Suggestions or feedback?

MIT News | Massachusetts Institute of Technology
- Machine learning
- Social justice
- Black holes
- Classes and programs
Departments
- Aeronautics and Astronautics
- Brain and Cognitive Sciences
- Architecture
- Political Science
- Mechanical Engineering
Centers, Labs, & Programs
- Abdul Latif Jameel Poverty Action Lab (J-PAL)
- Picower Institute for Learning and Memory
- Lincoln Laboratory
- School of Architecture + Planning
- School of Engineering
- School of Humanities, Arts, and Social Sciences
- Sloan School of Management
- School of Science
- MIT Schwarzman College of Computing
Powering the energy transition with better storage
Press contact :.
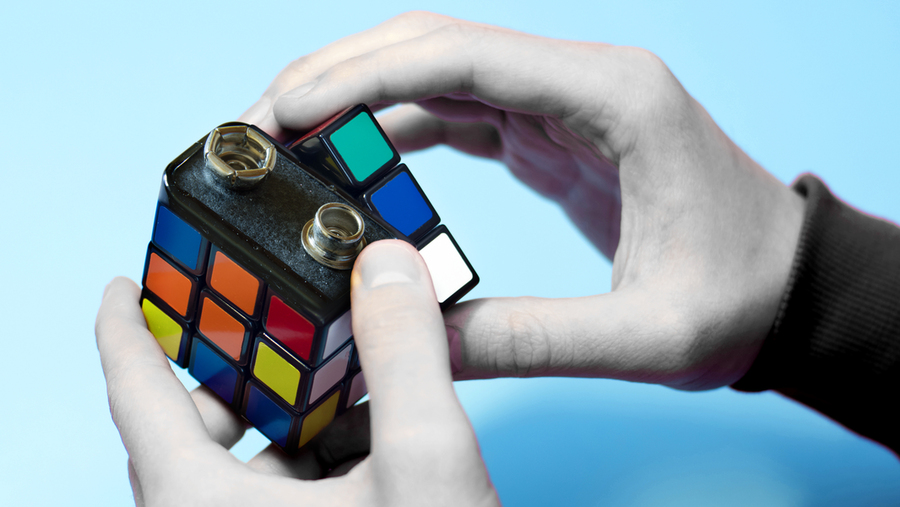
Previous image Next image
“The overall question for me is how to decarbonize society in the most affordable way,” says Nestor Sepulveda SM ’16, PhD ’20. As a postdoc at MIT and a researcher with the MIT Energy Initiative (MITEI), he worked with a team over several years to investigate what mix of energy sources might best accomplish this goal. The group’s initial studies suggested the “need to develop energy storage technologies that can be cost-effectively deployed for much longer durations than lithium-ion batteries,” says Dharik Mallapragada, a research scientist with MITEI.
In a new paper published in Nature Energy, Sepulveda, Mallapragada, and colleagues from MIT and Princeton University offer a comprehensive cost and performance evaluation of the role of long-duration energy storage (LDES) technologies in transforming energy systems. LDES, a term that covers a class of diverse, emerging technologies, can respond to the variable output of renewables, discharging electrons for days and even weeks, providing resilience to an electric grid poised to deploy solar and wind power on a large scale.
“If we want to rely overwhelmingly on wind and solar power for electricity — increasingly the most affordable way to decrease carbon emissions — we have to deal with their intermittency,” says Jesse Jenkins SM ’14, PhD ’18, an assistant professor of mechanical and aerospace engineering and the Andlinger Center for Energy and the Environment at Princeton University and former researcher at MITEI.
In their paper, the researchers analyzed whether LDES paired with renewable energy sources and short-duration energy storage options like lithium-ion batteries could indeed power a massive and cost-effective transition to a decarbonized grid. They also investigated whether LDES might even eliminate the need for available-on-demand, or firm, low-carbon energy sources such as nuclear power and natural gas with carbon capture and sequestration.
“The message here is that innovative and low-cost LDES technologies could potentially have a big impact, making a deeply decarbonized electricity system more affordable and reliable,” says lead author Sepulveda, who now works as a consultant with McKinsey and Company. But, he notes, “We will still be better off retaining firm low-carbon energy sources among our options.”
In addition to Jenkins and Mallapragada, the paper’s coauthors include Aurora Edington SM ’19, a MITEI research assistant at the time of this research and now a consultant at The Cadmus Group; and Richard K. Lester, the Japan Steel Industry Professor and associate provost at MIT, and former head of the Department of Nuclear Science and Engineering.
“As the world begins to focus more seriously on how to achieve deep decarbonization goals in the coming decades, the insights from these system-level studies are essential,” says Lester. “Researchers, innovators, investors, and policymakers will all benefit from knowledge of the cost and technical performance targets that are suggested by this work.”
Performance and cost
The team set out to assess the impacts of LDES solutions in hypothetical electric systems that reflect real-world conditions, where technologies are scrutinized not merely by their standalone attributes, but by their relative value when matched against other energy sources.
“We need to decarbonize at an affordable cost to society, and we wanted to know if LDES can increase our probability of success while also reducing overall system cost, given the other technologies competing in the space,” says Sepulveda.
In pursuit of this goal, the team deployed an electricity system capacity expansion model, GenX, earlier developed by Jenkins and Sepulveda while at MIT. This simulation tool made it possible to evaluate the potential system impact of utilizing LDES technologies, including technologies currently being developed and others that could potentially be developed, for different future low-carbon electric grid scenarios characterized by cost and performance attributes of renewable generation, different types of firm generation, as well as alternative electricity demand projections. The study, says Jenkins, was “the first extensive use of this sort of experimental method of applying wide-scale parametric uncertainty and long-term systems-level analysis to evaluate and identify target goals regarding cost and performance for emerging long-duration energy storage technologies.”
For their study, the researchers surveyed a range of long-duration technologies — some backed by the U.S. Department of Energy’s Advanced Research Projects Agency-Energy (ARPA-E) program — to define the plausible cost and performance attributes of future LDES systems based on five key parameters that encompass a range of mechanical, chemical, electrochemical, and thermal approaches. These include pumped hydropower storage, vanadium redox flow batteries, aqueous sulfur flow batteries, and firebrick resistance-heated thermal storage, among others.
“Think of a bathtub, where the parameter of energy storage capacity is analogous to the volume of the tub,” explains Jenkins. Continuing the analogy, another important parameter, charge power capacity, is the size of the faucet filling the tub, and discharge power capacity, the size of the drain. In the most generalized version of an LDES technology, each attribute of the system can be independently sized. In optimizing an energy system where LDES technology functions as “an economically attractive contributor to a lower-cost, carbon-free grid,” says Jenkins, the researchers found that the parameter that matters the most is energy storage capacity cost.
“For a comprehensive assessment of LDES technology design and its economic value to decarbonized grids, we evaluated nearly 18,000 distinctive cases,” Edington explains, “spanning variations in load and renewable resource availability, northern and southern latitude climates, different combinations of LDES technologies and LDES design parameters, and choice of competing firm low-carbon generation resources.”
Some of the key takeaways from the researchers’ rigorous analysis:
- LDES technologies can offer more than a 10 percent reduction in the costs of deeply decarbonized electricity systems if the storage energy capacity cost (the cost to increase the size of the bathtub) remains under the threshold of $20/kilowatt-hour. This value could increase to 40 percent if energy capacity cost of future technologies is reduced to $1/kWh and to as much as 50 percent for the best combinations of parameters modeled in the space. For purposes of comparison, the current storage energy capacity cost of batteries is around $200/kWh.
- Given today’s prevailing electricity demand patterns, the LDES energy capacity cost must fall below $10/kWh to replace nuclear power; for LDES to replace all firm power options entirely, the cost must fall below $1/kWh.
- In scenarios with extensive electrification of transportation and other end-uses to meet economy-wide deep decarbonization goals, it will be more challenging in northern latitudes to displace firm generation under any likely future combination of costs and efficiency performance range for known LDES technologies. This is primarily due to greater peak electricity demand resulting from heating needs in colder climates.
Actionable insights
While breakthroughs in fusion energy, next-generation nuclear power, or carbon capture could well shake up their models, the researchers believe that insights from their study can make an impact right now.
“People working with LDES can see where their technology fits in to the future electricity mix and ask: 'Does it make economic sense from a system perspective?'” says Mallapragada. “And it’s a call for action in policy and investment in innovation, because we show where the technology gaps lie and where we see the greatest value for research breakthroughs in LDES technology development.”
Not all LDES technologies can clear the bar in this design space, nor can there be reliance on LDES as the exclusive means to expand wind and solar swiftly in the near term, or to enable a complete transition to a zero-carbon economy by 2050.
“We show how promising LDES technologies could be,” says Sepulveda. “But we also show that these technologies are not the one solution, and that we are still better off with them complementing firm resources.”
Jenkins spies niche market opportunities for LDES immediately, such as places with a lot of wind and solar deployed and limits on transmission to export that power. In such locations, storage could fill up when transmission is at its limit, and export power later while maximizing use of the power line capacity. But LDES technologies must be ready to make a major impact by the late 2030s and 2040s, he believes, by which time economies might need to be weaned completely off of natural gas dependency if decarbonization is to succeed.
“We must develop and deploy LDES and improve other low-carbon technologies this decade, so we can present real alternatives to policymakers and power system operators,” he says.
In light of this urgent need, Jenkins at Princeton and Mallapragada at MIT are now working to evaluate and advance technologies with the greatest potential in the storage and energy fields to hasten the zero-carbon goal. With help from ARPA-E and MITEI, they are making the state-of-the-art GenX electricity system planning model an open-source tool for public use as well. If their research and modeling approach can show developers and policymakers what kind of designs are most impactful, says Sepulveda, “We could have a decarbonized system that’s less expensive than today’s system if we do things right.”
This research was supported by a grant from the National Science Foundation, and by MITEI’s Low-Carbon Energy Center for Electric Power Systems.
Share this news article on:
Press mentions.
MIT researchers have analyzed the role of long-duration energy storage technologies and found that large storage systems have the potential to lower electricity prices in a carbon-free grid by up to 40%, writes Eric Roston for Bloomberg.
Previous item Next item
Related Links
- Richard Lester
- Dharik Mallapragada
- Nestor Sepulveda
- Electric Power Systems Center
- MIT Energy Initiative
- Department of Nuclear Science and Engineering
Related Topics
- Nuclear science and engineering
- Renewable energy
- Energy storage
- Sustainability
- Carbon sequestration
Related Articles
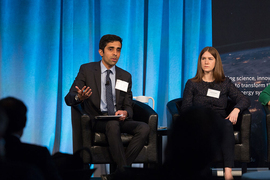
Meet the research scientists behind MITEI’s Electric Power Systems Center
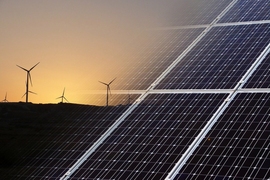
Assessing the value of battery energy storage in future power grids
More mit news.
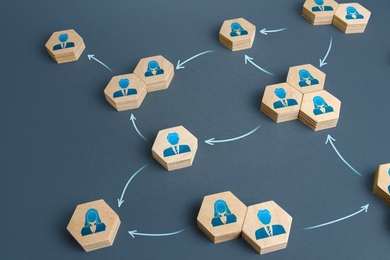
Characterizing social networks
Read full story →
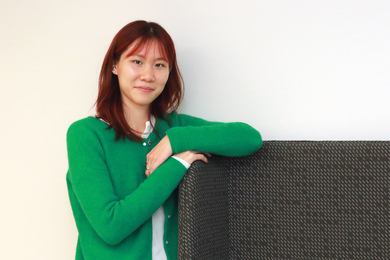
Designing solutions to ensure equity in health care
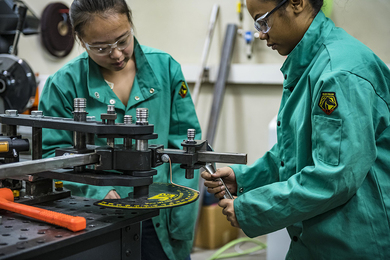

Training manufacturing technologists to be future shop floor leaders
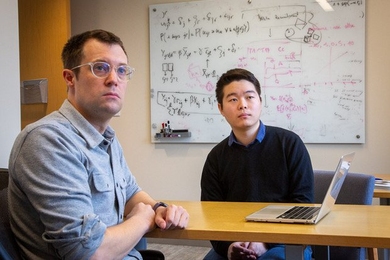
MIT economics to launch new predoctoral fellowship program
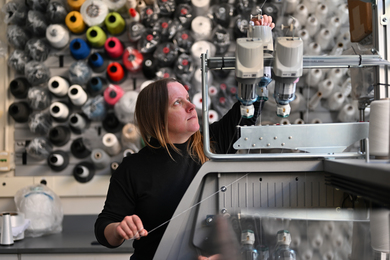
Programming functional fabrics
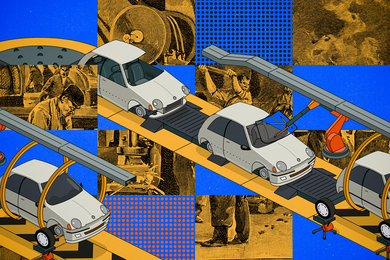
Does technology help or hurt employment?
- More news on MIT News homepage →
Massachusetts Institute of Technology 77 Massachusetts Avenue, Cambridge, MA, USA
- Map (opens in new window)
- Events (opens in new window)
- People (opens in new window)
- Careers (opens in new window)
- Accessibility
- Social Media Hub
- MIT on Facebook
- MIT on YouTube
- MIT on Instagram
- Future Energy Systems Center
- Studies and reports
- Funding opportunities
- Basic energy science
- Built environment and infrastructure
- Climate and environment
- Conventional energy
- Developing world
- Energy efficiency
- Nuclear energy
- Policy and economics
- Power distribution and energy storage
- Renewable energy
- Transportation
- Undergraduate education
- Graduate & postdoctoral
- Online education
- Education research
- Current members
- Energy Futures
- In the media
- Affiliations
Saving heat until you need it
A new concept for thermal energy storage
You can charge a battery, and it’ll store the electricity until you want to use it, say, in your cell phone or electric car. But people have to heat up their solar cooker when the sun’s out, and by the time they want to make dinner, it may well have given off all its stored heat to the cool evening air. Jeffrey Grossman
MIT researchers have demonstrated a new way to store unused heat from car engines, industrial machinery, and even sunshine until it’s needed. Central to their system is a “phase-change” material that absorbs lots of heat as it melts and releases it as it resolidifies. Once melted and activated by ultraviolet light, the material stores the absorbed heat until a beam of visible light triggers solidification and heat release. Key to that control are added molecules that respond to light by changing shape—from one that impedes solidification to a different one that permits it. In a proof-of-concept experiment, the researchers kept a sample mixture in liquid form down to room temperature—fully 10°C below where it should have solidified—and then, after 10 hours, used a light beam to trigger solidification and release the stored thermal energy.
Researchers
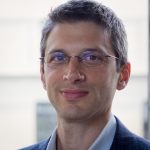
Department of Materials Science and Engineering
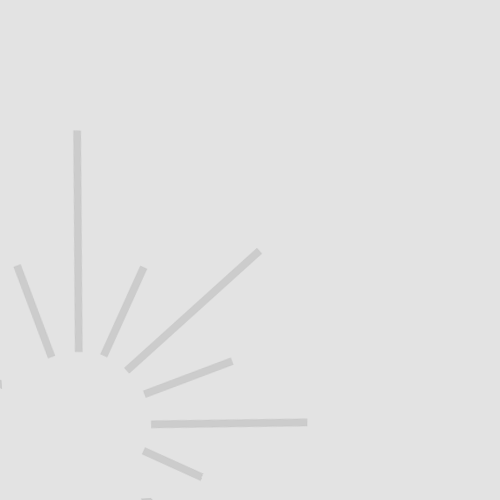
Related news
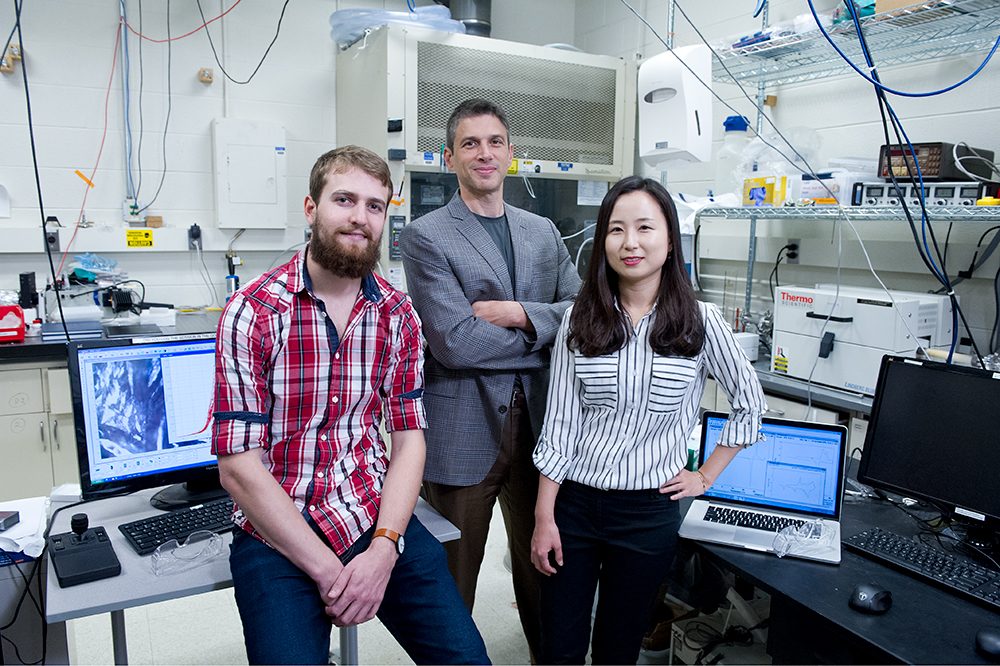

Journal of Materials Chemistry A
Mussel-mimetic thermal conductive films with solid-solid phase change and shape-adaptive performance.
Phase change thermal conductive materials have been applied as heat dissipation interface materials in new electronic devices due to their high thermal conductivity, phase change energy storage performance, low energy consumption, renewability, and long service life. However, it is a huge collage to achieve solid-solid phase change materials with high thermal conductivity, high latent heat of phase change, excellent shape stability, good shape adaptability, and high electrical insulation. Inspired by the oriented "brick-mortar" structure and function of natural mussel, a solid-solid phase change thermal conductive film material had been successfully constructed based on the self-assembly and self-crosslinking behavior of modified polyethylene glycol (CPEG) blending with oriented boron nitride (O-BN). The mussel-like O-BN/CPEG composite film (bio-composite film) was prepared by using boron nitride micro-sheets as "brick" and CPEG as "mortar" through hot pressing and stacking layer by layer. The bio-composite film with a vertical arrangement of boron nitride micron sheets was obtained by longitudinal cutting, which had good shape stability and shape adaptability, high out-of-plane thermal conductivity (12.05 W/(m·K)), low contact thermal resistance (<0.15 cm2·K/W), high latent heat of phase transition (101 J/g) and excellent electrical insulation (volume resistivity was greater than 1011 Ω·cm.) when BN loaded only 20 vol%. Moreover, the film could also be spliced together by heating, which was expected to obtain a large size film with a vertical arrangement structure of BN. Compared with commercial thermal grease, the bio-composite thermally interface management films exhibited higher heat dissipation efficiency in cooling LED chips, and the bio-composite films had excellent heat management capability and stability in the heat dissipation of LED lamps with different powers. This method of constructing mussel-like thermally conductive films with oriented structures offered potential applications for the thermal management of chips, electronic devices, flexible wearable devices, new energy battery systems, and other new electronic devices.
Supplementary files
- Supplementary information PDF (953K)
Article information
Download citation, permissions.

D. Li, C. Ding, S. Shen, J. Wang, L. Wu, B. You and G. Tao, J. Mater. Chem. A , 2024, Accepted Manuscript , DOI: 10.1039/D4TA00031E
To request permission to reproduce material from this article, please go to the Copyright Clearance Center request page .
If you are an author contributing to an RSC publication, you do not need to request permission provided correct acknowledgement is given.
If you are the author of this article, you do not need to request permission to reproduce figures and diagrams provided correct acknowledgement is given. If you want to reproduce the whole article in a third-party publication (excluding your thesis/dissertation for which permission is not required) please go to the Copyright Clearance Center request page .
Read more about how to correctly acknowledge RSC content .
Social activity
Search articles by author.
This article has not yet been cited.
Advertisements
- Share full article
Advertisement
Supported by
Energy Dept. Awards $6 Billion for Green Steel, Cement and Even Macaroni Factories
Industries produce 25 percent of America’s planet-warming emissions but so far have proved very hard to clean up. The Biden administration is trying.

By Brad Plumer
Reporting from Washington
The Biden administration plans to spend up to $6 billion on new technologies to cut carbon dioxide emissions from heavy industries like steel, cement, chemicals and aluminum, which are all enormous contributors to global warming but which have so far been incredibly difficult to clean up.
Energy Secretary Jennifer Granholm said Monday that her agency would partially fund 33 different projects in 20 states to test methods for curbing emissions from a wide variety of factories and industrial plants, calling it “the single largest industrial decarbonization investment in American history.”
Constellium, an aluminum producer, would receive up to $75 million to build a first-of-a-kind aluminum casting plant in Ravenswood, W.Va., that can run on cleaner burning hydrogen fuels rather than natural gas.
Kraft Heinz, a food manufacturer, would get up to $170.9 million to install electric boilers and heat pumps at 10 facilities across the country, where they would be used to generate the large amounts of heat needed for things like drying macaroni without directly burning fossil fuels.
Cleveland-Cliffs, a steel manufacturer, would get up to $500 million to help retire a large coal-consuming blast furnace in Middletown, Ohio, and replace it with two furnaces that use electricity to turn scrap into steel. The company would also test ways to produce steel using hydrogen.
While the projects themselves would put a relatively small dent in U.S. emissions, Ms. Granholm said the goal was to demonstrate novel technologies that can scale up rapidly and “set a new gold standard for clean manufacturing in the United States and around the world.”
Heavy industry is one of the nation’s largest sources of planet-warming pollution, accounting for roughly one-quarter of all emissions. Many factories burn coal or natural gas to produce the heat needed to create steam, temper glass or turn iron into steel. Cement makers emit carbon dioxide as part of the process of transforming limestone into cement. Chemical producers use oil and gas as raw materials for their products.
In theory, there are technologies that can cut emissions. Industrial heat pumps or thermal batteries could help factories generate heat from renewable electricity. Cement makers could capture and bury their carbon dioxide. Steel makers could use clean hydrogen instead of coal. But many of those solutions are expensive and in their infancy.
“It’s different from the electricity sector, where widely available alternatives to fossil fuels like wind, solar and batteries have come down dramatically in cost,” Morgan Bazilian, a professor of public policy at the Colorado School of Mines, said in a recent interview. “With industry, we haven’t yet seen clear winners emerge at the price needed.”
Policymakers have also been hesitant to crack down on industrial emissions for fear that factories and jobs could move abroad to places with looser environmental rules.
While the Biden administration has announced stringent limits for carbon dioxide emissions from vehicles and power plants, it has so far avoided similar regulations for industrial sectors like steel or cement. Instead, the administration has focused on funding new technologies in the hopes that they will get cheaper and become widely adopted.
Separately, various federal agencies have announced plans to buy steel, cement, asphalt and glass made with cleaner processes, in an effort to create a market for low-carbon industrial materials.
The money for the projects in Monday’s announcement comes from the Energy Department’s Industrial Demonstrations Program , which was funded by the 2021 bipartisan infrastructure law and the 2022 Inflation Reduction Act.
The 33 projects selected will need to undergo further negotiations with the agency before receiving final funding.
One is Sublime Systems, a startup exploring cleaner ways to make cement. Traditionally, cement manufacturers burn large quantities of coal or gas to generate temperatures in excess of 2,500 degrees Fahrenheit, which transforms limestone into lime and releases carbon dioxide as part of the chemical conversion.
In contrast, Sublime uses a process powered purely by electricity that doesn’t require high heat or release carbon dioxide. The company has tested its technology at a small pilot facility, and an award from the Energy Department, worth up to $87 million, would help the company build its first commercial plant in Holyoke, Mass.
That funding is valuable, said Leah Ellis, chief executive of Sublime. Many novel technologies to cut industrial emissions “are too expensive for traditional venture capitalists and too risky for conventional project financiers,” she said. Having the Energy Department share the costs of early projects “accelerates the scale-up of these technologies which must be developed and deployed globally as quickly as possible” to fight climate change.
The Energy Department could also fund several projects that use an emerging technology called thermal energy storage , which can take intermittent electricity from wind or solar farms to gradually heat up bricks or other materials, which can then be used to produce steady heat for industrial processes.
“The area that’s often written off as the hardest to decarbonize is the industrial sector,” said Ali Zaidi, President Biden’s national climate adviser. But, he added, “these projects are such a great example of the breadth and diversity of technologies that we can deploy to do this decarbonization work.”
Brad Plumer is a Times reporter who covers technology and policy efforts to address global warming. More about Brad Plumer

IMAGES
VIDEO
COMMENTS
Energy storage can enable dispatchable renewables, but only with drastic cost reductions compared to current batteries. In this thesis, I investigate an electricity storage concept that stores electricity as sensible heat in an extremely hot liquid (>2000°C) and uses multi-junction photovoltaics (MPV) as a heat engine to convert it back to ...
Thesis: Ph. D., Massachusetts Institute of Technology, Department of Mechanical Engineering, 2020 Cataloged from student-submitted PDF of thesis. Includes bibliographical references (pages 149-158).
Thermal energy storage (TES) is a promising class of technologies because energy can be stored cheaply as heat. A TES system converts electricity to heat and converts it back to electricity when needed. TES systems can utilize cheap storage material, but they must address the challenges of low discharge efficiency and to a lesser extent, high ...
storage (LCOS), should be below the $0.06 per kWh current average electricity price15 and 10 or more hours16 of storage are needed to reliably and cost-effectively supply the grid. Thermal energy grid storage In thinking about lower cost storage, one class of technologies that has not received much attention is thermal energy storage (TES).
12 MIT Study on the Future of Energy Storage that is returned upon discharge. The ratio of . energy storage capacity to maximum power . yields a facility's storage . duration, measured . in hours—this is the length of time over which the facility can deliver maximum power when starting from a full charge. Most currently
"The report focuses on a persistent problem facing renewable energy: how to store it. Storing fossil fuels like coal or oil until it's time to use them isn't a problem, but storage systems for solar and wind energy are still being developed that would let them be used long after the sun stops shining or the wind stops blowing," says Asher Klein for NBC10 Boston on MITEI's "Future of Energy ...
These include pumped hydropower storage, vanadium redox flow batteries, aqueous sulfur flow batteries, and firebrick resistance-heated thermal storage, among others. "Think of a bathtub, where the parameter of energy storage capacity is analogous to the volume of the tub," explains Jenkins.
Energy Storage 0420. This survey by MIT's Industrial Liaison Program identifies selected MIT expertise and research in areas. related to energy storage. A key interest for energy storage is in its application to electricity generation, allowing for present energy. production to be retained for use in the future.
Fig 1: Classification of Energy Storage System have been discussed in the report by Edison Electrical Institute. In US almost 93% of energy storage is by pumped storage , followed by thermal storage [12,13].A review of selected energy storage technologies in terms of energy density, efficiency, cost has been presented in the
MIT researchers have demonstrated a new way to store unused heat from car engines, industrial machinery, and even sunshine until it's needed. Central to their system is a "phase-change" material that absorbs lots of heat as it melts and releases it as it resolidifies. Once melted and activated by ultraviolet light, the material stores the ...
Therefore, the current study aims to investigate the influence of renewable generation profiles coupled with alternate storage options (i.e., Li-ion and hydrogen cavern) on the installed capacity of electric-to-thermal-to-electric systems using a 100% renewable electricity system in Germany as a case study.
Abstract. The use of thermal energy storage (TES) allows to cleverly exploit clean energy resources, decrease the energy consumption, and increase the efficiency of energy systems. In the past twenty years, TES has continuously attracted researchers generating an extensive scientific production growing year by year.
Thermal energy storage deals with the storage of energy by cooling, heating, melting, solidifying a material; the thermal energy becomes available when the process is reversed [5]. Thermal energy storage using phase change materials have been a main topic in research since 2000, but although the data is quantitatively enormous.
thermal-energy storage at moderate temperatures based on Phase Change Materials (PCM) Martina Trogrlic. Master's Thesis. Supervisor: Vojislav Novakovic, EPT. ... In this thesis, sensible and latent heat storage are described, as well as phase transformations of materials. Some properties of some phase change materials (PCM) are introduced, ...
The proposed design utilizes a pumped thermal energy storage (PTES) system, which also utilizes waste heat leaving a natural gas peaker plant. This system creates a low cost utility-scale energy storage system by leveraging this dual-functionality. This dissertation first presents a review of previous work in PTES as well as the details of the ...
this thesis, the merits of implementing thermal energy storage systems, or TES-systems, is investigated. This technology may become critical in the shift towards renewables. A huge share of the potential for energy savings of the Dutch industry is related to steam installations (Steam-up, 2020).
Notably, the assembled asymmetric supercapacitor retains 105.5 % of its capacitance after 5000 cycles and has a power density of 803.13 W kg-1 and an energy density of 17.78 Wh kg-1. Fe-doped Co3O4 has a strong future in energy storage, as evidenced by its exceptional electrochemical characteristics.
Phase change thermal conductive materials have been applied as heat dissipation interface materials in new electronic devices due to their high thermal conductivity, phase change energy storage performance, low energy consumption, renewability, and long service life. However, it is a huge collage to achieve
The Energy Department could also fund several projects that use an emerging technology called thermal energy storage, which can take intermittent electricity from wind or solar farms to gradually ...