; and octahedron, 2. The fact that only two different forms were known for all MA 4 B 2 complexes that had been prepared suggested that the correct structure was the octahedron but did not prove it. For some reason one of the three arrangements possible for the other two structures could have been less stable or harder to prepare and had simply not yet been synthesized. When combined with analogous results for other types of complexes (e.g., MA 3 B 3 ), however, the data were best explained by an octahedral structure for six-coordinate metal complexes.
Exercise \(\PageIndex{1}\)
Determine the maximum number of structures that are possible for a four-coordinate MA 2 B 2 complex with either a square planar or a tetrahedral symmetrical structure.
square planar, 2; tetrahedral, 1
Structures of Metal Complexes
The coordination numbers of metal ions in metal complexes can range from 2 to at least 9. In general, the differences in energy between different arrangements of ligands are greatest for complexes with low coordination numbers and decrease as the coordination number increases. Usually only one or two structures are possible for complexes with low coordination numbers, whereas several different energetically equivalent structures are possible for complexes with high coordination numbers (n > 6). The following presents the most commonly encountered structures for coordination numbers 2–9. Many of these structures should be familiar to you from our discussion of the valence-shell electron-pair repulsion ( VSEPR ) model because they correspond to the lowest-energy arrangements of n electron pairs around a central atom.
Compounds with low coordination numbers exhibit the greatest differences in energy between different arrangements of ligands.
Coordination Number 2
Although it is rare for most metals, this coordination number is surprisingly common for d 10 metal ions, especially Cu + , Ag + , Au + , and Hg 2 + . An example is the [Au(CN) 2 ] − ion, which is used to extract gold from its ores. As expected based on VSEPR considerations, these complexes have the linear L–M–L structure shown here.
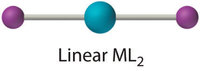
Coordination Number 3
Although it is also rare, this coordination number is encountered with d 10 metal ions such as Cu + and Hg 2 + . Among the few known examples is the HgI 3 − ion. Three-coordinate complexes almost always have the trigonal planar structure expected from the VSEPR model.

Coordination Number 4
Two common structures are observed for four-coordinate metal complexes: tetrahedral and square planar. The tetrahedral structure is observed for all four-coordinate complexes of nontransition metals, such as [BeF 4 ] 2− , and d 10 ions, such as [ZnCl 4 ] 2− . It is also found for four-coordinate complexes of the first-row transition metals, especially those with halide ligands (e.g., [FeCl 4 ] − and [FeCl 4 ] 2− ). In contrast, square planar structures are routinely observed for four-coordinate complexes of second- and third-row transition metals with d 8 electron configurations, such as Rh + and Pd 2 + , and they are also encountered in some complexes of Ni 2 + and Cu 2 + .

Coordination Number 5
This coordination number is less common than 4 and 6, but it is still found frequently in two different structures: trigonal bipyramidal and square pyramidal. Because the energies of these structures are usually rather similar for most ligands, many five-coordinate complexes have distorted structures that lie somewhere between the two extremes.
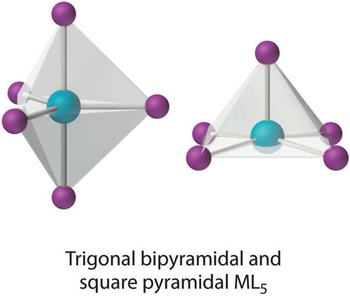
Coordination Number 6
This coordination number is by far the most common. The six ligands are almost always at the vertices of an octahedron or a distorted octahedron. The only other six-coordinate structure is the trigonal prism, which is very uncommon in simple metal complexes.
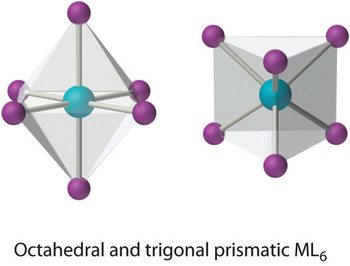
Coordination Number 7
This relatively uncommon coordination number is generally encountered for only large metals (such as the second- and third-row transition metals, lanthanides, and actinides). At least three different structures are known, two of which are derived from an octahedron or a trigonal prism by adding a ligand to one face of the polyhedron to give a “capped” octahedron or trigonal prism. By far the most common, however, is the pentagonal bipyramid.
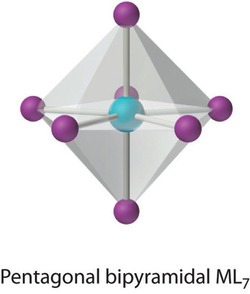
Coordination Number 8
This coordination number is relatively common for larger metal ions. The simplest structure is the cube, which is rare because it does not minimize interligand repulsive interactions. Common structures are the square antiprism and the dodecahedron, both of which can be generated from the cube.

Coordination Number 9
This coordination number is found in larger metal ions, and the most common structure is the tricapped trigonal prism, as in [Nd(H 2 O) 9 ] 3+ .

Key Takeaways
- Coordination compounds are a major feature of the chemistry of over half the elements.
- Coordination compounds have important roles as industrial catalysts in controlling reactivity, and they are essential in biochemical processes.
Transition metals form metal complexes, polyatomic species in which a metal ion is bound to one or more ligands, which are groups bound to a metal ion. Complex ions are electrically charged metal complexes, and a coordination compound contains one or more metal complexes. Metal complexes with low coordination numbers generally have only one or two possible structures, whereas those with coordination numbers greater than six can have several different structures. Coordination numbers of two and three are common for d 10 metal ions. Tetrahedral and square planar complexes have a coordination number of four; trigonal bipyramidal and square pyramidal complexes have a coordination number of five; and octahedral complexes have a coordination number of six. At least three structures are known for a coordination number of seven, which is generally found for only large metal ions. Coordination numbers of eight and nine are also found for larger metal ions.
coordination compounds Recently Published Documents
Total documents.
- Latest Documents
- Most Cited Documents
- Contributed Authors
- Related Sources
- Related Keywords
Reversible single-crystal-to-single-crystal transformations in coordination compounds induced by external stimuli
Study of triple system calcium iodide-hmta-water.
Research relevance: synthesis, physicochemical studies of complexes bioactivity with salts of biometals and organic ligands are of great interest for bio-coordination chemistry. Materials and research methods: isothermal method was used to study heterogeneous equilibria at 25 °C. The concentration limits of compounds existence and the types of their solubility have been established. Research objectives: to investigate compounds of hexamethylenetetramine with salts of biometals with organic ligands in order to reduce toxicity, increase the bioactivity and bioavailability of coordination compounds. Research results: concentration limits of compounds existence and types of their solubility have been established. Conclusions: in obtained complexes, HMTA ligand contains four equivalent electron donor nitrogen atoms.
Ultrahard magnetism from mixed-valence dilanthanide complexes with metal-metal bonding
Magnetic effects of lanthanide bonding Lanthanide coordination compounds have attracted attention for their persistent magnetic properties near liquid nitrogen temperature, well above alternative molecular magnets. Gould et al . report that introducing metal-metal bonding can enhance coercivity. Reduction of iodide-bridged terbium or dysprosium dimers resulted in a single electron bond between the metals, which enforced alignment of the other valence electrons. The resultant coercive fields exceeded 14 tesla below 50 and 60 kelvin for the terbium and dysprosium compounds, respectively. —JSY
Two New Photochromic Coordination Compounds with Thiazolothiazole Extended Viologen and Two Different Auxiliary Ligands
Changes in micro- and central hemodynamic parameters in rats under the action of acetylsalicylic acid and its coordination compounds with metals.
Introduction. The cutaneous blood circulation is a representative model both for studying the mechanisms of vascular diseases and for assessing the current state of the central hemodynamics in preclinical researches of various chemical compounds. Aim. The changes in the parameters of cutaneous microcirculation and central hemodynamics (heart rate and blood pressure) were studied in the animals under the action of acetylsalicylic acid and its coordination compounds with cations of cobalt, zinc, nickel and manganese at a dose of 20 mg/kg. Materials and methods. The research was conducted using the laser Doppler flowmetry method on the Lazma-MC device (manufactured by RPE Lazma, Russia) and the NIBP200A system (Biopac Systems, Inc., USA). Results. The study shows that animals develop bradycardia, and microcirculation and central hemodynamics change in two ways after the introduction of acetylsalicylic acid and the tested metal salicylates. These ways are hypotension-related hyperemia (acetylsalicylic acid and cobalt salicylate) and ischemia (zinc, nickel and manganese salicylates) associated with hypertension. Conclusion. The obtained data confirm the cardiotropic activity of new coordination compounds. The data also prove that the generation of the acetylsalicylic acid derivatives allows enhancing it physiological effects, as well as obtaining completely new molecules. The molecules are different from the precursor one and are necessary for the production of effective drugs.
Cobalt(II)‐Hexaazatriphenylene Hexacarbonitrile Coordination Compounds Based Cathode Materials with High Capacity and Long Cycle Stability
Two new bis(pyridine)-bis(amide)-based copper(ii) coordination compounds for the electrochemical detection of trace cr(vi) and efficient electrocatalytic oxygen evolution.
Abstract Two new metal-organic compounds (MOCs) [Cu(L)0.5(3-nba)2] (1) and [Cu(L)(2,5-tdc)] (2) have been hydrothermally synthesized by employing the ligand N,N′-di(3-pyridyl)adipoamide (L) and two carboxylic acids (3-Hnba = 3-nitrobenzoic acid, 2,5-H2tdc = 2,5-thiophenedicarboxylic acid) as ligands. Compound 1 displays a metal-organic chain-like structure formed by the {Cu2(3-nba)4} double-paddle wheel units and the µ 2-bridging L ligands. The adjacent polymeric chains form a supramolecular layered structure through hydrogen bonding. Compound 2 shows a 3D metal-organic polymeric framework derived from Cu-L layers and µ 2-bridging 2,5-tdc ligands, which presents a 3,5-connected {4.62}{4.66.83} topology. The electrochemical and electrocatalytic behavior of the two compounds has been studied in detail. Carbon paste working electrodes modified with compounds 1 and 2 can be used as highly selective sensors for detecting traces Cr(VI). Both electrodes show also electrocatalytic performance in oxygen evolution reactions (OERs).
NIR-OLED structures based on lanthanide coordination compounds: synthesis and luminescent properties
Crystal structures of 3/4-pyridyl-based thiosemicarbazones and related cu and ni coordination compounds.
In this investigation, the crystal structures of the thio-ligands 3-formylpyridine 4-phenylthiosemicarbazone (C13H12N4S, 1) and 4-benzoylpyridine 4-ethylthiosemicarbazone (C15H16N4S, 2), and of two new coordination compounds, chlorido(3-formylpyridine 4-phenylthiosemicarbazone-κS)bis(triphenylphosphane-κP)copper(I) acetonitrile monosolvate, [CuCl(C13H12N4S)(C18H15P)2]·CH3CN, 3, and bis(3-formylpyridine 4-ethylthiosemicarbazonato-κ2 N 1,S)nickel(II), [Ni(C9H11N4S)2], 4, are reported. In complex 3, the thio-ligand coordinates in a neutral form to the Cu atom through its S-donor atom, and in complex 4, the anionic thio-ligand chelates to the Ni atom through N- and S-donor atoms. The geometry of complex 3 is distorted tetrahedral [bond angles 99.70 (5)–123.23 (5)°], with the P—Cu—P bond angle being the largest, while that of complex 4 is square planar, with trans-S—Ni—S and N—Ni—N bond angles of 180°.
Controlled API release in azelaic acid coordination compounds with potential dermatological properties
Export citation format, share document.

IMAGES
VIDEO
COMMENTS
Coordination chemistry is the study of compounds that have a central atom (often metallic) surrounded by molecules or anions, known as ligands. ... Research Highlights 26 Feb 2024 Nature Reviews ...
The Journal of Coordination Chemistry publishes the results of original investigations of coordination complexes, loosely defined as the interactions of organic or inorganic ligands with metal centres. Original investigations may involve syntheses, structures, physical and chemical properties, kinetics and mechanisms of reactions, calculations and applications of coordination compounds.
This publication presents the new trends and opportunities for further development of coordination compounds used in the chemical industry. The review describes the influence of various physicochemical factors regarding the coordination relationship (for example, steric hindrance, electron density, complex geometry, ligand), which condition technological processes.
The journal offers rapid publication of research articles, reviews and Short communications on topics of current interest and importance in coordination chemistry.The term coordination chemistry is interpreted broadly, and includes aspects of organometallic, supramolecular, theoretical, and bioinorganic chemistry.The journal also publishes articles on catalysis, materials chemistry and metal ...
The journal offers rapid publication of review articles on topics of current interest and importance in coordination chemistry.The term coordination chemistry is interpreted broadly, and includes aspects of organometallic, supramolecular, theoretical, and bioinorganic chemistry.The journal also publishes review articles on catalysis, materials chemistry and metal-organic frameworks which focus ...
1. Introduction. Coordination compounds play a vital role in modern inorganic and bioinorganic chemistry. They are considered the backbone of various chemical industries due to their wide application in different areas such as metal sensing, bioimaging, drug delivery, chemosensor, pharmacological and medicinal chemistry [1].The substantial developments of new concepts and different bonding ...
Explore the latest full-text research PDFs, articles, conference papers, preprints and more on COORDINATION CHEMISTRY. Find methods information, sources, references or conduct a literature review ...
Explore the latest full-text research PDFs, articles, conference papers, preprints and more on COORDINATION COMPOUNDS. Find methods information, sources, references or conduct a literature review ...
A unique feature of the work carried out in the Collaborative Research Center 3MET continues to be its emphasis on innovative, advanced experimental methods which hyphenate mass-selection with further analytical tools such as laser spectroscopy for the study of isolated molecular ions. This allows to probe the intrinsic properties of the species of interest free of perturbing solvent or matrix ...
The third period of research (mid-1980s-1990s) focused on modification of the properties of ORs and coordination compounds they form. This was due to a weighty reason. A comparison of the results of extensive studies of organic reagents and conditions of complex formation with metal ions showed that, despite the theoretically infinite options for the design of new organic molecules that can ...
The 28th continuation of this series was held between 5 and 10 June 2022 at Smolenice Castle, Slovakia, and was named "Progressive Trends in Coordination, Bioinorganic and Applied Inorganic Chemistry". It hosted 39 scientists from Slovakia and 46 scientists from 14 European, North American, and Asian countries ( Figure 1 ).
Coordination in Compounds. Angela Ralli. Summary. Compounds are generally distinguished into those which involve a dependency. (subordinate and attributive) relation of one constituent upon the ...
Cyclometalated Pt (II)C^N phosphors bearing a bis (diphenylphorothioyl) amide ligand: synthesis and photophysical properties. Lige Qiao et al. Article | Published online: 23 May 2024. View all latest articles. Explore the current issue of Journal of Coordination Chemistry, Volume 77, Issue 5-6, 2024.
A milestone in the evolution of coordination chemistry is the revolutionary theory by Alfred Werner in 1893, which laid foundations to modern coordination chemistry. Today, IUPAC defines a coordination compound as any compound that is composed of a central atom, usually that of a metal, to which is attached a surrounding array of other atoms or ...
2. Coordination Chemistry and Its Theories and Characteristics The coordination chemistry refers to a discipline whose research objects are metal atoms, ions, inorganic and organic ions or coordination compounds characteristics obtained by the reaction in molecules and their bonding, structure, reaction, classification and preparation.
Oxford Research Encyclopedia of Linguistics. Compounds are generally divided in those that involve a dependency (subordinate and attributive) relation of one constituent upon the other and those where there is coordination, for which there is much controversy on delimiting the exact borders. This article offers an overview of compounds ...
This literature review represents a continuation of our ongoing endeavor to compile comprehensive data on research endeavors concerning acyl thioureas over the past two years. ... Exploring the latest trends in chemistry, structure, coordination, and diverse applications of 1-acyl-3-substituted thioureas: a comprehensive review S. A. Ullah ...
The coordination chemistry of polyfunctional ligands, ... This is a short review of recent research papers that focus on the synthesis, reactivity and properties of coordination compounds of ...
Chemistry of Coordination Compounds fWerner's Theory • This approach correctly predicts there would be two forms of CoCl3 ∙ 4 NH3. The formula would be written [Co (NH3)4Cl2]Cl. One of the two forms has the two chlorines next to each other. The other has the chlorines opposite each other.
This is a good point to start the synthesis of the iron complex. While you are waiting for the volume to be reduced, set up an ice bath. Obtain ~5 mL of methanol and put it in a small beaker or test tube in your ice bath. You will use the ice cold methanol to rinse your crystals in the final step of this synthesis.
The application of electrically conductive 1D coordination polymers (1D CPs) in nanoelectronic molecular recognition is theoretically promising yet rarely explored due to the challenges in their synthesis and optimization of electrical properties. In this regard, two tetrathiafulvalene-based 1D CPs, namely [Co(m-H2TTFTB)(DMF)2(H2O)]n (Co-m-TTFTB), and {[Ni(m-H2TTFTB)(CH3CH2OH)1.5(H2O)1.5 ...
Coordination Compounds. R.D. Gillard, in Encyclopedia of Physical Science and Technology (Third Edition), 2003 VI Therapeutic Metal Complexes. Whether a solution of a coordination compound is ingested or injected, it enters an aqueous medium and, depending on concentration, temperature, ionic strength, pH, and so forth, will come to equilibrium with the environment.
This publication presents the new trends and opportunities for further development of coordination compounds used in the chemical industry. The review describes the influence of various ...
0. These data led Werner to postulate that metal ions have two different kinds of valence: (1) a primary valence ( oxidation state) that corresponds to the positive charge on the metal ion and (2) a secondary valence ( coordination number) that is the total number of ligand-metal bonds bound to the metal ion. If Pt Pt had a primary valence of 4 ...
Coordination Compounds . Organic Ligands . Isothermal Method . Physicochemical Studies . Donor Nitrogen . Equivalent Electron . Concentration Limits. Research relevance: synthesis, physicochemical studies of complexes bioactivity with salts of biometals and organic ligands are of great interest for bio-coordination chemistry.