Brought to you by:


The Fukushima Nuclear Disaster: Causes, Consequences, and Implications
By: Jochen Reb, Yoshisuke Iinuma, Havovi Joshi
This case study discusses the causes, consequences and implications of the nuclear disaster at Tokyo Electric Power Company's (TEPCO's) Fukushima Dai-ichi nuclear power plant that was triggered by a…
- Length: 11 page(s)
- Publication Date: Oct 25, 2012
- Discipline: General Management
- Product #: SMU103-PDF-ENG
What's included:
- Teaching Note
- Educator Copy
$4.95 per student
degree granting course
$8.95 per student
non-degree granting course
Get access to this material, plus much more with a free Educator Account:
- Access to world-famous HBS cases
- Up to 60% off materials for your students
- Resources for teaching online
- Tips and reviews from other Educators
Already registered? Sign in
- Student Registration
- Non-Academic Registration
- Included Materials
This case study discusses the causes, consequences and implications of the nuclear disaster at Tokyo Electric Power Company's (TEPCO's) Fukushima Dai-ichi nuclear power plant that was triggered by a massive 9.0 magnitude earthquake and subsequent tsunami waves on March 11, 2011. There are two essential questions: First, "How could it have come so far?" Japan is rightfully considered a technologically advanced nation and is known for its diligence and high-quality products. While the combined earthquake/tsunami triggered the catastrophe, there are a number of deeper underlying causes that are described in the first section of the case. Second, "What next?" While the plant technically achieved cold shutdown with all damaged reactors reaching temperatures below 100°C, this did not mean that the Fukushima disaster was over. Instead, numerous consequences and implications extend into the future.
Learning Objectives
Students will become more aware of the psychological, interpersonal, organisational, and institutional contributors to crises. They will learn to recognise the development, characteristics, and negative consequences of groupthink; and understand the crucial role of communications during crisis management. Students will also discuss broader implications such as the future of nuclear energy and the responsibility of organisations in catastrophes.
Oct 25, 2012
Discipline:
General Management
Geographies:
Industries:
Energy and natural resources sector
Singapore Management University
SMU103-PDF-ENG
We use cookies to understand how you use our site and to improve your experience, including personalizing content. Learn More . By continuing to use our site, you accept our use of cookies and revised Privacy Policy .
Javascript Required!
Javascript is required for this site.
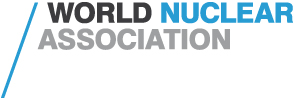
Fukushima Daiichi Accident
(Updated August 2023)
- Following a major earthquake, a 15-metre tsunami disabled the power supply and cooling of three Fukushima Daiichi reactors, causing a nuclear accident beginning on 11 March 2011. All three cores largely melted in the first three days.
- The accident was rated level 7 on the International Nuclear and Radiological Event Scale, due to high radioactive releases over days 4 to 6, eventually a total of some 940 PBq (I-131 eq).
- All four Fukushima Daiichi reactors were written off due to damage in the accident – 2719 MWe net.
- After two weeks, the three reactors (units 1-3) were stable with water addition and by July they were being cooled with recycled water from the new treatment plant. Official 'cold shutdown condition' was announced in mid-December.
- Apart from cooling, the basic ongoing task was to prevent release of radioactive materials, particularly in contaminated water leaked from the three units. This task became newsworthy in August 2013.
- There have been no deaths or cases of radiation sickness from the nuclear accident, but over 100,000 people were evacuated from their homes as a preventative measure. Government nervousness has delayed the return of many.
- Official figures show that there have been 2313 disaster-related deaths among evacuees from Fukushima prefecture. Disaster-related deaths are in addition to the about 19,500 that were killed by the earthquake or tsunami.
The Great East Japan Earthquake of magnitude 9.0 at 2.46 pm on Friday 11 March 2011 did considerable damage in the region, and the large tsunami it created caused very much more. The earthquake was centred 130 km offshore the city of Sendai in Miyagi prefecture on the eastern coast of Honshu Island (the main part of Japan), and was a rare and complex double quake giving a severe duration of about 3 minutes. An area of the seafloor extending 650 km north-south moved typically 10-20 metres horizontally. Japan moved a few metres east and the local coastline subsided half a metre. The tsunami inundated about 560 km 2 and resulted in a human death toll of about 19,500 and much damage to coastal ports and towns, with over a million buildings destroyed or partly collapsed.
Eleven reactors at four nuclear power plants in the region were operating at the time and all shut down automatically when the earthquake hit. Subsequent inspection showed no significant damage to any from the earthquake. The operating units which shut down were Tokyo Electric Power Company's (Tepco's) Fukushima Daiichi 1, 2, 3, and Fukushima Daini 1, 2, 3, 4, Tohoku's Onagawa 1, 2, 3, and Japco's Tokai, total 9377 MWe net. Fukushima Daiichi units 4, 5&6 were not operating at the time, but were affected. The main problem initially centred on Fukushima Daiichi 1-3. Unit 4 became a problem on day five.
The reactors proved robust seismically, but vulnerable to the tsunami. Power, from grid or backup generators, was available to run the residual heat removal (RHR) system cooling pumps at eight of the eleven units, and despite some problems they achieved 'cold shutdown' within about four days. The other three, at Fukushima Daiichi, lost power at 3.42 pm, almost an hour after the earthquake, when the entire site was flooded by the 15-metre tsunami. This disabled 12 of 13 backup generators onsite and also the heat exchangers for dumping reactor waste heat and decay heat to the sea. The three units lost the ability to maintain proper reactor cooling and water circulation functions. Electrical switchgear was also disabled. Thereafter, many weeks of focused work centred on restoring heat removal from the reactors and coping with overheated spent fuel ponds. This was undertaken by hundreds of Tepco employees as well as some contractors, supported by firefighting and military personnel. Some of the Tepco staff had lost homes, and even families, in the tsunami, and were initially living in temporary accommodation under great difficulty and privation, with some personal risk. A hardened onsite emergency response centre was unable to be used in grappling with the situation, due to radioactive contamination.
Three Tepco employees at the Daiichi and Daini plants were killed directly by the earthquake and tsunami, but there have been no fatalities from the nuclear accident.
Among hundreds of aftershocks, an earthquake with magnitude 7.1, closer to Fukushima than the 11 March one, was experienced on 7 April, but without further damage to the plant. On 11 April a magnitude 7.1 earthquake and on 12 April a magnitude 6.3 earthquake, both with the epicentre at Fukushima-Hamadori, caused no further problems.
The two Fukushima plants and their siting
The Daiichi (first) and Daini (second) Fukushima plants are sited about 11 km apart on the coast, Daini to the south.
The recorded seismic data for both plants – some 180 km from the epicentre – shows that 550 Gal (0.56 g) was the maximum ground acceleration for Daiichi, and 254 Gal was maximum for Daini. Daiichi units 2, 3 and 5 exceeded their maximum response acceleration design basis in an east-west direction by about 20%. The recording was over 130-150 seconds. (All nuclear plants in Japan are built on rock – ground acceleration was around 2000 Gal a few kilometres north, on sediments).
The original design basis tsunami height was 3.1 m for Daiichi based on assessment of the 1960 Chile tsunami and so the plant had been built about 10 metres above sea level with the seawater pumps 4 m above sea level. The Daini plant was built 13 metres above sea level. In 2002 the design basis was revised to 5.7 metres above, and the seawater pumps were sealed. In the event, tsunami heights coming ashore were about 15 metres, and the Daiichi turbine halls were under some 5 metres of seawater until levels subsided. Daini was less affected. The maximum amplitude of this tsunami was 23 metres at point of origin, about 180 km from Fukushima.
In the last century there have been eight tsunamis in the region with maximum amplitudes at origin above 10 metres (some much more), these having arisen from earthquakes of magnitude 7.7 to 8.4, on average one every 12 years. Those in 1983 and in 1993 were the most recent affecting Japan, with maximum heights at origin of 14.5 metres and 31 metres respectively, both induced by magnitude 7.7 earthquakes. The June 1896 earthquake of estimated magnitude 8.3 produced a tsunami with run-up height of 38 metres in Tohoku region, killing more than 27,000 people.
The tsunami countermeasures taken when Fukushima Daiichi was designed and sited in the 1960s were considered acceptable in relation to the scientific knowledge then, with low recorded run-up heights for that particular coastline. But some 18 years before the 2011 disaster, new scientific knowledge had emerged about the likelihood of a large earthquake and resulting major tsunami of some 15.7 metres at the Daiichi site. However, this had not yet led to any major action by either the plant operator, Tepco, or government regulators, notably the Nuclear & Industrial Safety Agency (NISA). Discussion was ongoing, but action minimal. The tsunami countermeasures could also have been reviewed in accordance with International Atomic Energy Agency (IAEA) guidelines which required taking into account high tsunami levels, but NISA continued to allow the Fukushima plant to operate without sufficient countermeasures such as moving the backup generators up the hill, sealing the lower part of the buildings, and having some back-up for seawater pumps, despite clear warnings.
A report from the Japanese government's Earthquake Research Committee on earthquakes and tsunamis off the Pacific coastline of northeastern Japan in February 2011 was due for release in April, and might finally have brought about changes. The document includes analysis of a magnitude 8.3 earthquake that is known to have struck the region more than 1140 years ago, triggering enormous tsunamis that flooded vast areas of Miyagi and Fukushima prefectures. The report concludes that the region should be alerted of the risk of a similar disaster striking again. The 11 March earthquake measured magnitude 9.0 and involved substantial shifting of multiple sections of seabed over a source area of 200 x 400 km. Tsunami waves devastated wide areas of Miyagi, Iwate and Fukushima prefectures.
(See also background on Earthquakes and Seismic Protection for Nuclear Power Plants in Japan )
Events at Fukushima Daiichi 1-3 & 4
It appears that no serious damage was done to the reactors by the earthquake, and the operating units 1-3 were automatically shut down in response to it, as designed. At the same time all six external power supply sources were lost due to earthquake damage, so the emergency diesel generators located in the basements of the turbine buildings started up. Initially cooling would have been maintained through the main steam circuit bypassing the turbine and going through the condensers.
Then 41 minutes later, at 3:42 pm, the first tsunami wave hit, followed by a second 8 minutes later. These submerged and damaged the seawater pumps for both the main condenser circuits and the auxiliary cooling circuits, notably the residual heat removal (RHR) cooling system. They also drowned the diesel generators and inundated the electrical switchgear and batteries, all located in the basements of the turbine buildings (the one surviving air-cooled generator was serving units 5&6). So there was a station blackout, and the reactors were isolated from their ultimate heat sink. The tsunamis also damaged and obstructed roads, making outside access difficult.
All this put reactors 1-3 in a dire situation and led the authorities to order, and subsequently extend, an evacuation while engineers worked to restore power and cooling. The 125-volt DC back-up batteries for units 1&2 were flooded and failed, leaving them without instrumentation, control or lighting. Unit 3 had battery power for about 30 hours.
At 7:03 pm Friday 11 March a nuclear emergency was declared, and at 8:50pm the Fukushima prefecture issued an evacuation order for people within 2 km of the plant. At 9:23 pm the prime minister extended this to 3 km, and at 5:44 am on 12 March he extended it to 10 km. He visited the plant soon after. Later on Saturday 12 March he extended the evacuation zone to 20 km.
Inside the Fukushima Daiichi reactors
The Fukushima Daiichi reactors were GE boiling water reactors (BWRs) of an early (1960s) design supplied by GE, Toshiba and Hitachi, with what is known as a Mark I containment. Reactors 1-3 came into commercial operation 1971-75. Reactor capacity was 460 MWe for unit 1, 784 MWe for units 2-5, and 1100 MWe for unit 6.

When the power failed at 3:42 pm, about one hour after shutdown of the fission reactions, the reactor cores would still have been producing about 1.5% of their nominal thermal power, from fission product decay – about 22 MW in unit 1 and 33 MW in units 2&3. Without heat removal by circulation to an outside heat exchanger, this produced a lot of steam in the reactor pressure vessels (RPVs) housing the cores, and this was released into the dry primary containment (PCV) through safety valves. Later this was accompanied by hydrogen, produced by the interaction of the fuel's very hot zirconium cladding with steam after the water level dropped.
As pressure started to rise here, the steam was directed into the suppression chamber/wetwell under the reactor, within the containment, but the internal temperature and pressure nevertheless rose quite rapidly. Water injection commenced, using the various systems provide for this and finally the emergency core cooling system (ECCS). These systems progressively failed over three days, so from early Saturday water injection to the RPV was with fire pumps, but this required the internal pressures to be relieved initially by venting into the suppression chamber/wetwell. Seawater injection into unit 1 began at 7:00 pm on Saturday 12, into unit 3 on Sunday 13 and unit 2 on Monday 14. Tepco management ignored an instruction from the prime minister to cease the seawater injection into unit 1, and this instruction was withdrawn shortly afterwards.
Inside unit 1 , it is understood that the water level dropped to the top of the fuel about three hours after the scram (about 6:00 pm) and the bottom of the fuel 1.5 hours later (7:30 pm). The temperature of the exposed fuel rose to some 2800°C so that the central part started to melt after a few hours and by 16 hours after the scram (7:00 am Saturday) most of it had fallen into the water at the bottom of the RPV. After that, RPV temperatures decreased steadily.
As pressure rose, attempts were made to vent the containment, and when external power and compressed air sources were harnessed this was successful, by about 2:30 pm Saturday, though some manual venting was apparently achieved at about 10:17 am. The venting was designed to be through an external stack, but in the absence of power much of it apparently backflowed to the service floor at the top of the reactor building, representing a serious failure of this system (though another possibility is leakage from the drywell). The vented steam, noble gases and aerosols were accompanied by hydrogen. At 3:36 pm on Saturday 12, there was a hydrogen explosion on the service floor of the building above unit 1 reactor containment, blowing off the roof and cladding on the top part of the building, after the hydrogen mixed with air and ignited. (Oxidation of the zirconium cladding at high temperatures in the presence of steam produces hydrogen exothermically, with this exacerbating the fuel decay heat problem.)
In unit 1 most of the core – as corium, composed of melted fuel and control rods – was assumed to be in the bottom of the RPV, but later it appeared that it had mostly gone through the bottom of the RPV and eroded about 65 cm into the drywell concrete below (which is 2.6 m thick). This reduced the intensity of the heat and enabled the mass to solidify.
Much of the fuel in units 2&3 also apparently melted to some degree, but to a lesser extent than in unit 1, and a day or two later. In mid-May 2011 the unit 1 core would still have been producing 1.8 MW of heat, and units 2&3 about 3.0 MW each.
In mid-2013 the Nuclear Regulation Authority (NRA) confirmed that the earthquake itself had caused no damage to unit 1.
In unit 2 , water injection using the steam-driven back-up water injection system failed on Monday 14, and it was about six hours before a fire pump started injecting seawater into the RPV. Before the fire pump could be used RPV pressure had to be relieved via the wetwell, which required power and nitrogen, hence the delay. Meanwhile the reactor water level dropped rapidly after backup cooling was lost, so that core damage started about 8 pm, and it is now understood that much of the fuel then melted and probably fell into the water at the bottom of the RPV about 100 hours after the scram. Pressure was vented on Sunday 13 and again on Tuesday 15, and meanwhile the blowout panel near the top of the building was opened to avoid a repetition of the hydrogen explosion at unit 1. Early on Tuesday 15, the pressure suppression chamber under the actual reactor seemed to rupture, possibly due to a hydrogen explosion there, and the drywell containment pressure inside dropped. However, subsequent inspection of the suppression chamber did not support the rupture interpretation. Later analysis suggested that a leak of the primary containment developed on Tuesday 15. Most of the radioactive releases from the site appeared to come from unit 2.
In unit 3 , the main backup water injection system failed at about 11:00 am on Saturday 12, and early on Sunday 13 water injection using the high pressure system failed also and water levels dropped dramatically. RPV pressure was reduced by venting steam into the wetwell, allowing injection of seawater using a fire pump from just before noon. Early on Sunday venting the suppression chamber and containment was successfully undertaken. It is now understood that core damage started about 5:30 am and much or all of the fuel melted on the morning of Sunday 13 and fell into the bottom of the RPV, with some probably going through the bottom of the reactor pressure vessel and onto the concrete below.
Early on Monday 14 PCV venting was repeated, and this evidently backflowed to the service floor of the building, so that at 11:00 am a very large hydrogen explosion here above unit 3 reactor containment blew off much of the roof and walls and demolished the top part of the building. This explosion created a lot of debris, and some of that on the ground near unit 3 was very radioactive.
In defuelled unit 4 , at about 6:00 am on Tuesday 15 March, there was an explosion which destroyed the top of the building and damaged unit 3's superstructure further. This was apparently from hydrogen arising in unit 3 and reaching unit 4 by backflow in shared ducts when vented from unit 3.
Units 1-3: Water had been injected into each of the three reactor units more or less continuously, and in the absence of normal heat removal via external heat exchanger this water was boiling off for some months. In the government report to the IAEA in June it was estimated that to the end of May about 40% of the injected water boiled off, and 60% leaked out the bottom. In June 2011 this was adding to the contaminated water onsite by about 500 m 3 per day. In January 2013 4.5 to 5.5 m 3 /h was being added to each RPV via core spray and feedwater systems, hence 370 m 3 per day, and temperatures at the bottom of RPVs were 19 °C in unit 1 and 32 °C in units 2&3, at little above atmospheric pressure.
There was a peak of radioactive release on Tuesday 15, apparently mostly from unit 2, but the precise source remains uncertain. Due to volatile and easily-airborne fission products being carried with the hydrogen and steam, the venting and hydrogen explosions discharged a lot of radioactive material into the atmosphere, notably iodine and caesium. NISA said in June that it estimated that 800-1000 kg of hydrogen had been produced in each of the units.
Nitrogen was being injected into the PCVs of all three reactors to remove concerns about further hydrogen explosions, and in December this was started also for the pressure vessels. Gas control systems which extract and clean the gas from the PCV to avoid leakage of caesium were commissioned for all three units.
Throughout 2011 injection into the RPVs of water circulated through the new water treatment plant achieved relatively effective cooling, and temperatures at the bottom of the RPVs were stable in the range 60-76 °C at the end of October, and 27-54 °C in mid-January 2012. RPV pressures ranged from atmospheric to slightly above (102-109 kPa) in January, due to water and nitrogen injection. However, since they were leaking, the normal definition of 'cold shutdown' did not apply, and Tepco waited to bring radioactive releases under control before declaring 'cold shutdown condition' in mid-December, with NISA's approval. This, with the prime minister's announcement of it, formally brought to a close the 'accident' phase of events.
The AC electricity supply from external source was connected to all units by 22 March. Power was restored to instrumentation in all units except unit 3 by 25 March. However, radiation levels inside the plant were so high that normal access was impossible until June.
Event sequence following earthquake (timing from it: 14:46, 11 March)
* according to 2012 MAAP (Modular Accident Analysis Program) analysis
By March 2016 total decay heat in units 1-3 had dropped to 1 MW for all three, about 1% of the original level, meaning that cooling water injection – then 100 m 3 /d – could be interrupted for up to two days.
Results of muon measurements in unit 2 in 2016 indicate that most of the fuel debris in unit 2 is in the bottom of the reactor vessel.
Tepco has written off the four reactors damaged by the accident, and is decommissioning them.
Summary : Major fuel melting occurred early on in all three units, though the fuel remained essentially contained except for some volatile fission products vented early on, or released from unit 2 in mid-March, and some soluble ones which were leaking with the water, especially from unit 2, where the containment is evidently breached. Cooling is provided from external sources, using treated recycled water, with a stable heat removal path from the actual reactors to external heat sinks. Access has been gained to all three reactor buildings, but dose rates remain high inside. Tepco declared 'cold shutdown condition' in mid-December 2011 when radioactive releases had reduced to minimal levels.
(See also background on nuclear reactors at Fukushima Daiichi .)
Fuel ponds: developing problems
Used fuel needs to be cooled and shielded. This is initially by water, in ponds. After about three years underwater, used fuel can be transferred to dry storage, with air ventilation simply by convection. Used fuel generates heat, so the water in ponds is circulated by electric pumps through external heat exchangers, so that the heat is dumped and a low temperature maintained. There are fuel ponds near the top of all six reactor buildings at the Daiichi plant, adjacent to the top of each reactor so that the fuel can be unloaded underwater when the top is off the reactor pressure vessel and it is flooded. The ponds hold some fresh fuel and some used fuel, the latter pending its transfer to the onsite central used/spent fuel storage. (There is some dry storage onsite to extend the plant's capacity.)
At the time of the accident, in addition to a large number of used fuel assemblies, unit 4's pond also held a full core load of 548 fuel assemblies while the reactor was undergoing maintenance, these having been removed at the end of November, and were to be replaced in the core.
A separate set of problems arose as the fuel ponds, holding fresh and used fuel in the upper part of the reactor structures, were found to be depleted in water. The primary cause of the low water levels was loss of cooling circulation to external heat exchangers, leading to elevated temperatures and probably boiling, especially in the heavily-loaded unit 4 fuel pond. Here the fuel would have been uncovered in about 7 days due to water boiling off. However, the fact that unit 4 was unloaded meant that there was a large inventory of water at the top of the structure, and enough of this replenished the fuel pond to prevent the fuel becoming uncovered – the minimum level reached was about 1.2 m above the fuel on about 22 April.
After the hydrogen explosion in unit 4 early on Tuesday 15 March, Tepco was told to implement injection of water to unit 4 pond which had a particularly high heat load (3 MW) from 1331 used fuel assemblies in it, so it was the main focus of concern. It needed the addition of about 100 m 3 /day to replenish it after circulation ceased.
From Tuesday 15 March attention was given to replenishing the water in the ponds of units 1, 2&3 as well. Initially this was attempted with fire pumps but from 22 March a concrete pump with 58-metre boom enabled more precise targeting of water through the damaged walls of the service floors. There was some use of built-in plumbing for unit 2. Analysis of radionuclides in water from the used fuel ponds suggested that some of the fuel assemblies might have been damaged, but the majority were intact.
There was concern about the structural strength of unit 4 building, so support for the pond was reinforced by the end of July.
New cooling circuits with heat exchangers adjacent to the reactor buildings for all four ponds were commissioned after a few months, and each reduced the pool temperature from 70 °C to normal in a few days. Each has a primary circuit within the reactor and waste treatment buildings and a secondary circuit dumping heat through a small dry cooling tower outside the building.
The next task was to remove the salt from those ponds which had seawater added, to reduce the potential for corrosion.
In July 2012 two of the 204 fresh fuel assemblies were removed from the unit 4 pool and transferred to the central spent fuel pool for detailed inspection to check damage, particularly corrosion. They were found to have no deformation or corrosion. Unloading the 1331 spent fuel assemblies in pond 4 and transferring them to the central spent fuel pool commenced in mid-November 2013 and was completed 13 months later. These comprised 783 spent fuel plus the full fuel load of 548.
The next focus of attention was the unit 3 pool. In 2015 the damaged fuel handling equipment and other wreckage was removed from the destroyed upper level of the reactor building. Toshiba built a 74-tonne fuel handling machine for transferring the 566 fuel assemblies into casks and to remove debris in the pool, and a crane for lifting the fuel transfer casks. Installation of a cover over the fuel handling machine was completed in February 2018. Removal and transferral of the fuel to the central spent fuel pool began in mid-April 2019 and was completed at the end of February 2021.
The onsite central spent fuel pool in 2011 held about 60% of the Daiichi used fuel, and is immediately west (inland) of unit 4. It lost circulation with the power outage, and temperature increased to 73 °C by the time mains power and cooling were restored after two weeks. In late 2013 this pond, with capacity for 6840*, held 6375 fuel assemblies, the same as at the time of the accident. In June 2018, Tepco announced it would transfer some of the fuel assemblies stored in the central spent fuel pool to an onsite temporary dry storage facility to clear sufficient space for the fuel assemblies from unit 3's pool. The dry storage facility has a capacity of at least 2930 assemblies in 65 casks – each dry cask holds 50 fuel assemblies. Eventually these will be shipped to JNFL’s Rokkasho reprocessing plant or to Recyclable Fuel Storage Company’s Mutsu facility.
* effectively 6750, due to one rack of 90 having some damaged fuel.
Summary: The spent fuel storage pools survived the earthquake, tsunami and hydrogen explosions without significant damage to the fuel, significant radiological release, or threat to public safety. The new cooling circuits with external heat exchangers for the four ponds are working well and temperatures are normal. Analysis of water has confirmed that most fuel rods are intact. All fuel assemblies have been removed from the unit 3&4 pools.
(See also background on Fukushima Fuel Ponds and Decommissioning section below.)
Radioactive releases to air
Regarding releases to air and also water leakage from Fukushima Daiichi, the main radionuclide from among the many kinds of fission products in the fuel was volatile iodine-131, which has a half-life of 8 days. The other main radionuclide is caesium-137, which has a 30-year half-life, is easily carried in a plume, and when it lands it may contaminate land for some time. It is a strong gamma-emitter in its decay. Cs-134 is also produced and dispersed; it has a two-year half-life. Caesium is soluble and can be taken into the body, but does not concentrate in any particular organs, and has a biological half-life of about 70 days. In assessing the significance of atmospheric releases, the Cs-137 figure is multiplied by 40 and added to the I-131 number to give an 'iodine-131 equivalent' figure.
As cooling failed on the first day, evacuations were progressively ordered, due to uncertainty about what was happening inside the reactors and the possible effects. By the evening of Saturday 12 March the evacuation zone had been extended to 20 km from the plant. From 20 to 30 km from the plant, the criterion of 20 mSv/yr dose rate was applied to determine evacuation, and is now the criterion for return being allowed. 20 mSv/yr was also the general limit set for children's dose rate related to outdoor activities, but there were calls to reduce this. In areas with 20-50 mSv/yr from April 2012 residency is restricted, with remediation action taken. See later section on Public health and return of evacuees .
A significant problem in tracking radioactive release was that 23 out of the 24 radiation monitoring stations on the plant site were disabled by the tsunami.
There is some uncertainty about the amount and exact sources of radioactive releases to air (see also background on Radiation Exposure ).
Japan’s regulator, the Nuclear & Industrial Safety Agency (NISA), estimated in June 2011 that 770 PBq (iodine-131 equivalent) of radioactivity had been released, but the Nuclear Safety Commission (NSC, a policy body) in August lowered this estimate to 570 PBq . The 770 PBq figure is about 15% of the Chernobyl release of 5200 PBq iodine-131 equivalent. Most of the release was by the end of March 2011.
Tepco sprayed a dust-suppressing polymer resin around the plant to ensure that fallout from mid-March was not mobilized by wind or rain. In addition it removed a lot of rubble with remote control front-end loaders, and this further reduced ambient radiation levels, halving them near unit 1. The highest radiation levels onsite came from debris left on the ground after the explosions at units 3&4.
Reactor covers
In mid-May 2011 work started towards constructing a cover over unit 1 to reduce airborne radioactive releases from the site, to keep out the rain, and to enable measurement of radioactive releases within the structure through its ventilation system. The frame was assembled over the reactor, enclosing an area 42 x 47 m, and 54 m high. The sections of the steel frame fitted together remotely without the use of screws and bolts. All the wall panels had a flameproof coating, and the structure had a filtered ventilation system capable of handling 40,000 cubic metres of air per hour through six lines, including two backup lines. The cover structure was fitted with internal monitoring cameras, radiation and hydrogen detectors, thermometers and a pipe for water injection. The cover was completed with ventilation systems working by the end of October 2011. It was expected to be needed for two years. In May 2013 Tepco announced its more permanent replacement, to be built over four years. It started demolishing the 2011 cover in 2014 and finished in 2016. In December 2019 it decided to install the replacement cover before removing debris from the top floor of the building. A crane and other equipment for fuel removal will be installed under the cover, similar to that over unit 4.
More substantial covers were designed to fit around units 3&4 reactor buildings after the top floors were cleared up in 2012.
A cantilevered structure was built over unit 4 from April 2012 to July 2013 to enable recovery of the contents of the spent fuel pond. This is a 69 x 31 m cover (53 m high) and it was fully equipped by the end of 2013 to enable unloading of used fuel from the storage pond into casks, each holding 22 fuel assemblies, and removal of the casks. This operation was accomplished under water, using the new fuel handling machine (replacing the one destroyed by the hydrogen explosion) so that the used fuel could be transferred to the central storage onsite. Transfer was completed in December 2014. A video of the process is available on Tepco's website.
A different design of cover was built over unit 3, and foundation work began in 2012. Large rubble removal took place from 2013 to 2015, including the damaged fuel handling machine. An arched cover was prefabricated, 57 m long and 19 m wide, and supported by the turbine building on one side and the ground on the other. A crane removed the 566 fuel assemblies from the pool and some remaining rubble. Spent fuel removal from unit 3 pool began in April 2019 and was completed in February 2021. Spent fuel removal from units 1&2 pools was scheduled in 2018, but is now scheduled to begin in 2023.

Maps from the Ministry of Education, Culture, Sports, Science and Tehcnology (MEXT) aerial surveys carried out approximately one year apart show the reduction in contamination from late 2011 to late 2012. Areas with colour changes in 2012 showed approximately half the contamination as surveyed in 2011, the difference coming from decay of caesium-134 (two-year half-life) and natural processes like wind and rain. In blue areas, ambient radiation is very similar to global background levels at <0.5 microsieverts per hour, which is equal to <4.4 mSv/yr.
Tests on radioactivity in rice have been made and caesium was found in a few of them. The highest levels were about one-quarter of the allowable limit of 500 Bq/kg, so shipments to market are permitted.
Summary : Major releases of radionuclides, including long-lived caesium, occurred to air, mainly in mid-March. The population within a 20km radius had been evacuated three days earlier. Considerable work was done to reduce the amount of radioactive debris onsite and to stabilize dust. The main source of radioactive releases was the apparent hydrogen explosion in the suppression chamber of unit 2 on 15 March. A cover building for unit 1 reactor was built and the unit is now being dismantled, a more substantial one for unit 4 was built to enable fuel removal during 2014. Radioactive releases in mid-August 2011 had reduced to 5 GBq/hr, and dose rate from these at the plant boundary was 1.7 mSv/yr, less than natural background.
Sequence of evacuation orders based on the report by The National Diet of Japan Fukushima Nuclear Accident Independent Investigation Commission : 11 March 14:46 JST The earthquake occurred. 15:42 TEPCO made the first emergency report to the government. 19:03 The government announced nuclear emergency. 20:50 The Fukushima Prefecture Office ordered 2km radius evacuation. 21:23 The government ordered 3km evacuation and to keep staying inside buildings in the area of 3-10km radius. 12 March 05:44 The government ordered 10km radius evacuation. 18:25 The government ordered 20km evacuation. 15 March 11:01 The government ordered to keep staying inside buildings in the area of 20-30km from the plant. 25 March The government requested voluntary evacuation in the area of 20-30km. 21 April The government set the 20km radius no-go area.
Radiation exposure on the plant site
By the end of 2011, Tepco had checked the radiation exposure of 19,594 people who had worked on the site since 11 March. For many of these both external dose and internal doses (measured with whole-body counters) were considered. It reported that 167 workers had received doses over 100 mSv. Of these 135 had received 100 to 150 mSv, twenty-three 150-200 mSv, three more 200-250 mSv, and six had received over 250 mSv (309 to 678 mSv) apparently due to inhaling iodine-131 fumes early on. The latter included the units 3&4 control room operators in the first two days who had not been wearing breathing apparatus. There were up to 200 workers onsite each day. Recovery workers wear personal monitors, with breathing apparatus and protective clothing which protect against alpha and beta radiation. The level of 250 mSv was the allowable maximum short-term dose for Fukushima Daiichi accident clean-up workers through to December 2011, 500 mSv is the international allowable short-term dose "for emergency workers taking life-saving actions". In January 2012 the allowable maximum reverted to 50 mSv/yr.
No radiation casualties (acute radiation syndrome) occurred, and few other injuries, though higher than normal doses, were being accumulated by several hundred workers onsite. High radiation levels in the three reactor buildings hindered access there.
Monitoring of seawater, soil and atmosphere is at 25 locations on the plant site, 12 locations on the boundary, and others further afield. Government and IAEA monitoring of air and seawater is ongoing. Some high but not health-threatening levels of iodine-131 were found in March, but with an eight-day half-life, most I-131 had gone by the end of April 2011.
A radiation survey map of the site made in March 2013 revealed substantial progress: the highest dose rate anywhere on the site was 0.15 mSv/h near units 3 and 4. (Soon after the accident a similar survey put the highest dose rate at 300 mSv/h near rubble lying alongside unit 3.) The majority of the power plant area was at less than 0.01 mSv/h. These reduced levels are reflected in worker doses: during January 2013, the 5702 workers at the site received an average of 0.86 mSv, with 75% of workers recorded as receiving less than 1 mSv. In total, only about 2% of workers received over 5 mSv and the highest dose in January was 12.65 mSv for one worker.
Media reports have referred to 'nuclear gypsies' – casual workers employed by subcontractors on a short-term basis, and allegedly prone to receiving higher and unsupervised radiation doses. This transient workforce has been part of the nuclear scene for at least four decades, and at Fukushima their doses are very rigorously monitored. If they reach certain levels, e.g. 30 mSv but varying according to circumstance, they are reassigned to lower-exposure areas.
Tepco figures submitted to the NRA for the period to end January 2014 showed 173 workers had received more than 100 mSv (six more than two years earlier) and 1578 had received 50 to 100 mSv. This was among a total of 32,024, 64% more than had worked there two years earlier. Since April 2013 none of the 13,154 who had worked onsite had received more than 50 mSv, and 96% of these had less than a 20 mSv dose. Early in 2014 there were about 4000 onsite each weekday.
Summary : Six workers received radiation doses apparently over the 250 mSv level set by NISA, but at levels below those which would cause radiation sickness.
Radiation exposure and fallout beyond the plant site
On 4 April 2011, radiation levels of 0.06 mSv/day were recorded in Fukushima city, 65 km northwest of the plant, about 60 times higher than normal but posing no health risk according to authorities. Monitoring beyond the 20 km evacuation radius to 13 April showed one location – around Iitate – with up to 0.266 mSv/day dose rate, but elsewhere no more than one-tenth of this. At the end of July the highest level measured within 30km radius was 0.84 mSv/day in Namie town, 24 km away. The safety limit set by the central government in mid-April for public recreation areas was 3.8 microsieverts per hour (0.09 mSv/day).
In June 2013, analysis from Japan's Nuclear Regulation Authority (NRA) showed that the most contaminated areas in the Fukushima evacuation zone had reduced in size by three-quarters over the previous two years. The area subject to high dose rates (over 166 mSv/yr) diminished from 27% of the 1117 km 2 zone to 6% over 15 months to March 2013, and in the ‘no residence’ portion (originally 83-166 mSv/yr) no areas remained at this level and 70% was below 33 mSv/yr. The least-contaminated area is now entirely below 33 mSv/yr.
In August 2011 The Act on Special Measures Concerning the Handling of Radioactive Pollution was enacted and it took full effect from January 2012 as the main legal instrument to deal with all remediation activities in the affected areas, as well as the management of materials removed as a result of those activities. It specified two categories of land: Special Decontamination Areas consisting of the 'restricted areas' located within a 20 km radius from the Fukushima Daiichi plant, and 'deliberate evacuation areas' where the annual cumulative dose for individuals was anticipated to exceed 20 mSv. The national government promotes decontamination in these areas. These areas are subdivided into three: dose 1-20 mSv/yr (green); dose 20-50 mSv/yr (yellow); and dose over 50 mSv/yr and over 20 mSv/yr averaged over 5 years (red). Intensive Contamination Survey Areas including the so-called Decontamination Implementation Areas, where an additional annual cumulative dose between 1 mSv and 20 mSv was estimated for individuals. Municipalities implement decontamination activities in these areas.
Following a detailed study by 80 international experts, the UN Scientific Committee on the Effects of Atomic Radiation's (UNSCEAR's) May 2013 Report to the General Assembly concluded: "No radiation-related deaths or acute diseases have been observed among the workers and general public exposed to radiation from the accident. The doses to the general public, both those incurred during the first year and estimated for their lifetimes, are generally low or very low. No discernible increased incidence of radiation-related health effects are expected among exposed members of the public or their descendants."
However, the report noted: "More than 160 additional workers received effective doses currently estimated to be over 100 mSv, predominantly from external exposures. Among this group, an increased risk of cancer would be expected in the future. However, any increased incidence of cancer in this group is expected to be indiscernible because of the difficulty of confirming such a small incidence against the normal statistical fluctuations in cancer incidence."
These workers are individually monitored annually for potential late radiation-related health effects. UNSCEAR’s follow-up white paper in October 2015 said that none of the new information appraised after the 2013 report “materially affected the main findings or challenged the major assumptions of the 2013 Fukushima report."
By contrast, the public was exposed to 10-50 times less radiation. Most Japanese people were exposed to additional radiation amounting to less than the typical natural background level of 2.1 mSv per year.
In 2018 UNSCEAR decided to update the 2013 report to reflect the latest findings. In March 2021, UNSCEAR published its 2020 Report , which broadly confirms the major findings and conclusions of the 2013 report. The 2020 Report states: "No adverse health effects among Fukushima residents have been documented that are directly attributable to radiation exposure from the Fukushima Daiichi nuclear plant accident. The Committee’s revised estimates of dose are such that future radiation-associated health effects are unlikely to be discernible."
People living in Fukushima prefecture are expected to be exposed to around 10 mSv over their entire lifetimes, while for those living further away the dose would be 0.2 mSv per year. The UNSCEAR conclusion reinforces the findings of several international reports to date, including one from the World Health Organization (WHO) that considered the health risk to the most exposed people possible: a postulated girl under one year of age living in Iitate or Namie that did not evacuate and continued life as normal for four months after the accident. Such a child's theoretical risk of developing any cancer would be increased only marginally, according to the WHO's analysis.
Despite the conclusions of reports from UNSCEAR and the WHO, the Japanese government in 2018 acknowledged a connection between the death of a former plant worker and radiation exposure. The man had been diagnosed with lung cancer in February 2016.
Eleven municipalities in the former restricted zone or planned evacuation area, within 20 km of the plant or where annual cumulative radiation dose is greater than 20 mSv, are designated 'special decontamination areas', where decontamination work is being implemented by the government. A further 100 municipalities in eight prefectures, where dose rates are equivalent to over 1 mSv per year are classed as 'intensive decontamination survey areas', where decontamination is being implemented by each municipality with funding and technical support from the national government. Decontamination of all 11 special decontamination areas has been completed.
In October 2013 a 16-member IAEA mission reported on remediation and decontamination in the special decontamination areas. Its preliminary report said that decontamination efforts were commendable but driven by unrealistic targets. If annual radiation dose was below 20 mSv, such as generally in intensive decontamination survey areas, this level was “acceptable and in line with the international standards and with the recommendations from the relevant international organizations, e.g. ICRP, IAEA, UNSCEAR and WHO.” The clear implication was that people in such areas should be allowed to return home. Furthermore the government should increase efforts to communicate this to the public, and should explain that its long-term goal of achieving an additional individual dose of 1 mSv/yr is unrealistic and unnecessary in the short term. Also, there is potential to produce more food safely in contaminated areas.
Radioactivity, primarily from caesium-137, in the evacuation zone and other areas beyond it has been reported in terms of kBq/kg (compared with kBq/m 2 around Chernobyl. A total of 3000 km 2 was contaminated above 180 kBq/m 2 , compared with 29,400 km 2 from Chernobyl). However the main measure has been presumed doses in mSv/yr. The government has adopted 20 mSv/yr as its goal for the evacuation zone and more contaminated areas outside it, and supports municipal government work to reduce levels below that. The total area under consideration for attention is 13,000 km 2 . In 2016 the Ministry of Environment announced that material with less than 8 kBq/kg caesium would no longer be specified as waste, and subject to restrictions on disposal. It allowed use of contaminated soil for embankments, where the activity was less then 8 kBq/kg, and unrestricted use if less than 100 Bq/kg. Most of the stored wastes have decayed to below the 8 kBq/kg level.
Summary : There have been no harmful effects from radiation on local people, nor any doses approaching harmful levels. However, some 160,000 people were evacuated from their homes and only from 2012 were allowed limited return. As of July 2020 over 41,000 remained displaced due to government concern about radiological effects from the accident.
Public health and return of evacuees
Permanent return remains a high priority, and the evacuation zone is being decontaminated where required and possible, so that evacuees can return. There are many cases of evacuation stress including transfer trauma among evacuees, and once the situation had stabilized at the plant these outweighed the radiological hazards of returning, with 2313 deaths reported (see below ).
In December 2011 the government said that where annual radiation dose would be below 20 mSv/yr, the government would help residents return home as soon as possible and assist local municipalities with decontamination and repair of infrastructure. In areas where radiation levels are over 20 mSv/yr evacuees will be asked to continue living elsewhere for “a few years” until the government completes decontamination and recovery work. The government said it would consider purchasing land and houses from residents of these areas if the evacuees wish to sell them.
In November 2013 the NRA decided to change the way radiation exposure was estimated. Instead of airborne surveys being the basis, personal dosimeters would be used, giving very much more accurate figures, often much less than airborne estimates. The same criteria would be used, as above, with 20 mSv/yr being the threshold of concern to authorities.
In February 2014 the results of a study were published showing that 458 residents of two study areas 20 to 30 km from the plant and a third one 50 km northwest received radiation doses from the contaminated ground similar to the country’s natural background levels. Measurement was by personal dosimeters over August-September 2012.

By September 2020, 2313 disaster-related deaths among evacuees from Fukushima prefecture*, that were not due to radiation-induced damage or to the earthquake or to the tsunami, had been identified by the Japanese authorities. About 90% of deaths were for persons above 66 years of age. Of these, about 30% occurred within the first three months of the evacuations, and about 80% within two years.
* An additional 1454 disaster-related deaths have been reported among evacuees from other tsunami- and earthquake-related prefectures. Disaster-related deaths are in addition to the over 19,500 that died in the actual earthquake and tsunami.
The premature disaster-related deaths were mainly related to (i) physical and mental illness brought about by having to reside in shelters and the trauma of being forced to move from care settings and homes; and (ii) delays in obtaining needed medical support because of the enormous destruction caused by the earthquake and tsunami.
However, the radiation levels in most of the evacuated areas were not greater than the natural radiation levels in high background areas elsewhere in the world where no adverse health effect is evident.
In its December 2018 report , the Fukushima prefectural government said that the number of ‘indirect’ deaths in the prefecture was greater than the number (1829) killed in the earthquake and tsunami. It put the figure then at 2259 (since revised up to 2313) as determined by municipal panels that examine links between the disaster’s aftermath and deaths. The figure is greater than for Iwate and Miyagi prefectures, with 469 and 929 respectively, though they had much higher loss of life in the earthquake and tsunami – over 14,000. The disparity is attributed to the older age group involved among Fukushima’s evacuated earthquake/tsunami survivors, about 90% of indirect deaths being of people over 66. Causes of indirect deaths include physical and mental stress stemming from long stays at shelters, a lack of initial care as a result of hospitals being disabled by the disaster, and suicides. Evaluation of ‘indirect deaths’ is according to a model developed by Niigata prefecture after the 2004 earthquake there ( Japan Times 20/2/14 ). As of July 2020, over 41,000 people from Fukushima were still living as evacuees.
Evacuees over a period of six years received ¥100,000 (about $1000) per month in psychological suffering compensation. The money was tax-exempt and paid unconditionally. In October 2013, about 84,000 evacuees received the payments. Statistics indicate that an average family of four has received about ¥90 million ($900,000) in compensation from Tepco. As of January 2021 the Fukushima accident evacuees had received ¥9.7 trillion in personal and property compensation.
The Fukushima prefecture had 17,000 government-financed temporary housing units for some 29,500 evacuees from the accident. The prefectural government said residents could continue to use these until March 2015 ( Japan Times 17/11/13 ). The number compared with very few built in Miyagi, Iwate and Aomori prefectures for the 222,700 tsunami survivor refugees there.
In April 2019, the first residents of Okuma, the closest town to the plant, were allowed to return home. In August 2022 the last remaining evacuation order – for Futaba, the town that hosts the Fukushima plant – was lifted.
According to a survey released by the prefectural government in April 2017, the majority of people who voluntarily evacuated their homes after the accident and who are now living outside of Fukushima prefecture do not intend to return. A Mainichi report said that 78.2% of respondents to the survey preferred to continue living in the area to which they had moved, while only 18.3% intended to move back to the prefecture. Of the voluntary evacuees still living in Fukushima prefecture, 23.6% planned to stay where they were, while 66.6% hoped to return to their original homes.
An August 2012 Reconstruction Agency report also considered workers at Fukushima power plant. Of almost 1500 surveyed, many were stressed, due to evacuating their homes (70%), believing they had come close to death (53%), the loss of homes in the tsunami (32%), deaths of colleagues (20%) and of family members (6%) mostly in the tsunami. The death toll directly due to the nuclear accident or radiation exposure remained zero, but stress and disruption due to the continuing evacuation remains high.
Tokyo’s Board of Audit reported in October 2013 that 23% of recovery funding – about ¥1.45 trillion ($14.5 billion) – had been misappropriated. Some 326 out of about 1400 projects funded had no direct relevance to the natural disaster or Fukushima Daiichi accident (Mainichi 1/11/13).
Summary : Many evacuated people remain unable to fully return home due to government-mandated restrictions based on conservative radiation exposure criteria. 2313 premature disaster-related deaths were caused by the evacuations, with 90% of the deaths occuring in people aged 66 and older. Decontamination work is proceeding while radiation levels decline naturally. The October 2013 IAEA report made clear that many evacuees should be allowed to return home. As of July 2020, over 41,000 people from Fukushima were still living as evacuees.
Managing contaminated water
Removing contaminated water from the reactor and turbine buildings had become the main challenge by week 3, along with contaminated water in trenches carrying cabling and pipework. This was both from the tsunami inundation and leakage from reactors. Run-off from the site into the sea was also carrying radionuclides well in excess of allowable levels. By the end of March all storages around the four units – basically the main condenser units and condensate tanks – were largely full of contaminated water pumped from the buildings.
Accordingly, with government approval, Tepco over 4-10 April released to the sea about 10,400 cubic metres of slightly contaminated water (0.15 TBq total) in order to free up storage for more highly-contaminated water from unit 2 reactor and turbine buildings which needed to be removed to make safe working conditions. Unit 2 was the main source of contaminated water, though some of it comes from drainage pits. NISA confirmed that there was no significant change in radioactivity levels in the sea as a result of the 0.15 TBq discharge in April 2011.
By the end of June 2011, Tepco had installed 109 concrete panels to seal the water intakes of units 1-4, preventing contaminated water leaking to the harbour. From mid-June some treatment with zeolite of seawater at 30 m 3 /hr was being undertaken near the water intakes for units 2&3, inside submerged barriers installed in April. From October, a steel water shield wall was built on the sea frontage of units 1-4. It extends about one kilometre, and down to an impermeable layer beneath two permeable strata which potentially leak contaminated groundwater to the sea. The inner harbour area which has some contamination is about 30 ha in area. The government in September 2013 said: “At present, statistically-significant increase of radioactive concentration in the sea outside the port of the Tepco’s Fukushima Daiichi NPS has not been detected.” And also: “The results of monitoring of seawater in Japan are constantly below the standard of 10 Bq/L” (the WHO standard for Cs-137 in drinking water). In 2012 the Japanese standard for caesium in food supply was dropped from 500 to 100 Bq/kg. In July-August 2014 only 0.6% of fish caught offshore from the plant exceeded this lower level, compared with 53% in the months immediately following the accident.
Long-term management and disposal
Tepco in 2011 built a new wastewater treatment facility to treat contaminated water. The company used both US proprietary adsorption and French conventional technologies in the new 1200 m 3 /day treatment plant. A supplementary and simpler SARRY (simplified active water retrieve and recovery system) plant to remove caesium using Japanese technology and made by Toshiba and The Shaw Group was installed and commissioned in August 2011. These plants reduce caesium from about 55 MBq/L to 5.5 kBq/L – about ten times better than designed. Desalination is necessary on account of the seawater earlier used for cooling, and the 1200 m 3 /day desalination plant produces 480 m 3 of clean water while 720 m 3 goes to storage. A steady increase in volume of the stored water (about 400 m 3 /d net) is due to groundwater finding its way into parts of the plant and needing removal and treatment.
Some 1000 storage tanks were set up progressively after the accident, including initially 350 steel tanks with rubber seams, each holding 1200 m 3 . A few of these developed leaks in 2013.
Early in 2013 Tepco started to test and commission the Advanced Liquid Processing System ( ALPS ), developed by EnergySolutions and Toshiba. Each of six trains is capable of processing 250 m 3 /day to remove 62 remaining radioisotopes. By the end of 2014, an ALPS of 500 m 3 /d had been added, making total capacity 2000 m 3 /d. The NRA approved the extra capacity in August 2014.
Earlier in September 2013 report from the Atomic Energy Society of Japan recommended diluting the ALPS-treated water with seawater and releasing it to the sea at the legal discharge concentration of 0.06 MBq/L, with monitoring to ensure that normal background tritium levels of 10 Bq/L are not exceeded. (The WHO drinking water guideline is 0.01 MBq/L tritium.) The IAEA supports release of tritiated water to the ocean, as does Dale Klein, chairman of Tepco’s Nuclear Reform Monitoring Committee (NRMC) and former chairman of the US Nuclear Regulatory Commission.
ALPS is a chemical system which removes radionuclides to below legal limits for release. However, because tritium is contained in water molecules, ALPS cannot remove it, which gives rise to questions about the discharge of treated water to the sea. Tritium is a weak beta-emitter which does not bio-accumulate (half-life 12 years), and its concentration has levelled off at about 1 MBq/L in the stored water, with dilution from groundwater balancing further release from the fuel debris.
In 2014 a new Kurion strontium removal system was commissioned. This is mobile and can be moved around the tank groups to further clean up water which has been treated by ALPS.
In June 2015 108 m 3 /day of clean water was being circulated through each reactor (units 1-3). Collected water from them, with high radioactivity levels, was being treated for caesium removal and re-used. Apart from this recirculating loop, the cumulative treated volume was then 1.232 million cubic metres. In storage onsite was 459,000 m 3 of fully treated water (by ALPS), and 190,000 m 3 of partially-treated water (strontium removed), which was being added to at 400 m 3 /day due to groundwater inflow. Almost 600 m 3 of sludge from the water treatment was stored in shielded containers.
In 2016 Kurion (now owned by Veolia) completed a demonstration project for tritium removal at low concentrations, with its new Modular Detritiation System (MDS),* in response to a ¥1 billion commission from METI.
* In this, an electrolyser produces hydrogen and oxygen, with the tritium reporting in the hydrogen. This is fed through a catalytic exchange column with a little water which preferentially takes up the tritium. The concentrated tritiated water is fed through a ‘getter bed’ of dry metal hydrate, where the tritium replaces hydrogen, and the material is stored, being stable up to 500 °C. It can be incorporated into concrete and disposed as low-level waste. The tritium is concentrated 1000 to 20,000 times. The MDS is the first system to be able economically to treat large volumes of water with low tritium concentrations, and builds on existing heavy water tritium removal systems. Each module treats up to 7200 litres per day.
In November 2019 the trade and industry ministry stated that annual radiation levels from the release of the tritium-tainted water are estimated at between 0.052 and 0.62 microsieverts if it were disposed of at sea and 1.3 microsieverts if it were released into the atmosphere, compared with the 2100 microsieverts (2.1 mSv) that humans are naturally exposed to annually.
In March 2020, TEPCO released a study in relation to two disposal methods of the ALPS-treated water – discharge into the sea and vapour discharge.
In April 2021 the Japanese government confirmed that the water would be released into the sea in 2023. In August 2021 Tepco announced plans for the construction of an undersea tunnel about 1 kilometre in length, which will allow the release of the water into an area where no fishing rights are in place. In May 2022 the NRA endorsed Tepco’s plans.
ALPS-treated water is currently stored in more than 1000 tanks onsite with a storage capacity of 1.37 million cubic metres which are expected to reach full capacity by late 2023 or early 2024. Some of the ALPS treated water will require secondary processing to further reduce concentrations of radionuclides in line with government requirements.
In August 2023 the Japanese government asked Tepco to begin preparations for the release of the treated water, with the first water set to be discharged from 24 August 2023. Tepco plans to discharge the water using a two-step process. First, a small amount of treated water will be diluted with seawater and stored in a vertical discharge shaft in order to sample, measure and verify the tritium levels of the water. The water will then be discharged into the sea via the 1km undersea tunnel. The IAEA opened an office at the Fukushima Daiichi plant in July 2023 in order to continuously monitor and assess the activities related to the release of the treated water and ensure alignment with safety standards. The IAEA will also provide real-time monitoring data.
Apart from the above-ground water treatment activity, there is now a groundwater bypass to reduce the groundwater level above the reactors by about 1.5 metres, pumping from 12 wells and from May 2014, discharging the uncontaminated water into the sea. This prevents some of it flowing into the reactor basements and becoming contaminated. In addition, an impermeable wall was constructed on the sea-side of the reactors, and inside this a frozen soil wall was created to further block water flow into the reactor buildings.
In October 2013 guidelines for rainwater release from the site allowed Tepco to release water to the sea without specific NRA approval as long as it conformed to activity limits. Tepco has been working to 25 Bq/L caesium and 10 Bq/L strontium-90.
Summary: A large amount of contaminated water has accumulated onsite and has been treated to remove radioactive elements, apart from tritium. In April 2021, the Japanese government confirmed that the water would be released into the sea. Some radioactivity has already been released to the sea, but this has mostly been low-level and it has not had any significant impact beyond the immediate plant structures. Concentrations outside these structures have been below regulatory levels since April 2011. The release of the treated water will commence in August 2023 and will be continuously monitored by IAEA staff present at the site.
IRID and NDF involvement
The International Research Institute for Nuclear Decommissioning ( IRID ) was set up in August 2013 Japan by the Japan Atomic Energy Agency (JAEA), Japanese utilities and reactor vendors, with a focus on Fukushima Daiichi 1-4.
In September 2013 IRID called for submissions on the management of contaminated water at Fukushima. In particular, proposals were sought for dealing with: the accumulation of contaminated water (in storage tanks, etc ); the treatment of contaminated water including tritium removal; the removal of radioactive materials from the seawater in the plant's 30 ha harbour; the management of contaminated water inside the buildings; measures to block groundwater from flowing into the site; and, understanding the flow of groundwater. Responses were submitted to the government in November.
In December 2013 IRID called for innovative proposals for removing fuel debris from units 1-3 about 2020.
In August 2014 the Nuclear Damage Compensation and Decommissioning Facilitation Corporation (NDF) was set up by the government as a planning body with management support for R&D projects, taking over IRID’s planning role. It works with IRID, whose focus now is on developing mid- and long-term decommissioning technologies. The NDF also works with Tepco Fukushima Daiichi D&D Engineering Co., which has responsibility for operating the actual decommissioning work there. The NDF will be the main body interacting with the government (METI) to implement policy.
Fukushima Daiichi 5&6
Units 5&6, in a separate building, also lost power on 11 March due to the tsunami. They were in 'cold shutdown' at the time, but still requiring pumped cooling. One air-cooled diesel generator at unit 6 was located higher and so survived the tsunami and enabled repairs on Saturday 19, allowing full restoration of cooling for units 5&6. While the power was off their core temperature had risen to over 100 °C (128 °C in unit 5) under pressure, and they had been cooled with normal water injection. They were restored to cold shutdown by the normal recirculating system on 20 March, and mains power was restored on 21-22 March.
In September 2013 Tepco commenced work to remove the fuel from unit 6. Prime minister Abe then called for Tepco to decommission both units. Tepco announced in December 2013 that it would decommission both units from the end of January 2014. Unit 5 was a 760 MWe BWR the same as units 2-4, and unit 6 was larger – 1067 MWe. They entered commercial operation in 1978 and 1979 respectively. It is proposed that they will be used for training.
Remediation on site and decommissioning units 1-4
Tepco published a six- to nine-month plan in April 2011 for dealing with the disabled Fukushima reactors, and updated this several times subsequently. Remediation over the first couple of years proceeded approximately as planned. In August 2011 Tepco announced its general plan for proceeding with removing fuel from the four units, initially from the spent fuel ponds and then from the actual reactors. At the end of 2013 Tepco announced the establishment of an internal entity to focus on measures for decommissioning units 1-6 and dealing with contaminated water. The new company, Fukushima Daiichi Decontamination & Decommissioning Engineering Company, commenced operations in April 2014.
In June 2015 the government revised the decommissioning plan for the second time, though without major change. It clarified milestones to accomplish preventive and multi-layered measures, involving the three principles of removing the source of the contamination, isolating groundwater from the contamination source, and preventing leakage of the contaminated water. It included a new goal of cutting the amount of groundwater flowing into the buildings to less than 100 m 3 per day by April 2016. The schedule for fuel removal from the pond at unit 1 was postponed from late FY17 to FY20, while that for unit 2 was delayed from early FY20 to later the same fiscal year, and that at unit 3 from early FY15 to FY17. Fuel debris removal was to begin in 2021, as before. In September 2017 the government updated the June 2015 decommissioning roadmap, with no changes to the framework, and confirming first removal of fuel debris from unit 1 in 2021. Treatment of all contaminated water accumulated in the reactor buildings was to be completed by 2020.
However, Tepco’s latest roadmap shows fuel removal from the pond at unit 1 is now expected FY27-28, and from unit 2 FY24-FY26. For unit 3, fuel removal was completed in February 2021.
Tepco has a website giving updates on decommissioning work and environmental monitoring.
Storage ponds : Debris has been removed from the upper parts of the reactor buildings using large cranes and heavy machinery. Casks to transfer the removed fuel to the central spent fuel facility have been designed and manufactured using existing cask technology.
In July 2012 two unused fuel assemblies were removed from unit 4 pond, and were found to be in good shape, with no deformation or corrosion. Tepco started removal of both fresh and used fuel from the pond in November 2013, 22 assemblies at a time in each cask, with 1331 used and 202 new ones to be moved. This was uneventful, and the task continued through 2014. By 22 December 2014, all 1331 used as well as all 202 new fuel assemblies had been moved in 71 cask shuttles without incident. All of the radioactive used fuel was removed by early November, eliminating a significant radiological hazard on the site. The used fuel went to the central storage pond, from which older assemblies were transferred to dry cask storage. The fresh fuel assemblies are stored in the pool of the undamaged unit 6.
Tepco completed moving fuel from unit 3 in February 2021. It will now focus on 292 used fuel assemblies and 100 new ones from unit 1, and then 587 used assemblies and 28 new ones from unit 2 will be transferred. The NRA has expressed concern about the unit 1 used fuel.
Reactors 1-3 order of work : The locations of leaks from the primary containment vessels (PCVs) and reactor buildings should first be identified using manual and remotely controlled dosimeters, cameras, etc. , then the conditions inside the PCVs should be indirectly analysed from the outside via measurements of gamma rays, echo soundings, etc . Any leakage points will be repaired and both reactor vessels (RPVs) and PCVs filled with water sufficient to achieve shielding. Then the vessel heads will be removed. The location of melted fuel and corium will then be established. In particular, the distribution of damaged fuel believed to have flowed out from the RPVs into PCVs will be ascertained, and it will be sampled and analysed. After examination of the inside of the reactors, states of the damaged fuel rods and reactor core internals, sampling will be done and the damaged core material will be removed from the RPVs as well as from the PCVs. Updated plans are on the IRID website.
The four reactors will be completely demolished in 30-40 years – much the same timeframe as for any nuclear plant. As noted above, units 5&6 commenced decommissioning in 2014 and will be used for training.
Earlier, consortia led by both Hitachi-GE and Toshiba submitted proposals to Tepco for decommissioning units 1-4. This would generally involve removing the fuel and then sealing the units for a further decade or two while the activation products in the steel of the reactor pressure vessels decay. They can then be demolished. Removal of the very degraded fuel will be a long process in units 1-3, but will draw on experience at Three Mile Island in the USA.
Tepco has allocated ¥207 billion ($2.5 billion) in its accounts for decommissioning units 1-4. In March 2020, Tepco announced that ¥1.37 trillion ($12.6 billion) would be required for the removal of damaged fuel from units 2&3, with no estimates being made for the fuel in unit 1 which suffered the most damage of the three reactors.
The government has allocated ¥1150 billion ($15 billion) for decontamination in the region, with the promise of more if needed.
The Agency for Natural Resources and Energy (ANRE) calculated that as of January 2017 Tepco needed an estimated ¥22,000 billion ($190 billion), double the original estimate, to implement fuel debris removal, cleanup and for compensation to firms and individuals in Fukushima prefecture. Of this amount, Tepco would pay ¥16 trillion. Other Japanese nuclear operators would pay ¥4 trillion through the Nuclear Damage Compensation and Decommissioning Facilitation Corp (NDF), and the Japanese government would pay ¥2 trillion for cleanup in Fukushima prefecture.
A 12-member international expert team assembled by the IAEA at the request of the Japanese government carried out a fact-finding mission in October 2011 on remediation strategies for contaminated land. Its report focused on the remediation of the affected areas outside of the 20 km restricted area. The team said that it agreed with the prioritization and the general strategy being implemented, but advised the government to focus on actual dose reduction. They should "avoid over-conservatism" which "could not effectively contribute to the reduction of exposure doses" to people. It warned the government against being preoccupied with "contamination concentrations ... rather than dose levels," since this "does not automatically lead to reduction of doses for the public." The report also called on the Japanese authorities to "maintain their focus on remediation activities that bring the best results in reducing the doses to the public." A follow-up mission was carried out in October 2013.
Fukushima Daini plant
The four units at Fukushima Daini were shut down automatically due to the earthquake. The tsunami – here only 9 m high – affected the generators and there was major interruption to cooling due to damaged heat exchangers, so the reactors were almost completely isolated from their ultimate heat sink. Damage to the diesel generators was limited and also the earthquake left one of the external power lines intact, avoiding a station blackout as at Daiichi units 1-4. Staff laid and energized 8.8 km of heavy-duty electric cables in 30 hours to supplement power.
In units 1, 2&4 there were cooling problems still evident on Tuesday 15. Unit 3 was undamaged and continued to 'cold shutdown' status on the 12th, but the other units suffered flooding to pump rooms where the equipment transfers heat from the reactor heat removal circuit to the sea. Pump motors were replaced in less than 30 hours. All units achieved 'cold shutdown' by 16 March, meaning core temperature less than 100 °C at atmospheric pressure (101 kPa), but still requiring some water circulation. The almost complete loss of ultimate heat sink for a day proved a significant challenge, but the cores were kept fully covered.
Radiation monitoring figures remained at low levels, little above background.
There was no technical reason for the Fukushima Daini plant not to restart. However, Tepco in October 2012 said it planned to transfer the fuel from the four reactors to used fuel ponds, and this was done. In February 2015 the prime minister said that restarting the four units was essentially a matter for Tepco to decide. In July 2019 Tepco announced its decision to decommission the four reactors.
International Nuclear Event Scale assessment
Japan's Nuclear & Industrial Safety Agency originally declared the Fukushima Daiichi 1-3 accident as level 5 on the International Nuclear Events Scale (INES) – an accident with wider consequences, the same level as Three Mile Island in 1979. The sequence of events relating to the fuel pond at unit 4 was rated INES level 3 – a serious incident.
However, a month after the tsunami the NSC raised the rating to level 7 for units 1-3 together, 'a major accident', saying that a re-evaluation of early radioactive releases suggested that some 630 PBq of I-131 equivalent had been discharged, mostly in the first week. This then matched the criterion for level 7. In early June NISA increased its estimate of releases to 770 PBq, from about half that, though in August the NSC lowered this estimate to 570 PBq.
For Fukushima Daini, NISA declared INES level 3 for units 1, 2, 4 – each a serious incident.
Accident liability and compensation
Beyond whatever insurance Tepco might carry for its reactors is the question of third party liability for the accident. Japan was not party to any international liability convention but its law generally conforms to them, notably strict and exclusive liability for the operator. Early in 2015 Japan ratified the Convention on Supplementary Compensation for Nuclear Damage (CSC). Two laws governing liability are revised about every ten years: the Law on Compensation for Nuclear Damage and Law on Contract for Liability Insurance for Nuclear Damage. Plant operator liability is exclusive and absolute (regardless of fault), and power plant operators must provide a financial security amount of JPY 120 billion ($1.5 billion) – it was half that to 2010. The government may relieve the operator of liability if it determines that damage results from “a grave natural disaster of an exceptional character” (which it did not do here), and in any case total liability is unlimited.
In mid-April 2011, the first meeting was held of a panel to address compensation for nuclear-related damage. The panel established guidelines for determining the scope of compensation for damage caused by the accident, and to act as an intermediary. It was established within the Ministry of Education, Culture, Sports, Science and Technology (MEXT), and was led by law professor Yoshihisa Nomi of Gakushuin University in Tokyo.
On 11 May 2011, Tepco accepted terms established by the Japanese government for state support to compensate those affected by the accident at the Fukushima Daiichi plant. The scheme included a new state-backed institution to expedite payments to those affected by the Fukushima Daiichi accident. The body receives financial contributions from electric power companies with nuclear power plants in Japan, and from the government through special bonds that can be cashed whenever necessary. The government bonds total JPY 5 trillion ($60 billion). Tepco accepted the conditions imposed on the company as part of the package. That included not setting an upper limit on compensation payments to those affected, making maximum efforts to reduce costs, and an agreement to cooperate with an independent panel set up to investigate its management.
This Nuclear Damage Compensation Facilitation Corporation, established by government and nuclear plant operators, includes representatives from other nuclear generators and also operates as an insurer for the industry, being responsible to have plans in place for any future nuclear accidents. The provision for contributions from other nuclear operators is similar to that in the USA. The government estimates that Tepco will be able to complete its repayments in 10 to 13 years, after which it will revert to a fully private company with no government involvement. Meanwhile it will pay an annual fee for the government support, maintain adequate power supplies and ensure plant safety. Tepco estimated its extra costs for fossil fuels in 2011-12 (April-March) would be about JPY 830 billion ($11 billion).
On 14 June 2011, Japan's cabinet passed the Nuclear Disaster Compensation Bill, and a related budget to fund post-tsunami reconstruction was also passed subsequently.
In September 2011 the Nuclear Damage Compensation Facilitation Corporation started by working with Tepco to compile a business plan for the next decade. This was approved by the Ministry of Economy, Trade and Industry (METI) so that some ¥900 billion ($12 billion) could be released to the company through bonds issued to the Nuclear Damage Facilitation Fund to cover compensation payments to March 2012. The plan also involved Tepco reducing its own costs by ¥2545 billion ($33 billion) over the next ten years, including shedding 7400 jobs. This special business plan was superseded by a more comprehensive business plan in March 2012, involving compensation payments of ¥910 billion ($12 billion) annually. Tepco wanted to include an electricity rate increase of 17% in the plan, to cover the additional annual fuel costs for thermal power generation to make up for lost capacity at idled nuclear power plants.
In February 2012 METI approved a further ¥690 billion ($9 billion) in compensation support from the Nuclear Damage Liability Facilitation Fund, subject to Tepco's business plan giving the government voting rights. In June 2012 shareholders voted to sell the Japanese government 50.11% of Tepco's voting shares and an additional 25.73% with no voting rights, for ¥1 trillion (about $13 billion), paid through the Nuclear Damage Liability Facilitation Fund. This was effected at the end of July, so that Tepco then became government-controlled, at least temporarily. Tepco said it appreciated the chance to "transform to New Tepco".
The government and 12 utilities are contributing funds into the new institution to pay compensation to individuals and businesses claiming damages caused by the accident. It received ¥7 billion ($90 million) in public funds as well as a total of ¥7 billion from 12 nuclear plant operators, the Tepco share of ¥2379 million ($30 million) being the largest. The percentage of utility contributions was fixed in proportion to the power output of their plants, so Kansai Electric Power provided ¥1229 million, followed by ¥660 million by Kyushu Electric Power and ¥622 million by Chubu Electric Power. Japan Nuclear Fuel Ltd., which owns a used nuclear fuel reprocessing plant in Aomori prefecture, provided ¥117 million to the entity. The utility companies also pay annual contributions to the body. Tepco is required to make extra contributions, with the specific amount to be decided later.
In June 2013 Tepco requested a further ¥666 billion ($6.7 billion) in government support through the Nuclear Damage Liability Facilitation Fund, bringing the total amount requested by Tepco to ¥3.79 trillion ($40 billion). The company said that more than half of the request – some ¥370 billion ($4 billion) – resulted from the re-evaluation of the evacuation zone around the damaged plant and a re-examination of the estimated amount "regarding compensation for mental damages, loss or depreciation of valuables such as housing lands and buildings." About ¥43 billion ($430 million) was due to a higher estimate of compensation coming from damages to the agriculture, forestry and fisheries industries, as well as the food processing and distribution industries. This, it said, also resulted from "harmful rumours" about the possible health effects of consuming food products from the region near the damaged power plant. As restrictions on the transport of foodstuffs from the Fukushima area seemed set to continue, an additional ¥240 billion ($2 billion) was included to cover for the further compensation claims resulting from this.
By mid-May 2014, Tepco had paid ¥3808 billion ($40 billion) in compensation, fairly evenly split between businesses and individuals, based on decisions of the Nuclear Damage Compensation Facilitation Corporation, and covered by loans from the NDLF Fund. Some $16 billion of this was distributed evenly among 85,000 evacuees – $188,200 each person including children, as directed early in 2011.
In July 2015 the government approved Tepco’s recovery plan, including compensation payments of ¥7075 billion ($57 billion), enabling it to receive ¥950 billion more than the ¥6125 billion estimated in April, according to METI.
As of January 2021 the Fukushima accident evacuees had received ¥9.7 trillion in personal and property compensation.
Earlier, in December 2013 the government raised the upper limit of its financial assistance to Tepco from ¥5 trillion to ¥9 trillion ($86 billion). Early in 2014 the government estimated it would take ¥11 trillion and 40 years to clean up the Fukushima site. The 2013 Japan trade deficit was ¥11.5 trillion.
In September 2019 Tokyo District Court acquitted three former executives – Tsunehisa Katsumata, Ichiro Takekuro and Sakae Muto – with failing to prevent the accident at the company's Fukushima Daiichi plant. The court found all three men not guilty of professional negligence resulting in death and injury and ruled that they could not have foreseen the earthquake and tsunami that led to the meltdown of three of the plant's reactors. In January 2023 the Tokyo High Court upheld the acquittal of the three former executives.
Inquiries and reports: the accident itself and decommissioning
In October 2014 the NRA published its Analysis of the TEPCO Fukushima Daiichi NPS Accident interim report. A provisional translation in English was published in February 2015. This focuses on a number of questions which remained unexplained in the 2012 National Diet Investigation Commission report .
Earlier in May 2011 a team of 18 experts from 12 countries spent a week at the plant on behalf of the International Atomic Energy Agency (IAEA), and that mission's final report was presented to the IAEA Ministerial Conference in Vienna in June. At the IAEA General Conference in 2012 the Director General promised a comprehensive report which would be "an authoritative, factual and balanced assessment, addressing the causes and consequences of the accident as well as the lessons learned." This report on The Fukushima Daiichi Accident was published in August 2015, accompanied by five technical volumes.
In February 2015 the IAEA completed its third review mission (as follow-up to that of late 2013, and involving some 180 experts from 42 IAEA member states and other organizations over two years) and reported on decommissioning to METI. In May 2015 its final report was delivered to member states, and was published in September. It was broadly positive regarding progress since 2013, but said that some challenging issues remain. It contains advisory points on topics such as long-term radioactive waste management, measures concerning contaminated water, and issues related to the removal of used fuel and fuel debris.
The Foreword to the Director General’s report in 2015 states: "A major factor that contributed to the accident was the widespread assumption in Japan that its nuclear power plants were so safe that an accident of this magnitude was simply unthinkable. This assumption was accepted by nuclear power plant operators and was not challenged by regulators or by the government. As a result, Japan was not sufficiently prepared for a severe nuclear accident in March 2011." The accident exposed "certain weaknesses" in Japan's regulatory framework, with responsibilities divided among a number of bodies. It also said there were certain weaknesses "in plant design, in emergency preparedness and response arrangement and in planning for the management of a severe accident".
The Director General said: "I am confident that the legacy of the Fukushima Daiichi accident will be a sharper focus on nuclear safety everywhere. I have seen improvements in safety measures and procedures in every nuclear power plant that I have visited. There is widespread recognition that everything humanly possible must be done to ensure that no such accident ever happens again." He added: "This is all the more essential as global use of nuclear power is likely to continue to grow in coming decades.” Furthermore, "there can be no grounds for complacency about nuclear safety in any country. Some of the factors that contributed to the Fukushima Daiichi accident were not unique to Japan. Continuous questioning and openness to learning from experience are key to safety culture and are essential for everyone involved in nuclear power."
The Executive Summary includes recommendations, but the following paragraphs indicate some salient points from the actual investigation.
Before the accident, there was a basic assumption in Japan that the design of nuclear power plants and the safety measures that had been put in place were sufficiently robust to withstand external events of low probability and high consequences. Because of the basic assumption that nuclear power plants in Japan were safe, there was a tendency for organizations and their staff not to challenge the level of safety. The reinforced basic assumption among the stakeholders about the robustness of the technical design of nuclear power plants resulted in a situation where safety improvements were not introduced promptly.
Before the accident, the operator had conducted some reassessments of extreme tsunami flood levels, using a consensus based methodology developed in Japan in 2002, which had resulted in values higher than the original design basis estimates. Based on the results, some compensatory measures were taken, but they proved to be insufficient at the time of the accident.
There were no indications that the main safety features of the plant were affected by the vibratory ground motions generated by the earthquake on 11 March 2011. This was due to the conservative approach to earthquake design and construction of nuclear power plants in Japan, resulting in a plant that was provided with sufficient safety margins. However, the original design considerations did not provide comparable safety margins for extreme external flooding events, such as tsunamis.
Despite the efforts of the operators at the Fukushima Daiichi nuclear power plant to maintain control, the reactor cores in units 1-3 overheated, the nuclear fuel melted and the three containment vessels were breached. Hydrogen was released from the reactor pressure vessels, leading to explosions inside the reactor buildings in units 1, 3&4 that damaged structures and equipment and injured personnel. Radionuclides were released from the plant to the atmosphere and were deposited on land and on the ocean. There were also direct releases into the sea.
Venting of the containment was necessary to relieve pressure and prevent its failure. The operators were able to vent units 1 and 3 to reduce the pressure in the primary containment vessels. However, this resulted in radioactive releases to the environment. Even though the containment vents for units 1 and 3 were opened, the primary containment vessels for units 1 and 3 eventually failed. Containment venting for unit 2 was not successful, and the containment failed, resulting in radioactive releases.
People within a radius of 20 km of the site and in other designated areas were evacuated, and those within a radius of 20-30 km were instructed to shelter before later being advised to voluntarily evacuate. Restrictions were placed on the distribution and consumption of food and the consumption of drinking water. Many people are still living outside the areas from which they were evacuated.
No early radiation induced health effects were observed among workers or members of the public that could be attributed to the accident.
Earlier reports 2011-2012
Early in June 2011 the independent Investigation Committee on the Accident at the Fukushima Nuclear Power Stations ( ICANPS ), a panel of ten experts, mostly academics and appointed by the Japanese cabinet, began meeting. It had two technological advisers. An initial report was published in December 2011 and a final report in July 2012. The panel set up four teams to undertake investigations on the causes of the accident and ensuing damage and on measures to prevent the further spread of damage caused by the accident, but not to pursue the question of responsibility for the accident.
The national Diet later set up the legally-constituted Nuclear Accident Independent Investigation Commission ( NAIIC , or National Diet Investigation Commission) of ten members which started its work in December 2011. One of the purposes of NAIIC was to provide suggestions including the “re-examination of an optimal administrative organization” for nuclear safety regulation based on its investigation of the accident. The NAIIC reported in July 2012, harshly criticizing the government, the plant operator and the country’s national culture. After conducting 900 hours of public hearings and interviews with more than 1100 people and visiting several nuclear power plants, the commission’s report concluded that the accident was a “manmade disaster,” the result of “collusion between the government, the regulators and Tokyo Electric Power Co.” It said the “root causes were the organizational and regulatory systems that supported faulty rationales for decisions and actions.” The NAIIC criticized the regulator for insufficiently maintaining independence from the industry in developing and enforcing safety regulations, the government for inadequate emergency preparedness and management, and Tepco for its poor governance and lack of safety culture. The report called for fundamental changes across the industry, including the government and regulators, to increase openness, trustworthiness and focus on protecting public health and safety.
The NAIIC Chairman wrote: "What must be admitted – very painfully – is that this was a disaster 'Made in Japan.' Its fundamental causes are to be found in the ingrained conventions of Japanese culture: our reflexive obedience; our reluctance to question authority; our devotion to 'sticking with the programme'; our groupism; and our insularity.” The mindset of the government and industry led the country to avoid learning the lessons of the previous major nuclear accidents at Three Mile Island and Chernobyl. "The consequences of negligence at Fukushima stand out as catastrophic, but the mindset that supported it can be found across Japan. In recognizing that fact, each of us (every Japanese citizen) should reflect on our responsibility as individuals in a democratic society."
The NAIIC reported that Tepco had been aware since 2006 that Fukushima Daiichi could face a station blackout if flooded, as well as the potential loss of ultimate heat sink in the event of a major tsunami. However, the regulator, NISA, gave no instruction to the company to prepare for severe flooding, and even told all nuclear operators that it was not necessary to plan for station blackout. During the initial response to the tsunami, this lack of readiness for station blackout was compounded by a lack of planning and training for severe accident mitigation. Plans and procedures for venting and manual operation of emergency cooling were incomplete and their implementation in emergency circumstances proved very difficult as a result. NISA was also criticized for its "negligence and failure over the years" to prepare for a nuclear accident in terms of public information and evacuation, with previous governments equally culpable. Then Tepco’s difficulty in mitigation was compounded by government interference which undermined NISA.
Earlier, on 7 June 2011 the government submitted a 750-page report to the IAEA compiled by the nuclear emergency task force, acknowledging reactor design inadequacies and systemic shortcomings. It said: "In light of the lessons learned from the accident, Japan has recognized that a fundamental revision of its nuclear safety preparedness and response is inevitable."
On 11 September 2011 a second report was issued by the government and submitted to the IAEA, summarising both onsite work and progress and offsite responses. It contained further analysis of the earthquake and tsunami, the initial responses to manage and cool the reactors, the state of spent fuel ponds and the state of reactor pressure vessels. It also summarised radioactive releases and their effects.
Meanwhile a July 2011 report from MIT's Centre for Advanced Nuclear Energy Systems provided a useful series of observations, questions raised, and suggestions. Its Appendix has some constructive comment on radiation exposure and balancing the costs of dose avoidance in circumstances of environmental contamination.
In November 2011 the US Institute of Nuclear Power Operations (INPO) released its Special Report on the Nuclear Accident at the Fukushima Daiichi Nuclear Power Station , with timeline. This 97-page report gives a detailed account of events.
Also in November 2011 the Japan Nuclear Technology Institute published a 280-page report on the accident, with proposals to be addressed in the future.
On 2 December 2011 Tepco released its interim investigation report on the accident (in Japanese).
The United Nations Scientific Committee on the Effects of Atomic Radiation (UNSCEAR) undertook a 12-month study on the magnitude of radioactive releases to the atmosphere and ocean, and the range of radiation doses received by the public and workers. A follow-up white paper was published in 2016.
An analysis by the Carnegie Endowment in March 2012 said that if best practice from other countries had been adopted by Tepco and NISA at Fukushima, the serious accident would not have happened, underlining the need for greater international regulatory collaboration.
In April 2012 the US Electric Power Research Institute (EPRI) published Fukushima Daiichi Accident – Technical Causal Factor Analysis , which identified the root cause beyond the flooding and its effects as a failure to consider the possibility of the rupture of combinations of geological fault segments in the vicinity of the plant.
Inquiries and reports: radiation effects
A preliminary report from the World Health Organization (WHO) in May 2012 estimated the radiation doses that residents of Japan outside the evacuated areas received in the year following the accident. The report's headline conclusion is that most people in Fukushima prefecture would have received a radiation dose of between 1 and 10 mSv during the first year after the accident. This compares with levels of about 2.4 mSv they would have received from unavoidable natural sources. In two places the doses were higher – between 10 and 50 mSv, still below any harmful level. Almost all were “below the internationally-agreed reference level for the public exposure due to radon in dwellings” (about 10 mSv/yr).
The UN Scientific Committee on the Effects of Atomic Radiation (UNSCEAR) in May 2012 reported that despite skin contamination of several workers, no clinically-observable effects have been reported and there is no evidence of acute radiation injury in any of the 20,115 workers who participated in Tepco’s efforts to mitigate the accident at the plant. Eighteen UNSCEAR member states provided 72 experts for the assessment. UNSCEAR also surveyed Fukushima prefecture to compare its data with Japanese measurements of exposures of some 2 million people living there at the time of the accident.
The results of UNSCEAR’s 12-month study on the magnitude of radioactive releases to the atmosphere and ocean, and the range of radiation doses received by the public and workers were announced in May 2013 are reported above in the subsection above on Radiation exposure .
UNSCEAR’s April 2014 report on radiation effects concluded that the rates of cancer or hereditary diseases were unlikely to show any discernible rise in affected areas because the radiation doses people received were too low. People were promptly evacuated from the vicinity of the nuclear power plant, and later from a neighbouring area where radionuclides had accumulated. This action reduced their radiation exposure by a factor of ten, to levels that were "low or very low." Overall, people in Fukushima are expected on average to receive less than 10 mSv due to the accident over their whole lifetime, compared with the 170 mSv lifetime dose from natural background radiation that people in Japan typically receive. "The most important health effect is on mental and social well-being, related to the enormous impact of the earthquake, tsunami and nuclear accident, and the fear and stigma related to the perceived risk of exposure to radiation." UNSCEAR’s follow-up white paper in October 2015 said that none of the new information appraised after the 2013 report “materially affected the main findings in, or challenged the major assumptions of, the 2013 Fukushima report."
UNSCEAR's update of its 2013 report , published in March 2021, broadly confirms the major findings and conclusions of the 2013 report. It concludes: "The Committee’s revised estimates of dose are such that future radiation-associated health effects are unlikely to be discernible."
In October 2013 a 16-member IAEA mission visited at government request and reported on remediation and decontamination in particular. Its preliminary report said that decontamination efforts were commendable but driven by unrealistic targets.
'Stress tests' on Japanese reactors and new regulatory authority
The government ordered nuclear risk and safety reassessments – so-called 'stress tests' – based on those in the EU for all Japan's nuclear reactors except Fukushima's before they restart following any shutdown, including for routine checks. These were in two stages, and are described in the Japan Nuclear Power information page.
The government then created the separate Nuclear Regulatory Agency (NRA) under the authority of the Environment Ministry and combining the roles of NISA and the NSC, commissioned in September 2012. The new Nuclear Regulatory Commission (NRC) replaced the NSC and will review the effectiveness of the NRA and be responsible for the investigation of nuclear accidents.
Notes & references
General sources.
Tepco NISA IAEA METI JAIF NSC Acton J.M. & Hibbs M, Why Fukushima was preventable , March 2012 Carnegie Paper INPO 11-005 Addendum, Aug 2012, Lessons learned from the Nuclear Accident at Fukushima Daiichi Nuclear Power Station Government’s Decision on Addressing the Contaminated Water Issue at TEPCO’s Fukushima Daiichi NPS , 3 Sept 2013, on Ministry of Foreign Affairs website K. Tateiwa, Decommissioning Fukushima Daiichi NPS, January 2014 UNSCEAR webpage on The Fukushima-Daiichi nuclear power plant accident IAEA Report by the Director General on The Fukushima Daiichi Accident , STI/PUB/1710 (ISBN:978-92-0-107015-9), August 2015 A. Komori, Current status and the future of Fukushima Daiichi NP station , World Nuclear Association 2015 Symposium presentation OECD Nuclear Energy Agency, Fukushima Daiichi Nuclear Power Plant Accident, Ten Years On: Progress, Lessons and Challenges , 2021
Earthquakes and Seismic Protection for Japanese Nuclear Power Plants
Fukushima: radiation exposure, fukushima: reactor background, related information, chernobyl accident, japan: nuclear power, nuclear energy and sustainable development, nuclear power in the world today, you may also be interested in.
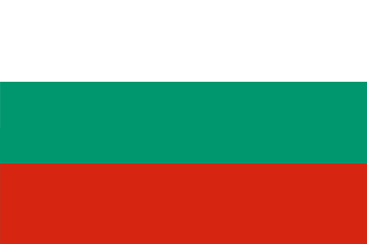
Bulgaria World Nuclear Performance Report 2023
Reactor archive june 2016.

Policy Responses to Climate Change

An official website of the United States government
The .gov means it's official. Federal government websites often end in .gov or .mil. Before sharing sensitive information, make sure you're on a federal government site.
The site is secure. The https:// ensures that you are connecting to the official website and that any information you provide is encrypted and transmitted securely.
- Publications
- Account settings
- Browse Titles
NCBI Bookshelf. A service of the National Library of Medicine, National Institutes of Health.
Committee on Lessons Learned from the Fukushima Nuclear Accident for Improving Safety and Security of U.S. Nuclear Plants; Nuclear and Radiation Studies Board; Division on Earth and Life Studies; National Research Council. Lessons Learned from the Fukushima Nuclear Accident for Improving Safety of U.S. Nuclear Plants. Washington (DC): National Academies Press (US); 2014 Oct 29.
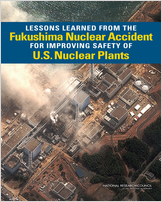
Lessons Learned from the Fukushima Nuclear Accident for Improving Safety of U.S. Nuclear Plants.
- Hardcopy Version at National Academies Press
The March 11, 2011, Great East Japan Earthquake and tsunami sparked a humanitarian disaster in northeastern Japan and initiated a severe nuclear accident at the Fukushima Daiichi nuclear plant. Three of the six reactors at the plant sustained severe core damage and released hydrogen and radioactive materials. Explosion of the released hydrogen damaged three reactor buildings and impeded onsite emergency response efforts.
At the time of the Fukushima Daiichi accident, the Blue Ribbon Commission on America's Nuclear Future was completing an assessment of options for managing spent nuclear fuel and high-level radioactive waste in the United States ( BRC, 2012 ). The Commission recommended that the National Academy of Sciences (NAS) conduct an assessment of lessons learned from the Fukushima Daiichi accident. This recommendation was taken up by the U.S. Congress, which subsequently directed the U.S. Nuclear Regulatory Commission to contract with NAS for this study.
The statement of task for this study is shown in Sidebar S.1 . Study charges 1, 3, and 4 are addressed in this report; study charge 2 (on spent fuel safety and security) will be addressed in a future report.
SIDEBAR S.1
Statement of Task for This National Research Council Study. The National Research Council will provide an assessment of lessons learned from the Fukushima nuclear accident for improving the safety and security of nuclear plants in the United States. This (more...)
A committee of 21 experts was appointed by NAS to carry out this study (see Appendix A ). The committee held 39 meetings during the course of this study to gather information and develop this report (see Appendix B for a list of the committee's information-gathering meetings). One of these meetings was held in Tokyo, Japan, to enable in-depth discussions about the accident with Japanese technical experts from industry, academia, and government. The committee also visited the Fukushima Daini, Fukushima Daiichi, and Onagawa nuclear plants (see Chapter 3 ) to learn about their designs, operations, and responses to the earthquake and tsunami. Subgroups of the committee visited two nuclear plants in the United States that are similar in design to the Fukushima Daiichi plant to learn about their designs and operations.
S.1. CAUSES OF THE FUKUSHIMA DAIICHI ACCIDENT (Study Charge 1)
NAS's examination of the Fukushima Daiichi accident is provided in Chapters 3 and 4 of this report. Chapter 3 describes the March 11, 2011, Great East Japan Earthquake and tsunami and their impacts on Japanese nuclear plants. Chapter 4 describes the accident at the Fukushima Daiichi plant, including the accident time line, key actions taken by plant personnel, and challenges faced in taking those actions. One finding emerged from this examination:
FINDING 4.1 1 : The accident at the Fukushima Daiichi nuclear plant was initiated by the March 11, 2011, Great East Japan Earthquake and tsunami. The earthquake knocked out offsite AC power to the plant and the tsunami inundated portions of the plant site. Flooding of critical plant equipment resulted in the extended loss of onsite AC and DC power with the consequent loss of reactor monitoring, control, and cooling functions in multiple units. Three reactors sustained severe core damage (Units 1, 2, and 3); three reactor buildings were damaged by hydrogen explosions (Units 1, 3, and 4); and offsite releases of radioactive materials contaminated land in Fukushima and several neighboring prefectures. The accident prompted widespread evacuations of local populations and distress of the Japanese citizenry, large economic losses, and the eventual shutdown of all nuclear power plants in Japan. Personnel at the Fukushima Daiichi plant responded with courage and resilience during the accident in the face of harsh circumstances; their actions likely reduced the severity of the accident and the magnitude of offsite radioactive material releases. Several factors prevented plant personnel from achieving greater success—in particular, averting reactor core damage—and contributed to the overall severity of the accident: 1. Failure of the plant owner (Tokyo Electric Power Company) and the principal regulator (Nuclear and Industrial Safety Agency) to protect critical safety equipment at the plant from flooding in spite of mounting evidence that the plant's current design basis for tsunamis was inadequate. 2. The loss of nearly all onsite AC and DC power at the plant—with the consequent loss of real-time information for monitoring critical thermodynamic parameters in reactors, containments, and spent fuel pools and for sensing and actuating critical valves and equipment—greatly narrowed options for responding to the accident. 3. As a result of (1) and (2), the Unit 1, 2 and 3 reactors were effectively isolated from their ultimate heat sink (the Pacific Ocean) for a period of time far in excess of the heat capacity of the suppression pools or the coping time of the plant to station blackout. 4. Multiunit interactions complicated the accident response. Unit operators competed for physical resources and the attention and services of staff in the onsite emergency response center. 5. Operators and onsite emergency response center staff lacked adequate procedures and training for accidents involving extended loss of all onsite AC and DC power, particularly procedures and training for managing water levels and pressures in reactors and their containments and hydrogen generated during reactor core degradation. 6. Failures to transmit information and instructions in an accurate and timely manner hindered responses to the accident. These failures resulted partly from the loss of communications systems and the challenging operating environments throughout the plant. 7. The lack of clarity of roles and responsibilities within the onsite emergency response center and between the onsite and headquarters emergency response centers may have contributed to response delays. 8. Staffing levels at the plant were inadequate for managing the accident because of its scope (affecting several reactor units) and long duration.
S.2. LESSONS LEARNED FOR THE UNITED STATES (Study Charges 3 and 4)
Findings and recommendations on lessons learned from the Fukushima Daiichi accident are provided in Chapters 3 - 7 . They are organized into five sections in this summary.
Seek out and act on new information about hazards.
Improve nuclear plant systems, resources, and training to enable effective ad hoc responses to severe accidents.
Strengthen capabilities for assessing risks from beyond-design-basis events.
Further incorporate modern risk concepts into nuclear safety regulations.
Examine offsite emergency response capabilities and make necessary improvements.
Improve the nuclear safety culture.
S.2.1. Seek Out and Act on New Information About Hazards
FINDING 3.1: The overarching lesson learned from the Fukushima Daiichi accident is that nuclear plant licensees and their regulators must actively seek out and act on new information about hazards that have the potential to affect the safety of nuclear plants. Specifically, 1. Licensees and their regulators must continually seek out new scientific information about nuclear plant hazards and methodologies for estimating their magnitudes, frequencies, and potential impacts. 2. Nuclear plant risk assessments must incorporate new information and methodologies as they become available. 3. Plant operators and regulators must take timely actions to implement countermeasures when such new information results in substantial changes to risk profiles at nuclear plants.
S.2.2. Improve Nuclear Plant Systems, Resources, and Training
Many national governments and international bodies initiated reviews of nuclear plant safety following the Fukushima Daiichi accident (see Table 1.1 in Chapter 1 ). Two major initiatives are now under way in the United States—one by the U.S. Nuclear Regulatory Commission and the other by the U.S. nuclear industry—and are resulting in changes to U.S. nuclear plant systems, operations, and regulations.
FINDING 5.1: Nuclear plant operators and regulators in the United States and other countries have identified and are taking useful actions to upgrade nuclear plant systems, operating procedures, and operator training in response to the Fukushima Daiichi accident. In the United States, these actions include the nuclear industry's FLEX (diverse and flexible coping strategies) initiative as well as regulatory changes proposed by the U.S. Nuclear Regulatory Commission's Near-Term Task Force. Implementation of these actions is still under way; consequently, it is too soon to evaluate their comprehensiveness, effectiveness, or status in the regulatory framework. RECOMMENDATION 5.1A: As the nuclear industry and its regulator implement the actions referenced in Finding 5.1 , they should give specific attention to improving plant systems in order to enable effective responses to beyond-design-basis events, including, when necessary, developing and implementing ad hoc responses 2 to deal with unanticipated complexities. Attention to availability, reliability, redundancy, and diversity of plant systems and equipment is specifically needed for DC power for instrumentation and safety system control; Tools for estimating real-time plant status during loss of power; Decay-heat removal and reactor depressurization and containment venting systems and protocols; Instrumentation for monitoring critical thermodynamic parameters in reactors, containments, and spent fuel pools; Hydrogen monitoring (including monitoring in reactor buildings) and mitigation; Instrumentation for both onsite and offsite radiation and security monitoring; and Communications and real-time information systems to support communication and coordination between control rooms and technical support centers, between control rooms and the field, and between onsite and offsite support facilities The quality and completeness of the changes that result from this recommendation should be adequately peer reviewed. RECOMMENDATION 5.1B: As the nuclear industry and its regulator implement the actions referenced in Finding 5.1 , they should give specific attention to improving resource availability and operator training to enable effective responses to beyond-design-basis events, including, when necessary, developing and implementing ad hoc responses to deal with unanticipated complexities. Attention to the following is specifically needed: 1. Staffing levels for emergencies involving multiple reactors at a site, that last for extended durations, and/or that involve stranded-plant conditions. 3 2. Strengthening and better integrating emergency procedures, extensive damage mitigation guidelines, and severe accident management guidelines, in particular for Coping with the complete loss of AC and DC power for extended periods, Depressurizing reactor pressure vessels and venting containments when DC power and installed plant air supplies (i.e., compressed air and gas) are unavailable, Injecting low-pressure water when plant power is unavailable, Transitioning between reactor pressure vessel depressurization and low-pressure water injection while maintaining sufficient water levels to protect the core from damage, Preventing and mitigating the effects of large hydrogen explosions on cooling systems and containments, and Maintaining cold shutdown in reactors that are undergoing maintenance outages when critical safety systems have been disabled. 3. Training of operators and plant emergency response organizations, in particular, Specific training on the use of ad hoc responses for bringing reactors to safe shutdown during extreme beyond-design-basis events, and More general training to reinforce understanding of nuclear plant system design and operation and enhance operators' capabilities for managing emergency situations. The quality and completeness of the changes that result from this recommendation should be adequately peer reviewed.
S.2.3. Strengthen Capabilities for Assessing Risks from Beyond-Design-Basis Events
A “design-basis event” is a postulated event that a nuclear plant system, including its structures and components, must be designed and constructed to withstand without a loss of functions necessary to protect public health and safety. An event that is “beyond design basis” has characteristics that could challenge the design of plant structures and components and lead to a loss of critical safety functions. The Great East Japan Earthquake and tsunami were beyond-design-basis events.
FINDING 5.2: Beyond-design-basis events—particularly low-frequency, high-magnitude 4 (i.e., extreme) events—can produce severe accidents at nuclear plants that damage reactor cores and stored spent fuel. Such accidents can result in the generation and combustion of hydrogen within the plant and release of radioactive material to the offsite environment. There is a need to better understand the safety risks 5 that arise from such events and take appropriate countermeasures to reduce them. RECOMMENDATION 5.2A: The U.S. nuclear industry and the U.S. Nuclear Regulatory Commission should strengthen their capabilities for identifying, evaluating, and managing the risks from beyond-design-basis events. Particular attention is needed to improve the identification of such events; better account for plant system interactions and the performance of plant operators and other critical personnel in responding to such events; and better estimate the broad range of offsite health, environmental, economic, and social consequences that can result from such events. RECOMMENDATION 5.2B: The U.S. Nuclear Regulatory Commission should support industry's efforts to strengthen its capabilities by providing guidance on approaches and by overseeing independent review by technical peers (i.e., peer review). RECOMMENDATION 5.2C: As the U.S. nuclear industry and the U.S. Nuclear Regulatory Commission carry out the actions in Recommendation 5.2A , they should pay particular attention to the risks from beyond-design-basis events that have the potential to affect large geographic regions and multiple nuclear plants. These include earthquakes, tsunamis and other geographically extensive floods, and geomagnetic disturbances.
S.2.4. Further Incorporate Modern Risk Concepts into Nuclear Safety Regulations
A design-basis accident is a stylized accident—for example, a loss-of-coolant accident or transient overpower accident—that is required (by regulation) to be considered in a reactor system's design. The Fukushima Daiichi accident was a beyond-design-basis accident. Other major nuclear accidents (Three Mile Island in 1979 and Chernobyl in 1986) are also considered to be beyond-design-basis accidents.
FINDING 5.3: Four decades of analysis and operating experience have demonstrated that nuclear plant core-damage risks are dominated by beyond-design-basis accidents. Such accidents can arise, for example, from multiple human and equipment failures, violations of operational protocols, and extreme external events. Current approaches for regulating nuclear plant safety, which traditionally have been based on deterministic concepts such as the design-basis accident, are clearly inadequate for preventing core-melt accidents and mitigating their consequences. Modern risk assessment principles are beginning to be applied in nuclear reactor licensing and regulation. The more complete application of these principles in licensing and regulation could help to further reduce core-melt risks and their consequences and enhance the overall safety of all nuclear plants, especially currently operating plants. RECOMMENDATION 5.3: The U.S. Nuclear Regulatory Commission should further incorporate modern risk concepts into its nuclear reactor safety regulations. This effort should utilize the strengthened capabilities for identifying and evaluating risks that are described in Recommendation 5.2A .
The committee uses the term “modern risk concepts” to mean risk that is defined in terms of the risk triplet (What can go wrong? How likely is that to happen? What are the consequences if it does happen?) and subject to the limitations for quantitative analyses discussed in Section 5.2 in Chapter 5 . Implementing this recommendation fully would likely require changes to some current U.S. Nuclear Regulatory Commission regulatory procedures, for example, those used for backfit analyses.
S.2.5. Examine Offsite Emergency Response Capabilities and Make Necessary Improvements
Emergency response to the Fukushima Daiichi accident was greatly inhibited by the widespread and severe destruction caused by the March 11, 2011, earthquake and tsunami. Japan is known to be well prepared for natural hazards; however, the earthquake and tsunami caused devastation on a scale beyond what was expected and prepared for. Twenty prefectures on three of Japan's major islands (Hokkaido, Honshu, and Shikoku) were affected by the earthquake and tsunami.
FINDING 6.1: The Fukushima Daiichi accident revealed vulnerabilities in Japan's offsite emergency management. The competing demands of the earthquake and tsunami diminished the available response capacity for the accident. Implementation of existing nuclear emergency plans was overwhelmed by the extreme natural events that affected large regions, producing widespread disruption of communications, electrical power, and other critical infrastructure over an extended period of time. Additionally: Emergency management plans in Japan at the time of the Fukushima Daiichi accident were inadequate to deal with the magnitude of the accident, requiring emergency responders to improvise. Decision-making processes by government and industry officials were challenged by the lack of reliable, real-time information on the status of the plant, offsite releases, accident progression, and projected doses to nearby populations. Coordination among the central and local governments was hampered by limited and poor communications. Protective actions were improvised and uncoordinated, particularly when evacuating vulnerable populations (e.g., the elderly and sick) and providing potassium iodide. Different and revised radiation standards and changes in decontamination criteria and policies added to the public's confusion and distrust of the Japanese government. Cleanup of contaminated areas and possible resettlement of populations are ongoing efforts 3 years after the accident with uncertain completion time lines and outcomes. Failure to prepare and implement an effective strategy for communication during the emergency contributed to the erosion of trust among the public for Japan's government, regulatory agencies, and the nuclear industry. FINDING 6.2: The committee did not have the time or resources to perform an in-depth examination of U.S. preparedness for severe nuclear accidents. Nevertheless, the accident raises the question of whether a severe nuclear accident such as occurred at the Fukushima Daiichi plant would challenge U.S. emergency response capabilities because of its severity, duration, and association with a regional-scale natural disaster. The natural disaster damaged critical infrastructure and diverted emergency response resources. RECOMMENDATION 6.2A: The nuclear industry and organizations with emergency management responsibilities in the United States should assess their preparedness for severe nuclear accidents associated with offsite regional-scale disasters. Emergency response plans, including plans for communicating with affected populations, should be revised or supplemented as necessary to ensure that there are scalable and effective strategies, well-trained personnel, and adequate resources for responding to long-duration accident and/or disaster scenarios involving Widespread loss of offsite electrical power and severe damage to other critical offsite infrastructure, for example, communications, transportation, and emergency response infrastructure; Lack of real-time information about conditions at nuclear plants, particularly with respect to releases of radioactive material from reactors and/or spent fuel pools; and Dispersion of radioactive materials beyond the 10-mile emergency planning zones for nuclear plants that could result in doses exceeding one or more of the protective action guidelines. RECOMMENDATION 6.2B: The nuclear industry and organizations with emergency management responsibilities in the United States should assess the balance of protective actions (e.g., sheltering in place, evacuation, relocation, and distribution of potassium iodide) for offsite populations affected by severe nuclear accidents and revise the guidelines as appropriate. Particular attention should be given to the following issues: Protective actions for special populations (children, ill, elderly) and their caregivers; Long-term impacts of sheltering in place, evacuation and/or relocation, including social, psychological and economic impacts; and Decision making for resettlement of evacuated populations in areas contaminated by radioactive material releases from nuclear plant accidents.
S.2.6. Improve the Nuclear Safety Culture
The term “safety culture” is generally understood to encompass a set of attitudes and practices that emphasize safety over competing goals such as production or costs. There is universal acceptance by the nuclear community that safety culture practices need to be adopted by regulatory bodies and other organizations that set nuclear power policies; by senior management of organizations operating nuclear power plants; and by individuals who work in those plants.
FINDING 7.1: While the Government of Japan acknowledged the need for a strong nuclear safety culture prior to the Fukushima Daiichi accident, TEPCO and its nuclear regulators were deficient in establishing, implementing, and maintaining such a culture. Examinations of the Japanese nuclear regulatory system following the Fukushima Daiichi accident concluded that regulatory agencies were not independent and were subject to regulatory capture. 6 FINDING 7.2: The establishment, implementation, maintenance, and communication of a nuclear safety culture in the United States are priorities for the U.S. nuclear power industry and the U.S. Nuclear Regulatory Commission. The U.S. nuclear industry, acting through the Institute of Nuclear Power Operations, has voluntarily established nuclear safety culture programs and mechanisms for evaluating their implementation at nuclear plants. The U.S. Nuclear Regulatory Commission has published a policy statement on nuclear safety culture, but that statement does not contain implementation steps or specific requirements for industry adoption. RECOMMENDATION 7.2A: The U.S. Nuclear Regulatory Commission and the U.S. nuclear power industry must maintain and continuously monitor a strong nuclear safety culture in all of their safety-related activities. Additionally, the leadership of the U.S. Nuclear Regulatory Commission must maintain the independence of the regulator. The agency must ensure that outside influences do not compromise its nuclear safety culture and/or hinder its discussions with and disclosures to the public about safety-related matters. RECOMMENDATION 7.2B: The U.S. nuclear industry and the U.S. Nuclear Regulatory Commission should examine opportunities to increase the transparency of and communication about their efforts to assess and improve their nuclear safety cultures.
All committee members agree with the safety culture findings and recommendations (i.e., Findings 7.1 - 7.2 and Recommendations 7.2A , B ), but members have a range of views about the current status of the nuclear safety culture in the United States. A selection of views is provided in Section 7.4 in Chapter 7 .
The first digit denotes the chapter in which the finding (or recommendation) appears; the second digit denotes the serial order of the finding (or recommendation) in the chapter.
The term “ad hoc” refers to responses that are not planned and trained on in advance but rather are developed on the spot.
That is, when the plant is cut off from outside supply of materials and personnel.
The term “extreme event” refers to high-magnitude environmental events, such as large earthquakes or floods, that occur very infrequently, for example, on the order of once every few centuries to millennia. The Great East Japan Earthquake and tsunami are examples of extreme events.
Risk is defined and discussed in Appendix I.
The term “regulatory capture” refers to the processes by which regulated entities manipulate regulators to put their interests ahead of public interests.
- Cite this Page Committee on Lessons Learned from the Fukushima Nuclear Accident for Improving Safety and Security of U.S. Nuclear Plants; Nuclear and Radiation Studies Board; Division on Earth and Life Studies; National Research Council. Lessons Learned from the Fukushima Nuclear Accident for Improving Safety of U.S. Nuclear Plants. Washington (DC): National Academies Press (US); 2014 Oct 29. Summary.
- PDF version of this title (20M)
In this Page
- CAUSES OF THE FUKUSHIMA DAIICHI ACCIDENT (Study Charge 1)
- LESSONS LEARNED FOR THE UNITED STATES (Study Charges 3 and 4)
Recent Activity
- Summary - Lessons Learned from the Fukushima Nuclear Accident for Improving Safe... Summary - Lessons Learned from the Fukushima Nuclear Accident for Improving Safety of U.S. Nuclear Plants
Your browsing activity is empty.
Activity recording is turned off.
Turn recording back on
Connect with NLM
National Library of Medicine 8600 Rockville Pike Bethesda, MD 20894
Web Policies FOIA HHS Vulnerability Disclosure
Help Accessibility Careers
- History Classics
- Your Profile
- Find History on Facebook (Opens in a new window)
- Find History on Twitter (Opens in a new window)
- Find History on YouTube (Opens in a new window)
- Find History on Instagram (Opens in a new window)
- Find History on TikTok (Opens in a new window)
- This Day In History
- History Podcasts
- History Vault
By: History.com Editors
Updated: April 23, 2021 | Original: April 24, 2018
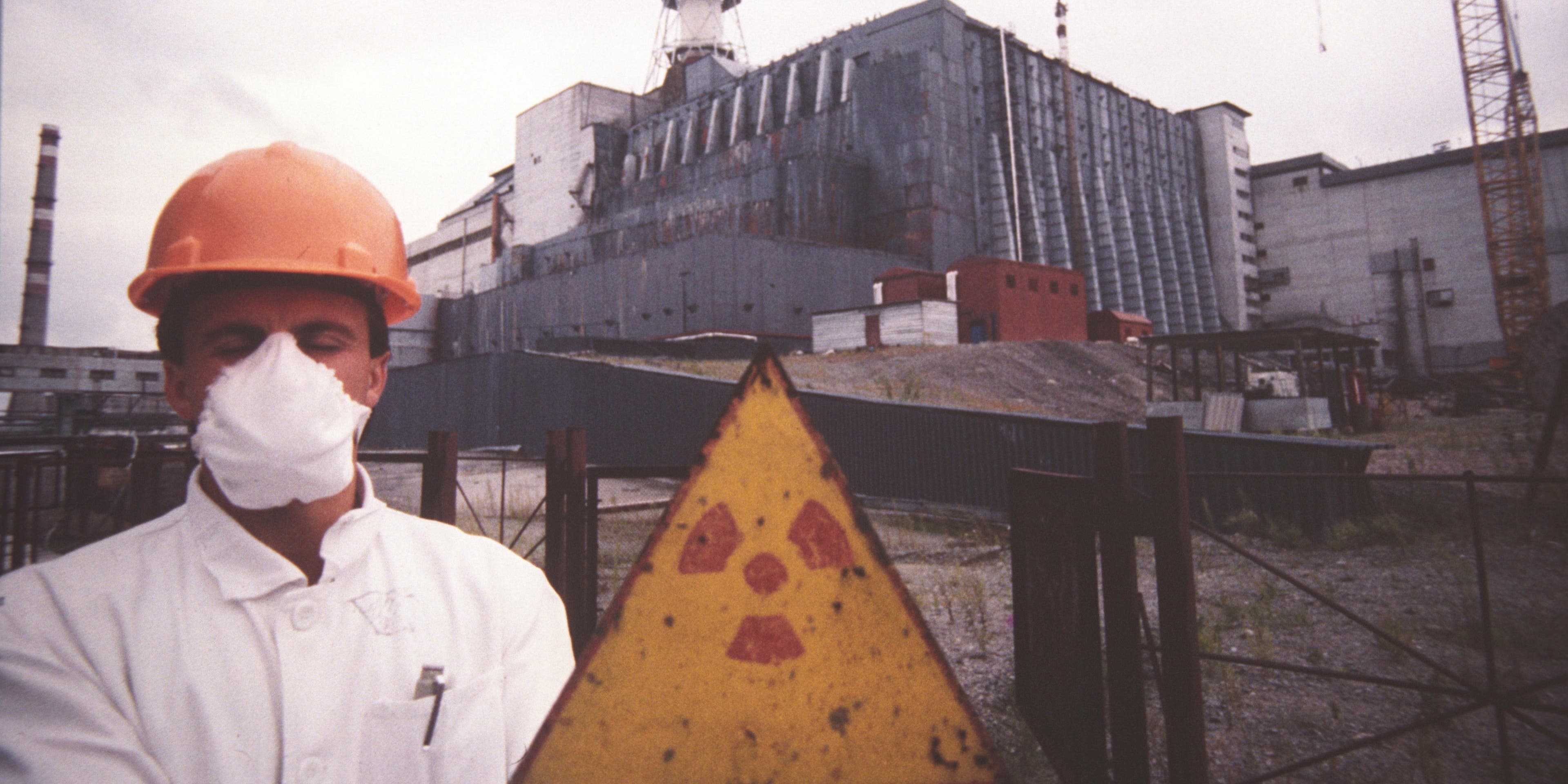
Chernobyl is a nuclear power plant in Ukraine that was the site of a disastrous nuclear accident on April 26, 1986. A routine test at the power plant went horribly wrong, and two massive explosions blew the 1,000-ton roof off one of the plant’s reactors, releasing 400 times more radiation than the atomic bomb dropped on Hiroshima. The worst nuclear disaster in history killed two workers in the explosions and, within months, at least 28 more would be dead by acute radiation exposure. Eventually, thousands of people would show signs of health effects—including cancer—from the fallout.
The Chernobyl disaster not only stoked fears over the dangers of nuclear power, it also exposed the Soviet government’s lack of openness to the Soviet people and the international community. The meltdown and its aftermath drained the Soviet Union of billions in clean-up costs, led to the loss of a primary energy source and dealt a serious blow to national pride.
Then-Soviet leader Mikhail Gorbachev would later say that he thought the Chernobyl meltdown, “even more than my launch of perestroika , was perhaps the real cause of the collapse of the Soviet Union five years later.”
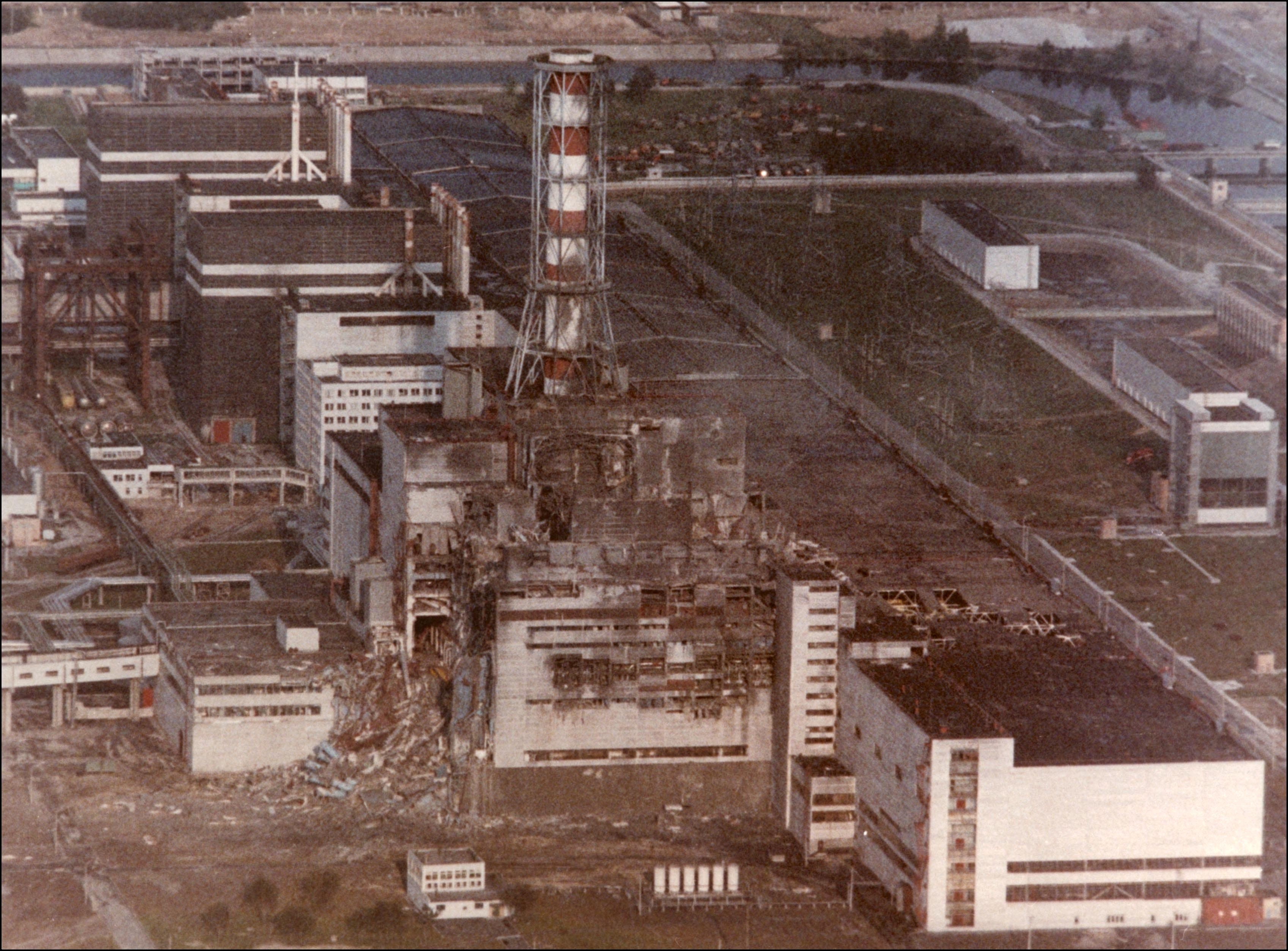
Where Is Chernobyl?
Chernobyl is located in northern Ukraine, about 80 miles north of Kiev. A small town, Pripyat, was constructed a few miles from the site of the nuclear plant to accommodate workers and their families.
Construction of the Chernobyl power plant began in 1977, when the country was still part of the Soviet Union. By 1983, four reactors had been completed, and the addition of two more reactors was planned in subsequent years.
What Happened at Chernobyl?
A routine exercise to test whether an emergency water cooling system would work during a power loss started at 1:23 a.m. on April 26.
Within seconds, an uncontrolled reaction caused pressure to build up in Reactor No. 4 in the form of steam. The steam blasted the roof off the reactor, releasing plumes of radiation and chunks of burning, radioactive debris.
About two to three seconds later, a second explosion hurled out additional fuel. A fire started at the roof of Reactor No. 3, risking a breach at that facility. Automatic safety systems that would normally have kicked into action did not because they had been shut down prior to the test.
READ MORE: Chernobyl Disaster: The Meltdown by the Minute
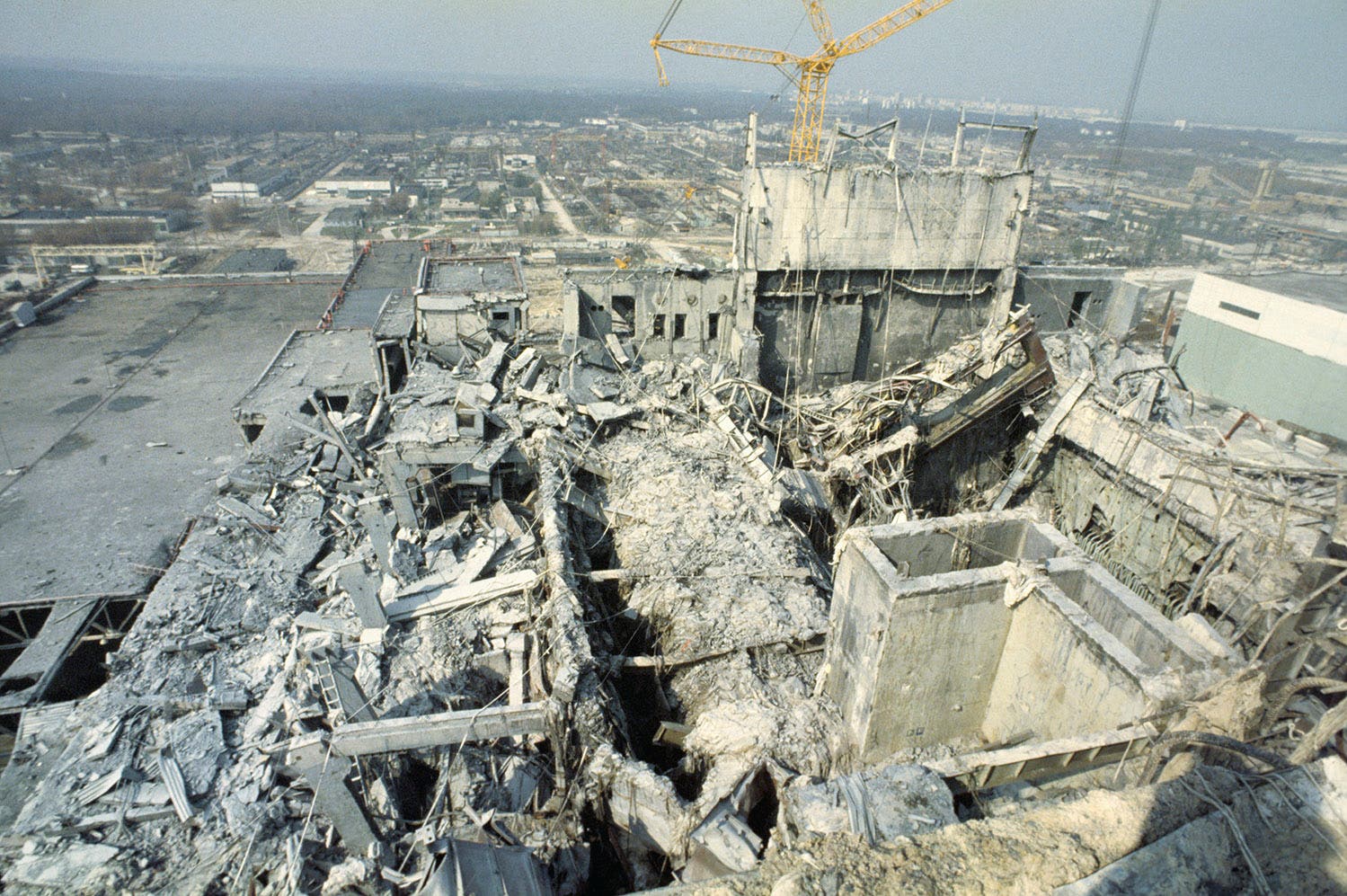
Firefighters arrived at the scene within minutes and began to fight the blaze without gear to protect them from radiation. Many of them would soon number among the 28 killed by acute radiation exposure.
Eyewitness accounts of the firefighters who had helped battle the fires described the radiation as “tasting like metal,” and feeling pain like pins and needles on their faces, according to the CBC documentary series, Witness . Days later, many of those firefighters would be dead.
It wasn’t until 5 a.m. the following day that Reactor No. 3 was shut down. Some 24 hours later, Reactors No. 1 and 2 were also shut down.
By the afternoon of April 26, the Soviet government had mobilized troops to help fight the blaze. Some were dropped at the rooftop of the reactor to furiously shovel debris off the facility and spray water on the exposed reactor to keep it cool.
The workers were picked up within seconds to minimize their radiation exposure. It would take nearly two weeks to extinguish all the fires using sand, lead and nitrogen.
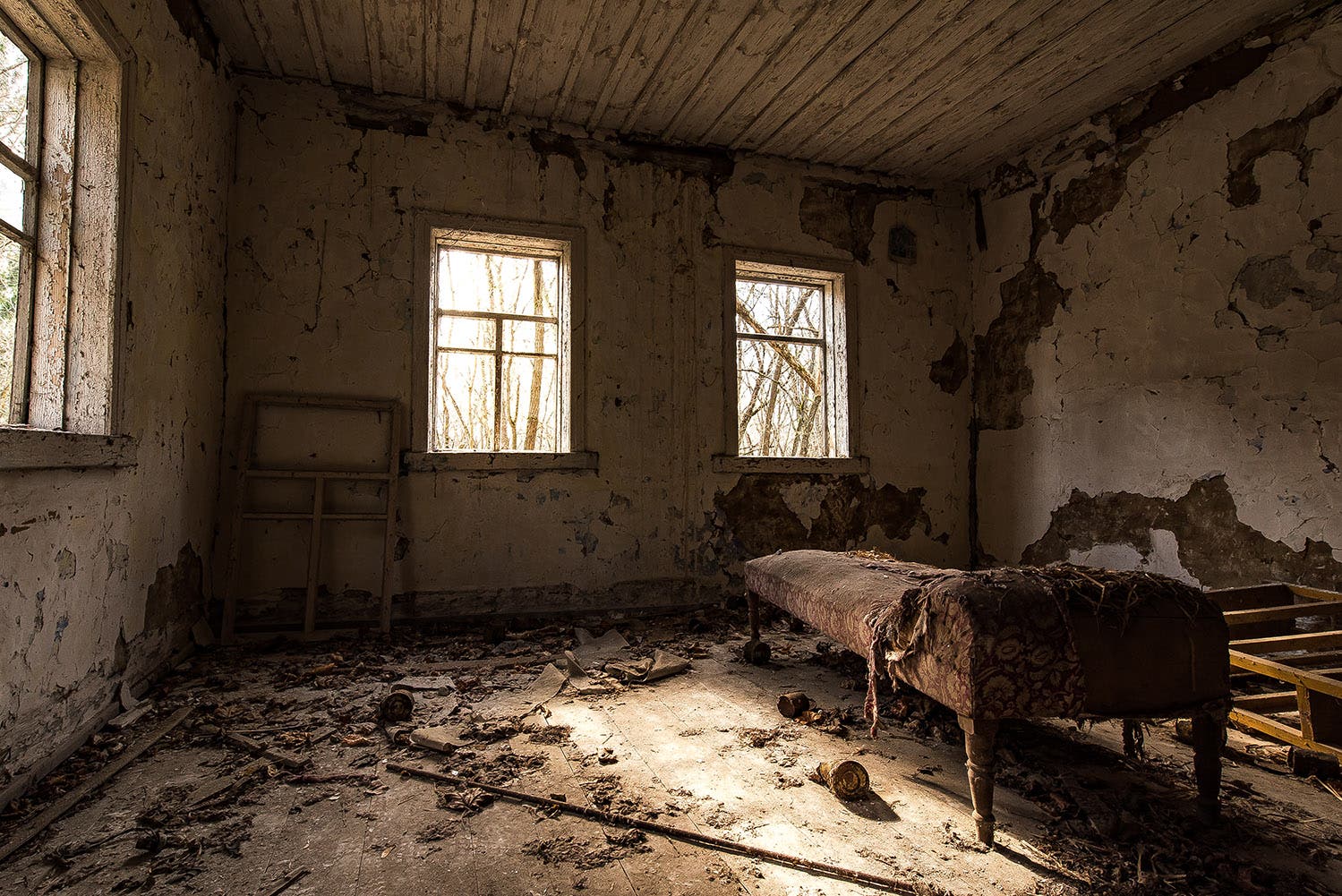

Pripyat Evacuated
Meanwhile, life went on as usual for almost a day in the neighboring town of Pripyat. Aside from the sight of trucks cleaning the streets with foam, there were initially few signs of the disaster unfolding just miles away.
It wasn’t until the next day, April 27, when the government began evacuations of Pripyat’s 50,000 residents. Residents were told they would be away for just a few days, so they took very little with them. Most would never return to their homes.
Soviet Secrecy
It took days for Soviet leadership to inform the international community that the disaster had occurred. The Soviet government made no official statement about the global-scale accident until Swedish leaders demanded an explanation when operators of a nuclear power plant in Stockholm registered unusually high radiation levels near their plant.
Finally, on April 28, the Kremlin reported that there had been an accident at Chernobyl and that authorities were handling it. The statement was followed by a state broadcast detailing the U.S. nuclear accident at Three Mile Island and other nuclear incidents in western countries.
Three days later, Soviet May Day parades to celebrate workers went ahead as usual in Moscow, Kiev and Belarus’ capital Minsk—even as hazardous amounts of radiation were still streaming from the wrecked power plant.
Most people, even within the Ukraine, were still unaware of the accident, the deaths, and the hasty evacuations of Pripyat.
READ MORE: The Chernobyl Cover-Up: How Officials Botched Evacuating an Irradiated City
Chernobyl Disaster Spewed Radiation
The damaged plant released a large quantity of radioactive substances, including iodine-131, cesium-137, plutonium and strontium-90, into the air for over a period of 10 days.
The radioactive cloud was deposited nearby as dust and debris, but was also carried by wind over the Ukraine, Belarus, Russia, Scandinavia and other parts of Europe.
In an attempt to contain the fallout, on May 14, Soviet leader Mikhail Gorbachev ordered the dispatch of hundreds of thousands of people, including firefighters, military reservists and miners, to the site to aid in clean-up. The corps worked steadily, often with inadequate protective gear, through 1989 to clear debris and contain the disaster.
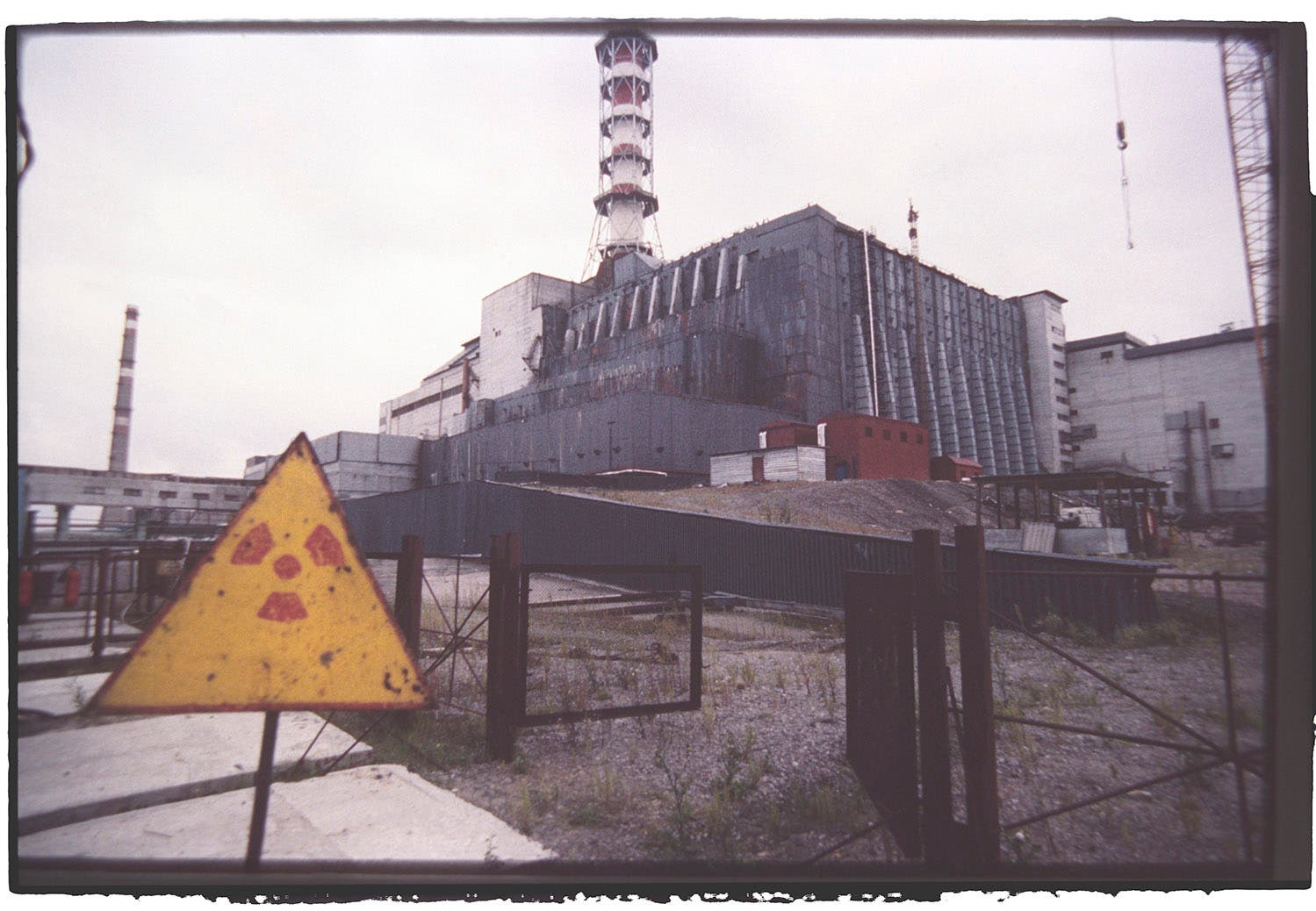
Chernobyl Sarcophagus
Over a hurried construction period of 206 days, crews erected a steel and cement sarcophagus to entomb the damaged reactor and contain any further release of radiation.
As former liquidator, Yaroslav Melnik, told the BBC in January 2017, “We worked in three shifts, but only for five to seven minutes at a time because of the danger. After finishing, we’d throw our clothes in the garbage.”
Starting in 2010, an international consortium organized the building of a bigger, more secure sarcophagus for the site. The 35,000-ton New Safe Confinement was built on tracks and then slid over the damaged reactor and existing sarcophagus in November 2016.
After the installation of the new structure, radiation near the plant dropped to just one-tenth of previous levels, according to official figures. The structure was designed to contain the radioactive debris for 100 years.
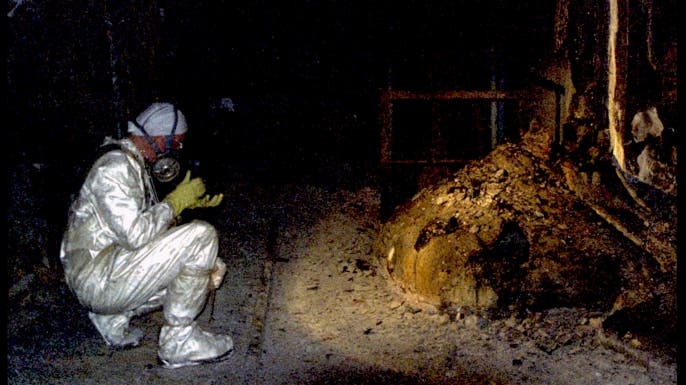
Chernobyl Elephant’s Foot
Deep within the basement of Reactor 4 lies the Chernobyl Elephant’s Foot, a huge mass of melted concrete, sand and highly radioactive nuclear fuel.
The mass was named for its wrinkled appearance, which reminded some observers of the wrinkled skin of an elephant’s leg and foot.
In the 1980s, the Elephant’s Foot gave off an estimated 10,000 roentgens of radiation each hour, enough to kill a person three feet away in less than two minutes. By 2001, that rate had dropped to roughly 800 roentgens per hour.
How Many People Died in Chernobyl?
Ukraine’s government declared in 1995 that 125,000 people had died from the effects of Chernobyl radiation. A 2005 report from the United Nations Chernobyl Forum estimated that while fewer than 50 people were killed in the months following the accident, up to 9,000 people could eventually die from excess cancer deaths linked to radiation exposure from Chernobyl.
As of 2005, according to the Union of Concerned Scientists , some 6,000 thyroid cancers and 15 thyroid cancer deaths had been attributed to Chernobyl.
Health effects from the Chernobyl disaster remain unclear, apart from the initial 30 people the Soviet government confirmed killed from the explosions and acute radiation exposure. No official government studies were conducted following the explosion to assess its effects on workers, the liquidators and nearby populations.
A 2011 study by the U.S. National Institutes of Health concluded that exposure to radioactive iodine-131 from Chernobyl fallout was likely responsible for thyroid cancers that were still being reported among people who were children or adolescents at the time of the accident.
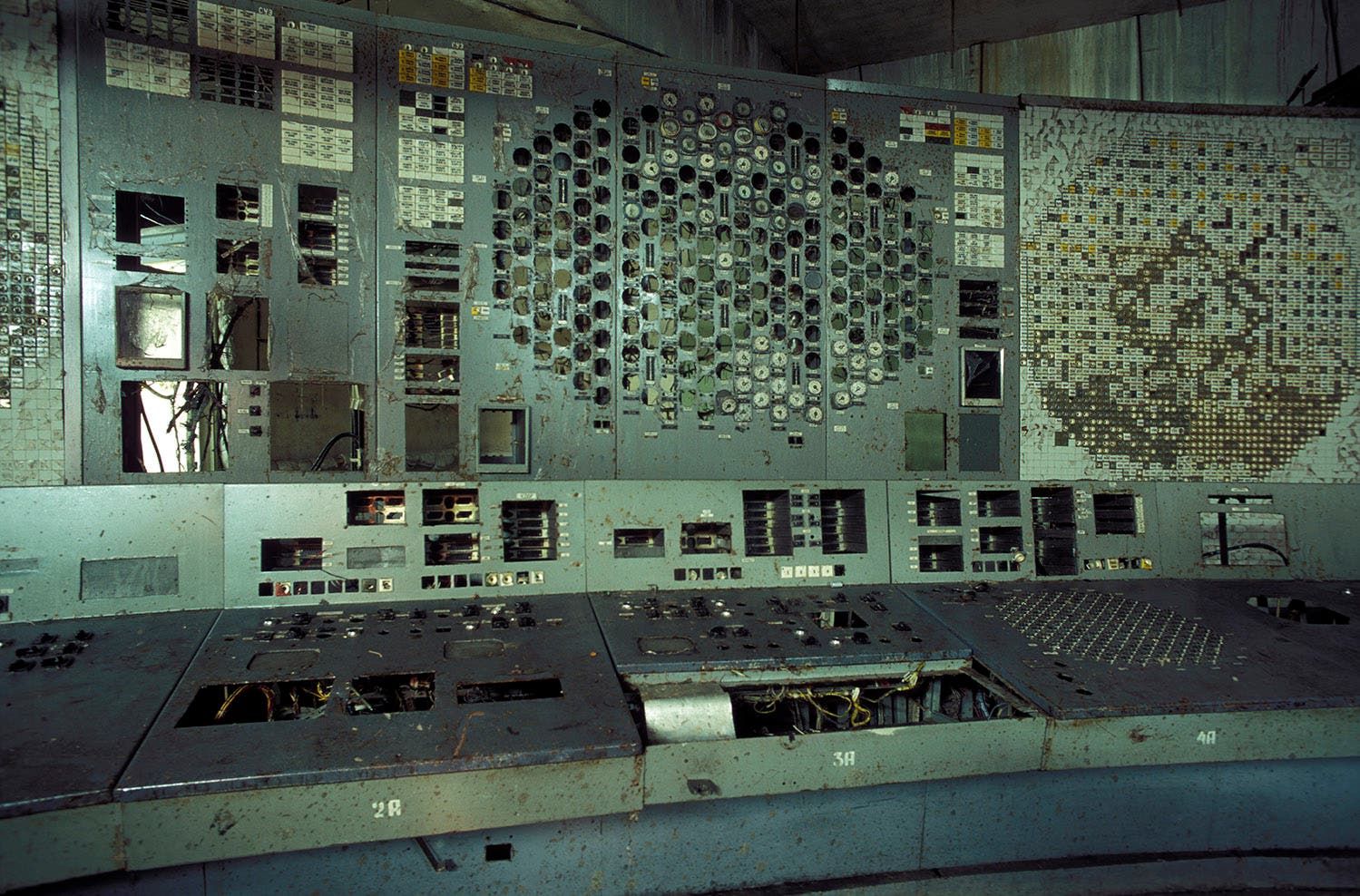
Chernobyl Exclusion Zone
Apart from the ever-unfolding human toll from the disaster, the Chernobyl accident also left behind a huge area of radiation-tainted land.
A 770-mile-wide Chernobyl Exclusion Zone around the site isn’t considered safe for human habitation and can’t be used for logging or agriculture due to contaminated plants and soil. By 2017, however, entrepreneurs found a new use for the territory.
In December 2017, a Ukrainian-German company, Solar Chernobyl, announced construction of a massive solar power plant in the abandoned territory. The one-megawatt power plant, built just a few hundred feet from the damaged Reactor 4, was fitted with 3,800 photovoltaic panels. The Ukrainian government said that a collection of companies planned to eventually develop up to 99 more megawatts of solar power at the site.
That’s a lot of power, but still not close to the former output of the ruined nuclear power plant. At the time of the accident Chernobyl’s four reactors could generate 1,000 megawatts each .
Chernobyl Animals Thrive
Meanwhile, wildlife, including boars, wolves, beavers and bison, showed signs of flourishing at the Chernobyl site, according to an April 2016 study .
The researchers pointed out that while radiation exposure couldn’t be good for the animals, the benefits of the absence of humans outweighed radiation risk.
Chernobyl Today
Humans, on the other hand, aren’t expected to repopulate the area any time soon. Ukrainian authorities have said it will not be safe for people to live in the Chernobyl Exclusion Zone for more than 24,000 years.
Today tourists can visit the site, which appears frozen in time, apart from signs of looting, natural weathering and the encroachment of nature.
“Chernobyl: The True Scale of the Accident,” September 5, 2005, World Health Organization . Chernobyl Accident 1986, updated November 2016, World Nuclear Association “Health Effects of the Chernobyl Accident: An Overview,” April 2006, World Health Organization . “Chernobyl’s Legacy 30 Years On,” by Tom Burridge, April 26, 2016, BBC News “Higher Cancer Risk Continues After Chernobyl,” March 17, 2011, National Institutes of Health . “How Many Cancer Deaths Did Chernobyl Really Cause?” by Lisbeth Gronlund, Union of Concerned Scientists . “Animals Rule Chernobyl Three Decades After Nuclear Disaster,” by John Wendle, April 18, 2016, National Geographic . “A Nuclear Disaster That Brought Down an Empire,” April 26, 2016, The Economist . “World’s Largest Moveable Steel Structure Shelters Sarcophagus at Chernobyl,” April 27, 2017, PhysOrg/Pacific Northwest National Laboratory . “Pictures: ‘Liquidators’ Endured Chernobyl 25 Years Ago,” by Marianne Lavelle, April 27, 2011, National Geographic . “Chernobyl: Timeline of a Nuclear Nightmare,” by Kim Hjelmgaard, USA Today . “A Vast New Tomb for the Most Dangerous Disaster Site in the World,” by Christian Borys, January 3, 2017, BBC Future Now . “The Lessons of Chernobyl May Be Different Than We Thought,” by Ryan Faith, April 26, 2016, Vice News . “25 Years After Chernobyl, We Don’t Know How Many Died,” by Roger Highfield, April 21, 2011, New Scientist . “Chernobyl’s Transformation Into a Massive Solar Plant Is Almost Complete,” by David Nield, January 13, 2018, Science Alert . “The Famous Photo of Chernobyl’s Most Dangerous Radioactive Material Was a Selfie.” January 24, 2016, Atlas Obscura .
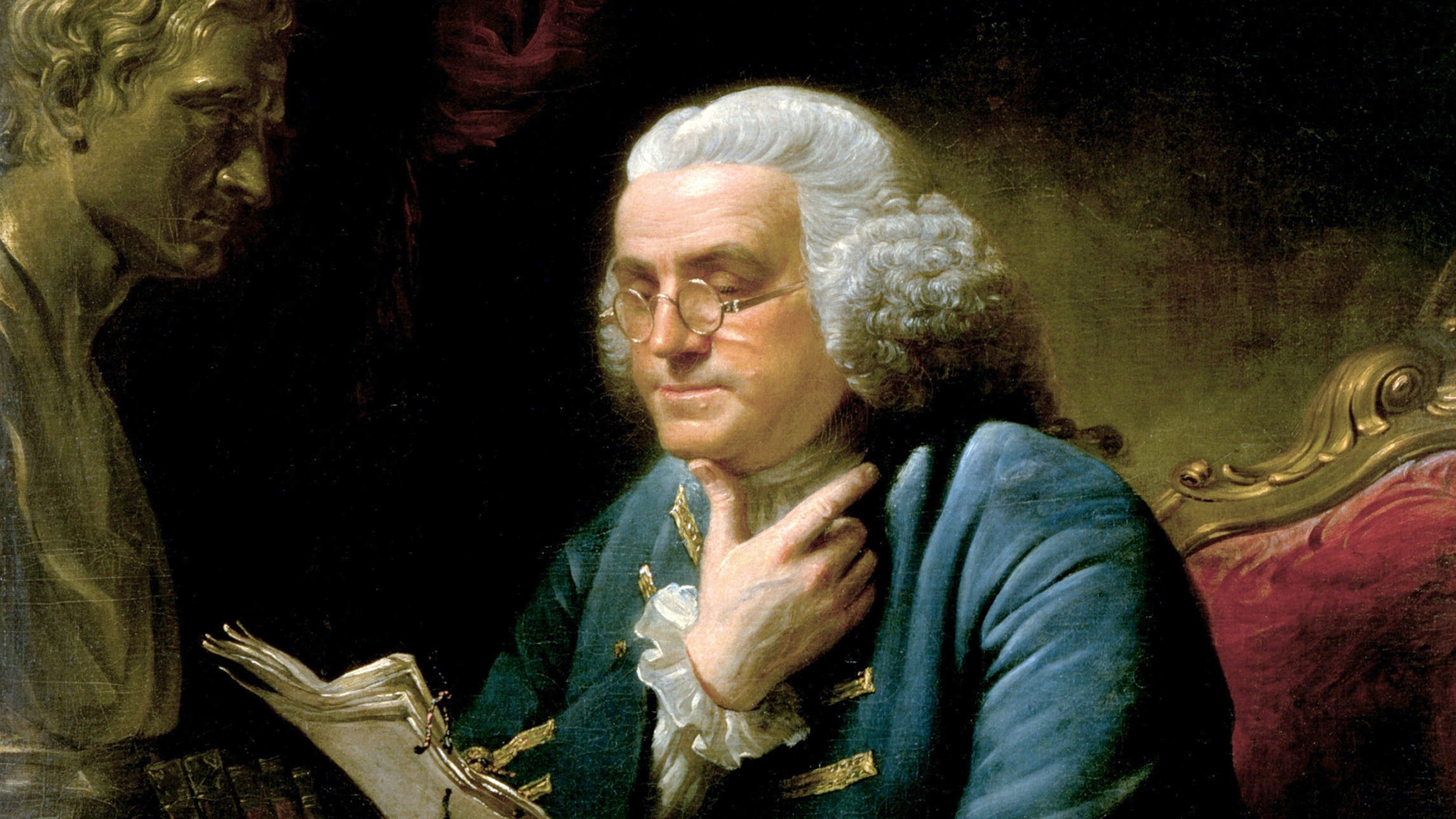
Sign up for Inside History
Get HISTORY’s most fascinating stories delivered to your inbox three times a week.
By submitting your information, you agree to receive emails from HISTORY and A+E Networks. You can opt out at any time. You must be 16 years or older and a resident of the United States.
More details : Privacy Notice | Terms of Use | Contact Us
- Publications
- Conferences & Events
- Professional Learning
- Science Standards
- Awards & Competitions
- Daily Do Lesson Plans
- Free Resources
- American Rescue Plan
- For Preservice Teachers
- NCCSTS Case Collection
- Partner Jobs in Education
- Interactive eBooks+
- Digital Catalog
- Regional Product Representatives
- e-Newsletters
- Bestselling Books
- Latest Books
- Popular Book Series
- Prospective Authors
- Web Seminars
- Exhibits & Sponsorship
- Conference Reviewers
- National Conference • Denver 24
- Leaders Institute 2024
- National Conference • New Orleans 24
- Submit a Proposal
- Latest Resources
- Professional Learning Units & Courses
- For Districts
- Online Course Providers
- Schools & Districts
- College Professors & Students
- The Standards
- Teachers and Admin
- eCYBERMISSION
- Toshiba/NSTA ExploraVision
- Junior Science & Humanities Symposium
- Teaching Awards
- Climate Change
- Earth & Space Science
- New Science Teachers
- Early Childhood
- Middle School
- High School
- Postsecondary
- Informal Education
- Journal Articles
- Lesson Plans
- e-newsletters
- Science & Children
- Science Scope
- The Science Teacher
- Journal of College Sci. Teaching
- Connected Science Learning
- NSTA Reports
- Next-Gen Navigator
- Science Update
- Teacher Tip Tuesday
- Trans. Sci. Learning
MyNSTA Community
- My Collections
Nuclear Meltdown in Fukushima
Human Accident or Natural Disaster
By Beth A. Carle
Share Start a Discussion
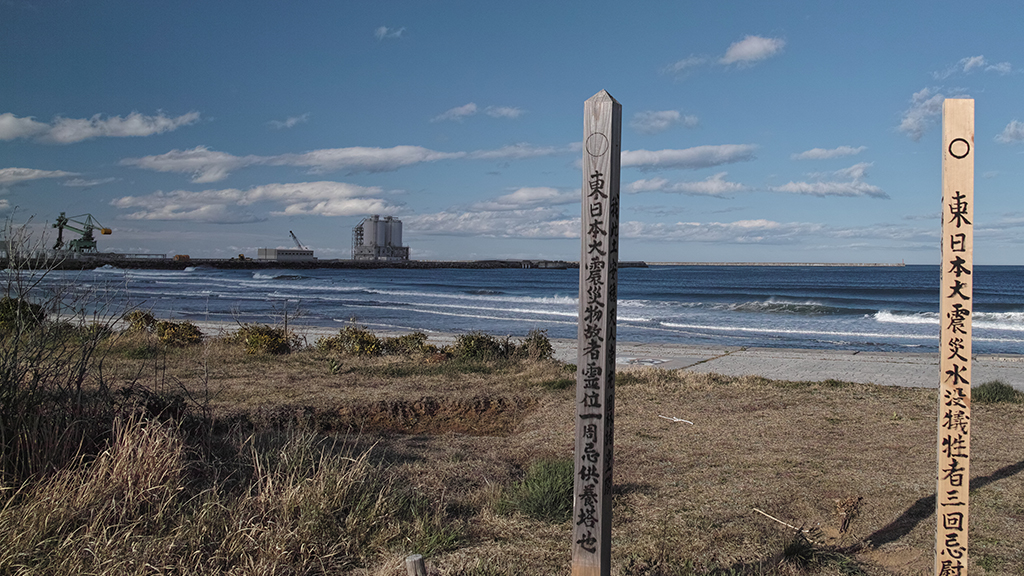
This decision case study is based on the nuclear accident in Fukushima, Japan that began on March 11, 2011 and examines its status through September 2013. The seven parts of the case were originally developed for delivery over two 75-minute class periods. Day 1 focuses on the technical background of the accident, the effects on the local population, and cleanup efforts. Day 2 focuses on the human actions that contributed to the accident and its progression, the plans intended to prevent an accident, and the responses of the government and electric company. Day 2 wraps up with a discussion of the overall question of "Was the Fukushima disaster primarily a natural disaster exacerbated by human actions, or was it primarily a human accident precipitated by a natural disaster?" This case was developed as a starting point for an introduction to nuclear energy course at the junior or senior level in engineering technology or engineering. It could also be used at a lower level and in other energy-related courses. It presumes little-to-no nuclear energy background. Technical background on nuclear reactors and radiation is provided in the teaching notes.
Download Case
Date Posted
- Describe the role of nuclear power world-wide.
- Outline the accident at Fukushima.
- Discuss the human toll of the accident.
- Describe the cleanup efforts.
- Discuss how nuclear power plants are designed to avoid accidents.
- Discuss emergency planning and response.
- Discuss how the management of the accident has affected the residents of the surrounding areas.
Fukushima; nuclear energy; disaster; regulatory bodies; radiation; tsunami; evacuation; Chernobyl
Subject Headings
EDUCATIONAL LEVEL
High school, Undergraduate lower division
TOPICAL AREAS
Ethics, Regulatory issues, Science and the media
TYPE/METHODS
Teaching Notes & Answer Key
Teaching notes.
Case teaching notes are protected and access to them is limited to paid subscribed instructors. To become a paid subscriber, purchase a subscription here .
Teaching notes are intended to help teachers select and adopt a case. They typically include a summary of the case, teaching objectives, information about the intended audience, details about how the case may be taught, and a list of references and resources.
Download Notes
Answer Keys are protected and access to them is limited to paid subscribed instructors. To become a paid subscriber, purchase a subscription here .
Download Answer Key
Materials & Media
Supplemental materials, you may also like.
Web Seminar
Join us on Thursday, June 13, 2024, from 7:00 PM to 8:00 PM ET, to learn about the science and technology of firefighting. Wildfires have become an e...
Join us on Thursday, October 10, 2024, from 7:00 to 8:00 PM ET, for a Science Update web seminar presented by NOAA about climate science and marine sa...
Secondary Pre-service Teachers! Join us on Monday, October 21, 2024, from 7:00 to 8:15 PM ET to learn about safety considerations for the science labo...
A business journal from the Wharton School of the University of Pennsylvania
Lessons in Leadership from the Fukushima Nuclear Disaster
October 3, 2013 • 18 min read.
What can we learn from the Fukushima disaster in Japan? Part of a special report.
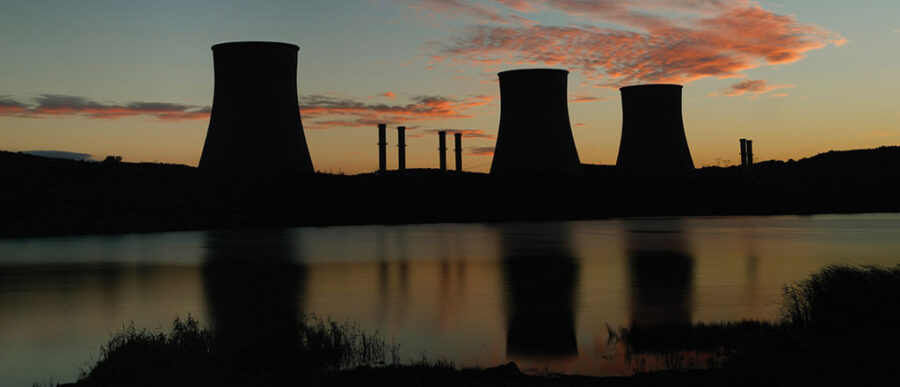
- Public Policy
Three widely cited investigations of the Fukushima disaster — one by the Japanese government, one by an independent team of experts in Japan and a third by The Carnegie Endowment for International Peace — have now concluded that the nuclear disaster of March 2011 was not, as it first seemed, the inevitable result of events no one could have predicted.
“It was a profoundly man-made disaster that could and should have been foreseen and prevented, ” said Kiyoshi Kurokawa, chairman of the Fukushima Nuclear Accident Independent Investigation Commission, established by the National Diet of Japan.
In an effort to understand what went wrong and what lessons in leadership the tragedy can offer, leaders directly and indirectly involved in the disaster spoke candidly at the Tokyo panel on Fukushima sponsored by Wharton’s Initiative for Global Environmental Leadership (IGEL). Based on their presentations in Tokyo and the analyses of others in Japan and elsewhere, three areas emerge as essential to leadership in a crisis: preparation for emergencies, leadership style and communications.
Lesson 1: To prepare for the worst, leaders have to face up to what might actually occur.
Erwann Michel-Kerjan , managing director of the Risk Management and Decision Processes Center at Wharton, says that Japan spent the years following the 1995 Kobe earthquake, the nation’s last great crisis, diligently preparing for future disasters. Since many people died in train wrecks during the Kobe quake, the Japanese government re-engineered its entire railway system. As a result, just moments after the earthquake in 2011, and well before the tsunami hit, Japan’s high-speed bullet trains were successfully shut down, saving countless lives (no one died on trains on 3/11, says Michel-Kerjan).
But just as generals are sometimes faulted for “fighting the last war,” Japan’s mistake was preparing for a disaster like Kobe, which was far less severe and complex than Fukushima. The 3/11 earthquake was significantly more intense (9.0 vs. 7.2 on the logarithmic Richter Scale) and it not only badly damaged the Fukushima Daiichi nuclear plant but also crippled the area’s power grid, cutting off the nuclear facility’s access to any off-site electricity.
This series of events — the destruction of the Fukushima Daiichi plant by a massive earthquake and tsunami — was entirely predictable.
Back-up generators kicked in when the power grid went down, but they were obliterated when the tsunami hit. This loss of virtually all power meant that the nuclear fuel inside damaged reactors went without essential cooling. In Reactor 1, the exposed fuel soon reached 2,800 degrees Centigrade. Desperate workers tried to cool the fuel with water from fire trucks and relieve building pressure inside the reactors by venting gases and steam. The venting, subsequent explosions and leaks led to the release of radioactive material into both the atmosphere and the ground water.
This series of events — the destruction of the Fukushima Daiichi plant by a massive earthquake and tsunami — was entirely predictable. In “Why Power Companies Build Nuclear Reactors on Fault Lines: The Case of Japan,” J. Mark Ramseyer, a visiting professor of Japanese legal studies at Harvard, notes that earthquakes of comparable magnitude have struck the northeast coast of Japan, on average, every 100 years, each one generating a devastating tsunami. In fact, the previous tsunami in 1933 was almost precisely as high as the one that struck Japan on 3/11.
Robert Meyer , co-director of the Risk Management and Decision Processes Center, points out that this pattern has been well known since ancient times. A monument from the first century still sits on a hill, high above the area destroyed by the 2011 tsunami, he says. Its inscription reads, “Beware the great tsunami; do not build below this level.”
Despite these historical warnings, “Nobody was remotely prepared,” says Akihisa Shiozaki, an attorney who was instrumental in putting together the first independent, non-governmental investigation of the Fukushima nuclear disaster, titled, “The Independent Investigation Report on the Fukushima Nuclear Crisis.” Not only were leaders unprepared for a tsunami following a major earthquake, they also failed to anticipate both the damage inflicted on the nuclear plant and the total loss of power to the cooling systems of the plant.
The failure to adequately prepare was widespread. The leaders of Tokyo Electric Power Company (TEPCO) built the reactors on a known fault line and then colluded with government regulators to avoid preparing for the inevitable. And the office of then-Prime Minister Naoto Kan (the Kantei) was totally unprepared to manage the crisis that resulted. As the report commissioned by the National Diet of Japan concluded, “The government, the regulators, TEPCO management, and the Kantei lacked the preparation and the mindset to efficiently operate an emergency response to an accident of this scope. None, therefore, were effective in preventing or limiting the consequential damage.”
Several reasons have been offered to explain why so many in leadership positions ignored the warnings of history. All are valid and offer valuable lessons to leaders in future crises.
Shiozaki looks to history itself to explain the reluctance of those in power to consider worst-case scenarios. After World War II and the destruction of Nagasaki and Hiroshima by nuclear weapons, the Japanese population vehemently opposed all use of nuclear power in their country. So the government undertook a campaign to persuade people of “the absolute safeness” of nuclear power, says Shiozaki. “Absolute safeness meaning that there was no risk that something could go wrong, no risk that a meltdown could happen. Well, that myth of absolute safeness developed over the years into a culture where it almost became a taboo to even talk about this…. Discussing a worst-case scenario was feared because it might bring panic to the citizens. And therefore it was omitted from the regulatory discussions.”
Eric Feldman , a law professor at the University of Pennsylvania, emphasizes the political and economic forces behind this reluctance to confront worst-case scenarios. “There was, of course, a good deal of local opposition [to nuclear power] despite the government’s downplaying of the risks, which is why it took so long for the first plants to be built,” he notes. With significant political and economic forces backing nuclear power, “talking about worst-case scenarios was avoided not simply because it would scare people, but because such fear would mean that local communities would oppose the building of reactors, and without local support the reactors would not be built.”
Ramseyer views the problem from a legal perspective, pointing to the “moral hazard” that arises when the potential losses of a catastrophe far exceed the value of a company. Like any private company, TEPCO’s liability was “capped by the value of its net assets.” Beyond that amount, the company would pay nothing, leaving them with “no incentive to limit damages beyond the value of those net assets. For risks beyond that point, they capture all the returns but bear none of the costs.” The result was that “Tokyo Electric wildly underplayed the risk of a large earthquake and tsunami, but it did not underplay it carelessly or negligently. It underplayed it rationally — wildly, but rationally.”
And then there is simple human nature – there is a “threshold of concern,” says Howard Kunreuther , Wharton professor of operations and information management and co-director of the Risk Management and Decision Processes Center. “You have a lot of things to worry about, and often when you talk about what the chances are of an accident like this occurring, the general feeling is that it’s not going to happen to me,” he said. “That’s not just true in Japan, it’s true around the world.”
Despite the historical inevitability of the earthquake and tsunami, says Kunreuther, the earlier events happened so long ago that “there was a tendency to ignore them. They come out of the woodwork after an event, when everyone looks back and says, ‘Oh, we should have known this.’ But it’s not easy for people to make these decisions when there are so many things on their agenda.”
One way to combat this natural tendency, suggests Kunreuther, is to “stretch time horizons, so you don’t just think about the likelihood of this occurring next year but over a period of years.” The probability of an event, or series of events, increases considerably “when you look at the situation over the next 20 years instead of over the next year.” The geologic time scale involved in the Fukushima disaster — and the decades of radioactive contamination left in its wake—make it seem prudent to extend the planning time horizon a good deal further in some cases.
The lesson is clear: To adequately prepare for worst-case situations, those in power need to look past cultural prejudices, shortsighted financial reasoning and their own limited experience. Whether the leaders of private companies worried about quarterly earnings or governments struggling to meet pressing needs can, or will take this lesson to heart, is an open question.
Lesson 2: In the midst of chaos, leaders should stop looking for control and start looking for answers.
Asked what experience he found most challenging during 3/11, Kenichi Shimomura, former deputy director general for public relations and chief spokesman for the Japan’s prime minister during the crisis, replied, “Being a leader without information.” It is easy, he said, to make decisions when a leader has all the necessary facts, “but information about the nuclear crisis was a luxury we did not have in the prime minister’s office at the time.”
The National Diet’s report explains that “as the situation deteriorated and the planned government accident response systems failed to function, control of the emergency response was taken by the Kantei, with Prime Minister Kan at the center of an ad hoc group of politicians, advisors and the chairman of the Nuclear and Industrial Safety Agency (NISA).”
While the government report states that this group did not include any experts, Shimomura told those attending the IGEL panel that there were in fact three top-ranked nuclear experts on hand. However, these experts were incapable of contributing to the decision-making, because of what Shimomura called not just a blackout of energy, but a “blackout of experts,” who seemed incapable of providing any useful guidance.
“With each new report from the site, the prime minister would ask [the experts] what he should do … and each time they averted their eyes…. Once the prime minister asked one of the scientific experts a question directly, but the expert was at a complete loss for words.” Even when asked to consult with his company, this expert was frozen in place until Shimomura literally walked up to him, and whispered into his ear that he should take out his cell phone and call his company for answers in the moment. “I was shocked,” said Shimomura. “He had lost his ability to make any decisions on his own.”
The problem was not just that Prime Minister Kan made these mistakes, but that no one in his inner circle questioned his decisions, offered other options or acted independently of the Kantei.
The prime minister also contributed to the confusion. Instead of helping to orchestrate the vast multitude of problems contributing to the disaster, Prime Minister Kan immersed himself in the minutiae of the nuclear plant. Shiozaki reports that Kan and his closest advisors brought a white board into his office and started “counting the number of trucks that were trying to arrive at the Fukushima nuclear plant, trying to check what types of electricity codes could be connected to the power plants.” In a vain attempt to gain direct knowledge of what was going on, Kan himself visited the Fukushima Daiichi plant, which “disrupted the chain of command and brought disorder to an already dire situation at the site,” according to the National Diet’s report.
The prime minister also refused to delegate decision-making. Kan “allowed the reporting line to stretch out in a very multi-layered hierarchy up to the final decision maker, which was him,” impeding what was already poor communications with TEPCO, noted Shiozaki. It was not until “five days after the crisis that he delegated the decision-making power to a lower-level joint team,” which included both his advisors and TEPCO management.
The problem was not just that Prime Minister Kan made these mistakes, but that no one in his inner circle questioned his decisions, offered other options or acted independently of the Kantei. In his introduction to the National Diet’s report, Kurokawa attributes this failing to Japanese culture. “What must be admitted, very painfully, is that this was a disaster ‘Made in Japan,’ ” Kurokawa writes. “ Its fundamental causes are to be found in the ingrained conventions of Japanese culture: Our reflexive obedience; our reluctance to question authority; our devotion to ‘sticking with the program’; our group-ism; and our insularity.” It was this “mindset of obedience to authority” that hindered the free flow of ideas and information, according to the parliamentary report.
Japanese culture and authoritarian leadership, which have endured for centuries, clearly offer important advantages. But those benefits are much more evident when information and time are plentiful. “Hierarchical culture works well when you have lots of time to make a decision; it helps you make a good decision,” notes Michel-Kerjan. “But by definition, crisis management is about making very quick decisions with very limited information.”
Feldman believes that “stereotypes such as ‘reflexive obedience’ and ‘reluctance to question authority’ are not terribly illuminating, and seem an odd juxtaposition with the public anti-nuclear demonstrations post-Fukushima.” He agrees with those who suggest that Prime Minister Kan’s personal leadership style is just as likely as Japanese culture to have discouraged dissent.
It is never easy to distinguish the personal from the cultural. Was Kan’s failure to delegate the result of a personality trait or of Japanese culture? Did the nuclear experts in his office and at the site fail to speak up because of a cultural mindset or because, as some of them later told interviewers, they were intimidated by Kan, who berated them publicly?
Whatever the mix of culture and personal qualities were in the Fukushima case, the lesson for leaders struggling to manage a crisis is as simple to state as it is difficult to implement: Rise above the fog of details; encourage fresh thinking and frank communication; and delegate decision-making to those best able to make decisions on the ground.
Lessson 3: In a crisis, leaders have to prevent panic and maintain credibility. Rehearsals help.
At each stage of the crisis, experts offered Kan varying estimates of the area that should be evacuated, and each time, Kan took the safest option. But the estimates kept changing, so Kan kept changing what he told the public. Paradoxically, this emphasis on safety and truthfulness led to growing fear and mistrust.
“When the safest option was 10 kilometers, the prime minister went with 10 kilometers. When it was 20 kilometers, he chose 20 kilometers,” Shimomura said. “But each time the radius of the evacuation zone increased, so did the people’s mistrust of the government. People accused the government of underestimating and playing down the gravity of the situation. It was far from the case. The public thought we were lying or hiding something.” Yet, “we didn’t have the luxury of any certain information to hide.”
“Try to share information about the whole iceberg, not only the tip of the iceberg.” –Kenichi Shimomura
“What would you do in a situation like that?” asked Shiozaki. “If you said on the first day, ‘Twenty kilometers should evacuate,’ you’re likely to cause a panic. Roads would be filled. Elderly people would be left behind in their homes. People in hospitals might lose their lives because of the confusion.” And in fact, when Kan first announced an evacuation zone of just three kilometers, he had been told that was the safest distance.
Shimomura, who was in charge of communication with the public, noted: “If your leader is a liar, you can solve the problem by kicking out your leader. But if the leader is honest, but still causes these problems, what should you do? This was my most challenging leadership experience.”
Shimomura told those at the IGEL panel that “for two years, I have asked myself the question, was there a better way to handle the crisis communication?” His answer: “Try to share information about the whole iceberg, not only the tip of the iceberg.”
In particular, Shimomura believes that while Kan was personally announcing the official evacuation zone, others in his office — but not the prime minister himself — should have provided the public with the information leading up to the decision, including other possibilities or scenarios that were considered during various meetings. This would allow Kan to maintain his position of authority but also make it clear that his announcement was not likely to be the last word, that what was being released was provisional. “The PM could have shown the tip and his office could have shown the rest of the iceberg.”
A more direct version of this approach, reminiscent of the way Mayor Rudolph Giuliani communicated with the public during the 9/11 crisis in New York City, has proven effective in other crises. The prime minister, suggests Michel-Kerjan, could have told people that he would report to them on a regular basis, say twice a day, and share what he knew at that point. This approach would allow him to maintain a reassuring position of authority, but also admit the truth about what he did and did not know. And by telling people that ‘we are all in this together, all struggling to get the right information,’ he could also help build a sense of trust and community.
What many people don’t realize about Giuliani’s mastery of this approach was that he had rehearsed it numerous times. Michel-Kerjan points out that New York City had large-scale crisis management rehearsals on a quarterly basis before 9/11, and Giuliani participated in every one.
This last point is key. When Michel-Kerjan works with corporations on similar rehearsals, he always insists that the CEOs participate, because they are the ones who will be on the front lines. “When you look at companies that have handled crises well,” says Michel-Kerjan, “every one of them has had conventional and unconventional rehearsal exercises quite a few times, typically with the CEO present.”
The performance of the JX Nippon Oil and Energy Corporation offers compelling evidence of how useful rehearsals can be. For the past 20 years, ever since the Kobe earthquake, the company has conducted annual disaster drills that included the formation and training of teams charged with specific duties: employee safety, the gathering of information from within the company and from outside sources, identifying and securing emergency supplies, information technology and engineering and construction.
Hiroshi Hosoi, executive officer and senior vice president of the company, told the IGEL attendees that this preparation helped explain how JX Nippon was able to overcome the devastation of its Sendai refinery and the loss of all its trucks and railroad tankers, and find ways to deliver badly needed fuel for cars, home heating and emergency vehicles.
The company also worked with competitors and the government to “enable fuel supplies to flow smoothly to where they were most needed, like evacuation centers, hospitals, power plants, etc.,” said Hosoi. And throughout the crisis, JX Nippon communicated on a regular basis with the public, updating which service stations were open and discussing the supply situation on their website.
While Hosoi pointed out that “March 11 was different,” it is clear that the yearly disaster drills prepared the company to rise to the same challenges all leaders confront in a crisis — facing up to a worst-case situation, quickly gathering information, encouraging fresh thinking, delegating decision-making and communicating openly and regularly with a community desperate for answers.
More From Knowledge at Wharton
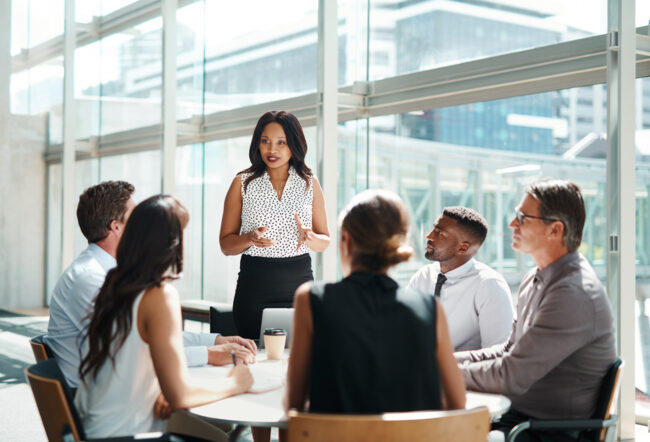
Three Things All New Managers Should Be Doing
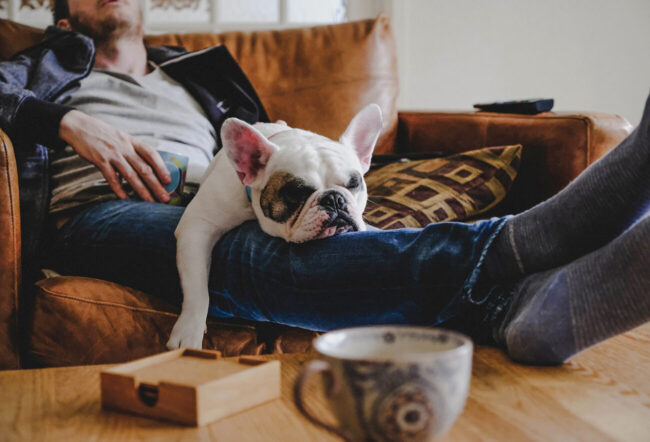
Working for the Weekend: Downtime and Performance
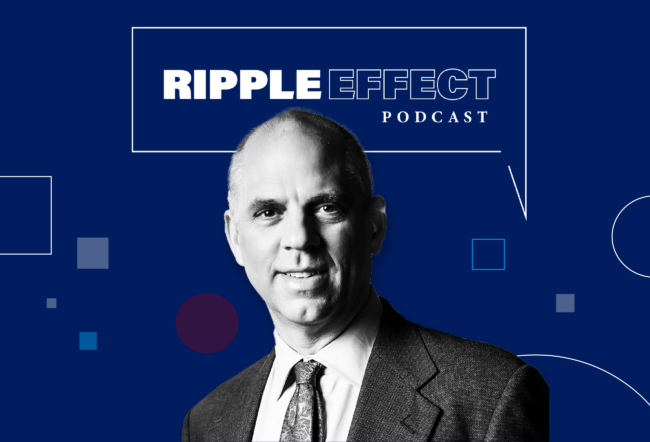
How Financial Literacy Helps Underserved Students | David Musto
Looking for more insights.
Sign up to stay informed about our latest article releases.
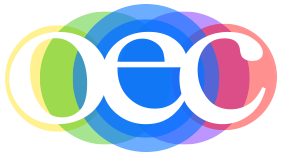
Site Search
- How to Search
- Advisory Group
- Editorial Board
- OEC Fellows
- History and Funding
- Using OEC Materials
- Collections
- Research Ethics Resources
- Ethics Projects
- Communities of Practice
- Get Involved
- Submit Content
- Open Access Membership
- Become a Partner
Three Mile Island Nuclear Accident
Detailed description of accident.
At 36 seconds past 4:00 a.m. on the 28th of March, 1979 the first of a series of pumps feeding water to the steam generators at the Three Mile Island nuclear plant stopped functioning.
It was later determined that this was caused by maintenance work being done on the Number 7 Polisher at the time. The steam produced by the reactor not only runs the generators to produce electricity but also serves to cool down the reactor core, reducing the risk of a meltdown. When the flow of the water stopped the temperature inside the reactor core increased. This caused the water inside the reactor to expand, increasing the pressure inside the pressurizer to 2200 psi, 100 psi more then the normal. As designed the increase in pressure caused the Pilot Operated Relief Valve to open (PORV) draining the steam and water from the reactor core in to a tank on the floor.
At this point the three emergency feedwater pumps started. An operator noticed that they were running, but he did not notice that the valves on the emergency feedwater lines were closed. The panel in the control room had lights to indicate that the valves were closed, one of the lights was covered by a yellow maintenance tag, while the operator did not notice the other light.
Despite the opening of the PORV the pressure continued to rise, 9 seconds into the accident the control rods automatically lowered as designed to halt the fission reaction. This halted the fission reaction but the latent heat of the radioactive material continued to heat the water. Even though this heat was a fraction of what is normally produced during the fission reaction, it was enough to potentially overheat the core. 13 seconds into the accident the PORV should have closed since the pressure in the reactor had dropped. The light indicating that the PORV was energized (i.e. open) shut off, leading the operators to assume that the PORV had was now closed. In reality the PORV was stuck open and steam and water was escaping from the reactor. This is known as a Loss of Coolant Accident (LOCA).
In the first 100 minutes of the accident almost 32,000 gallons of water, or one third of the reactors capacity escaped through the PORV. The accident at TMI could have been contained had either the PORV shut at this point, or the operators noticed that the valve was stuck open. During the initial stages of the accident Edward Fredrick and Craig Faust, the Control Room Operators were present in the control room. One of the factors which added to the severity of the accident at TMI was the inadequate training of the employees at the facility. The training was the responsibility of Met Ed and Babcock and Wilcox.
Over 100 alarms went off in the control room during the first few minutes of the accident. This added to the confusion without providing any useful information to the operators. Both Faust and Fredrick dealt with the alarms to the best of their ability based on the training they had received.
2 Minutes into the accident the pressure if the water in the reactor dropped sharply which caused the Emergency Injection Water (EIW) to be automatically activated. This sent about 1000 gallons of water per minute into the reactor coolant system.
The falling pressure coupled with constant reactor coolant temperature should have alerted the operators to the LOCA. Instead, fearing that the core would have too much water, Operator Fredrick turned off one of the EIW pumps, reducing the flow of water into the core to less then 100 gallons per minute.
8 Minutes into the accident it was discovered that the emergency feed water was not reaching the core because of the closed valves. Operator Faust proceeded to open these valves, allowing water to rush into the steam generators. It was later discovered that these valves were closed two days earlier during a routine test of the pumps. At 4:11 a.m. an alarm signaled high water in the containment building. This is the building where all the water draining from the core through the PORV is stored. In itself this alarm should have been a clear indication of a leak in the coolant system. When Fredrick was informed of this he recommended that the sump pumps draining water to the containment building be shut off.
By about 6:15 a.m., roughly 2 hours and 15 minutes into the accident, the level of water in the reactor had dropped below the level of the core. Unknown to the operators the core is now uncovered. It is essential that the core remain covered at all times to prevent it from over heating. Once the core over heats the zirconium alloy of the fuel rod cladding reacts with the steam to produce hydrogen gas. This is what happened in the TMI reactor, not only is the hydrogen produced flammable, but the diminishment of the fuel rod cladding increases the risk of radioactive contamination.
The water continued to leak out of the PORV until 6:22 a.m. when Leland Rogers, the Babcock & Wilcox site representative at TMI asked the operators if they had shut the block valve which is the backup valve to the PORV. At this point the PORV was shut after having remained open for 2 hours and 22 minutes. It is still unclear whether Rogers was responsible for the valve being shut. Edward Fredrick testified that the valve was closed at the suggestion of a shift supervisor coming onto the next shift. Based on increasing radiation levels, at around 7:00 a.m. a site emergency was declared, indicating the threat of release of radioactivity into the surrounding environment. As the radiation levels continued to increase, the staff of TMI-2 was contacting local law enforcement and local energy providers. At 7:24 a general emergency was declared at the TMI-2 site and the site began to be evacuated. By 11:00 a.m. all nonessential personnel were ordered off of the island, and the remaining technicians were forced to wear face masks to block any air bourne radioactive particles. At 1:50 p.m. the operators in the control room heard what they described as a loud "thud." While they dismissed the sound as well as the pressure spike indicated by their instruments as a malfunction, the thud was actually a hydrogen explosion inside the containment building. It is another several hours before the partially melted core is brought to a controllable temperature using water from the primary loops.
Immediate Reaction to the Accident
Shortly after the accident occurred, an incorrect reading reported traces of radioactive iodine-131 in the Goldsboror area. While the reading caused an immediate need for concern, the readings were later found to be erroneous. A build up in radioactive iodine in human's thyroids from inhaling radioactive particles or drinking contaminated milk remained the primary concern of the top officials including Governor William Scranton III. The Governor was incorrectly informed that the release of steam represented the primary concern to the surrounding environment, despite the fact that there was no indication that radioactive steam had escaped from the reactor core or the pumps feeding the core.
The evening of the accident, mayor of Goldsboro, Ken Meyers, met with his council members to discuss the possibility of an evacuation. The council members proceeded to go door to door through the town providing families with whatever information they could, mostly consisting of information they themselves had heard from news reports, as well as explaining the evacuation procedure should Governor Scranton declare a state of emergency.
Following the accident, numerous tests were conducted in attempts to conclude what if any effect increased radiation would have on the health of human, animal and plant life in the surrounding area. Through these studies, it was revealed that the majority of radioactive material had been contained by the core. Despite the immediate concern, however, a later government report indicated that there would likely be one additional death as a result of cancer due to increased radiation levels in the surrounding area in a 30 year period.
Back to Top
The Cleanup Operation
During the cleanup operations at the Three Mile Island nuclear reactor there were issues of ethical misconduct on the part of both organizations and individuals that occurred. Professor Stephen Unger of Columbia University has discussed one such example in his book "Controlling Technology."
Background Information
In order to further discuss these issues it is important to understand the relationship between the organizations and individuals involved.
The key individuals involved were:
The task for cleaning up was given to an organization involving both General Public Utilities ( GPU) and the Bechtel Northern Corporation. At the same time the Nuclear Regulatory Commission (NRC) was present on site to monitor the cleanup operations.
Laurence P. King was responsible for the site cleanup operations on the GPU side.
Richard D. Parks was employed by Bechtel as a senior startup engineer and seconded as an operations engineer to the Site Operations Department under Laurence P. King. His duties involved liaison with the NRC and ensuring compliance with licensing requirements. In addition he served as an alternate startup and test manager for the plant.
Edwin H. Gischel was the Plant Engineering Director and worked under Laurence P. King.
One of the issues later brought up was that the NRC worked too closely with the organizations directly involved with the clean up of the reactor. This tended to take away from the NRC's ability to regulate the safety and effectiveness of the operations. There were times when proposals would be unofficially approved by the NRC before the other organizations were able to fully develop or approve them. This, combined with the perception that the NRC was more concerned with expediting the cleanup process rather than ensuring it was done correctly encouraged people to cut corners.
In any industrial operation overhead cranes used for lifting heavy equipment play an important role. The polar crane used during the cleanup of TMI-2 had been damaged during the original accident. During the repairing of the crane various parts that were damaged had been substituted with replacements that were not exactly the same as the originals. In addition the crane had been modified to perform duties that exceeded the original manufacturer specifications. Due to the nature of the cleanup operation the changes made posed a hazard to not only the workers on site but also to people in the surrounding areas.
King received reports from both Parks and Gischel which stated concerns over the use of the Polar Crane. Prior to that King had already experienced difficulties with the cleanup operations. He felt that established engineering procedures were not being followed by other high level managers, particularly Bechtel people.
Based on what he had seen and the reports he received King decided that the crane should be carefully tested before being put into service. Top management at GPUN and Bechtel did not agree with King's assessment of the situation and felt that he was being overly cautious.
In reaction to the Gischel's report, King's superior ordered King to immediately fire Gischel. King refused and defended Gischel's position. At this point GPU's tactics turned nasty.
After suffering a stroke a year before, Gischel had seen a psychologist working under GPU. At the time, the psychologist suggested Gischel take a neuropsychological evaluation, but Gischel refused to take the test. Almost immediately following the release of the report, he again received a letter suggesting he take the exam. Robert Arnold, the president of GPU Nuclear, continued pressuring Gischel and in a clear violation of the doctor-patient relationship disclosed that he had knowledge of the topics of Gischel's meetings with the GPU employed psychologist. After a letter insisting Gischel take the exam from the board chairman of GPU, Gischel sent a sworn affidavit to Arnold explaining his take on the TMI cleanup operations and the events that followed. Gischel requested that Arnold forward the affidavit to the NRC which he did, but on July 1 after Gischel still refused to take the neuropsychological exam he was transferred to a different subsidiary of GPU.
Richard Parks also filed a sworn affidavit and submitted it to the NRC, and a harassment complaint filed against GPU Nuclear. Parks's complaint was filed on the grounds that a manager told him Parks's superiors were considering transferring him off site, he was demoted from his position as alternate startup and test manager, and was informed of a false rumor that his ex-wife (who was actually deceased) was trying to dig up dirt on Parks to get custody of their two children. The day after he filed his complaint Parks was suspended with pay on the grounds that his continued working would subject him to continued harassment from his coworkers.
In February of 1983 GPU Nuclear accused King of hiring employees away from GPUN for his own firm, Quiltec. Accused of a conflict of interest, King was suspended and locked out of his office while he claims that documents he wrote concerning the cleanup process were destroyed. King's secretary, Joyce Wagner, was also forced into suspension and within a month both king and Wagner had been fired.
The Accident's Impact
The numerous ethical questions surrounding the TMI-2 cleanup procedure might suggest that nothing positive could have resulted from the incident. Beyond the questionable behavior on the part of the companies responsible for the cleanup, radiation presented a major problem for the cleanup crews as did algae that not only grew, but thrived in the contaminated water. There were important scientific as well as sociological issues that resulted from the accident. The following is based on Matthew L. Wald's article "After the Meltdown, Lessons from a Cleanup" that appeared in the April 24, 1990 edition of the New York Times
What the cleanup effort did prove was that, despite requiring 400 workers, over 4 1/2 years, and 970 million dollars, the cleanup of a nuclear disaster area is possible. Unlike the Soviet approach to the cleanup at the Chernobyl disaster site, where the reactor was encased in an enormous concrete structure, the cleanup of the reactor site and especially the reactor vessel at TMI-2 provided many answers to questions surrounding the accident and even provided some hints as to the nature of exactly how stable reactor vessels are.
During the cleanup process the team learned that despite the 51% meltdown of the reactor core, that the reactor vessel was relatively undamaged. This indicated two separate points. First that it was far easier to have a meltdown to the core than expected, but secondly that at least in this case, the reactor vessel was able to withstand temperature much higher than anticipated.
Beyond scientific impact, however, remains the impact that the accident had on the public. Many analysts in the nuclear industry believe that the incident at TMI-2 has meant the nuclear industry has only one more chance. Analysts feel that another nuclear accident on the scale of TMI-2 will result in public outcry for the closing of the other nuclear plants around the nation.
Timeline of Events Occurring During the Three Mile Island Disaster
Nuclear reactor technical information.
It is important to understand the basics of how a nuclear reactor produces electricity and what the basic components of the reactor at Three Mile Island were. Three Mile Island was the home to two nuclear plants, TMI-1 and TMI-2. Their combined power generating capacity was 1,700 megawatts or enough electricity to supply 300,000 homes. In a nutshell, nuclear power plants generate electricity by using steam turbines. The function of the nuclear fuel is to heat water and convert it to steam. Now in theory this sounds quite simple, but the hazards associated with nuclear fuels add to the complications. A nuclear reactor such as TMI-2 can be divided into various sub-systems.
In order to understand the accident at TMI it is essential that we have a rudimentary understanding of how these systems function.
THE NUCLEAR REACTOR
A nuclear reactor generates heat by harnessing atomic energy. The core of an atom, which is also called the nucleus, can be split by bombarding it with neutrons. When a free neutron strikes the core it splits the atom into two smaller atom fragments, giving off energy in the form of kinetic energy of the fragments, plus 2 or three neutrons -and likely some gamma rays-. One of the neutrons produced by this reaction strikes another atom, which in turn produces more energy and more neutrons. This chain reaction continues from one atom to another producing nuclear fission energy (1) .
The TMI-2 Reactor Core held 100 tons of uranium to fuel the nuclear reaction. This was in the form of cylindrical uranium oxide pellets that were one inch tall and roughly one half inch wide. These pellets were stacked inside 12-foot long fuel rods made of a zirconium alloy (Zircaloy-4). The entire reactor consisted of 36,816 such fuel rods along with 69 control rods, 52 instrument tubes and spaces between them for the cooling water to flow.
Control rods are used to control the amount of power produced by the reactor, or to completely halt the reaction in the case of an emergency. The control rods are able to control the rate of nuclear fission taking place by absorbing a portion of the free neutrons produced. The rate varies depending on the number of rods and the length of the rod inserted into the core of the reactor. TMI-2 used control rods made of 80% Silver, 15% indium and 5% cadmium.
Control rods are designed such that in the event of an emergency the magnetic clamps holding them release the rods into the core of the reactor thus completely halting the reaction.
The Instrument Tubes contain instruments used to measure such things as the temperature within the reactor.
WATER SYSTEMS
There are three basic closed loop Water Systems in a nuclear power plant such as the one at TMI.
The first water system is the Primary Loop or the Reactor Coolant System. This is a closed loop system that circulates water through the nuclear core and serves two purposes. Firstly, by coming in contact with the hot fuel rods the water is heated. At the same time the water flowing through the core cools down the fuel rods preventing them from melting. It is important that the water coming in contact with the reactor core remain in liquid form so that it can effectively cool the rods. In order to prevent the water from turning into steam it is pressurized to about 2,155 psi. In the event of an accident either an increase in temperature or a decrease in pressure can convert the heated water to steam, this is undesirable but not dangerous because the steam too can help cool the reactor core.
Once the steam from the Feedwater System has driven the turbines it goes to the condenser where it is cooled and converted back to water. The final water system is the Condenser Water that is cooled in the cooling towers. This is the water used to cool the steam from the turbines and is also a closed loop water system. This ensures that the radioactive water from the reactor cannot contaminate the water in the cooling towers or the water vapor that is released from the cooling towers to the atmosphere.
Under normal operating conditions the primary loop is the only system which contains traces of radiation.
STEAM GENERATORS
Heated water from the reactor goes to the Steam Generator where it is passed through corrosion resistant tubes. At the same time water from the Secondary Loop or Feedwater System is passed through the steam generator around the tubes containing hot water from the reactor. This converts the Secondary Loop water into steam and also cools down the reactor coolant water. It is important to note that at no point does water from the two separate systems mix with each other.
STEAM TURBINE
The steam is then sent to the Steam Turbines where it runs the electricity-producing generator while the relatively cooler water of the Reactor Coolant System is sent back into the reactor core to pick up more heat and repeat the whole cycle.
AUXILIARIES
The Pressurizer ensures that the reactor coolant water is kept at the correct pressure. If for some reason the water pressure increases above safe levels the Pilot Operated Relief Valve (PORV) is supposed to open, thus relieving excess pressure by releasing water/steam to the drain tank. While this solves the immediate problem and prevents the reactor vessel from getting damaged, it reduces the level of water in nuclear core.
A reduction in the reactor core water level would lead to the core being uncovered. This is undesirable because it would allow the core temperature to increase beyond safe levels. If the core temperature were to reach around 2,200 degrees Fahrenheit the water would begin to react with the cladding producing hydrogen. This could potentially explode. If the temperature were to reach 5,200 degrees Fahrenheit the uranium could potentially melt, releasing far more radioactive materials into the surroundings.
- (1) Thanks to Elaine Walker for providing feedback and some of the information contained in this paragraph.
Related Resources
Submit Content to the OEC Donate
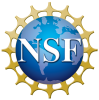
This material is based upon work supported by the National Science Foundation under Award No. 2055332. Any opinions, findings, and conclusions or recommendations expressed in this material are those of the author(s) and do not necessarily reflect the views of the National Science Foundation.
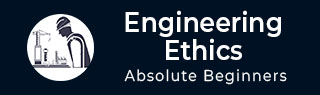
- Engineering Ethics Tutorial
- Engineering Ethics - Home
- Engineering Ethics - Introduction
- Engineering Ethics - Moral Issues
- Moral Dilemmas
- Moral Autonomy
- Kohlberg’s Theory
- Heinz’s Dilemma
- Engineering Ethics - Gilligan’s Theory
- Professions and Professionalism
- Engineering Ethics - Ethical Theories
- Social Experimentation
- Balanced Outlook on Law
- Responsibility for Safety
- Chernobyl’s Case Study
- Bhopal’s Gas Tragedy
- Responsibilities of Engineers
- Engineering Ethics - Confidentiality
- Rights of Engineers
- Engineering Ethics - Global Issues
- Moral Leadership
- Engineering Ethics Useful Resources
- Engineering Ethics - Quick Guide
- Engineering Ethics - Resources
- Engineering Ethics - Discussion
- Selected Reading
- UPSC IAS Exams Notes
- Developer's Best Practices
- Questions and Answers
- Effective Resume Writing
- HR Interview Questions
- Computer Glossary
Engineering Ethics - Chernobyl’s Case Study
The Chernobyl disaster was nuclear accident that occurred at Chernobyl Nuclear Power Plant on April 26, 1986. A nuclear meltdown in one of the reactors caused a fire that sent a plume of radioactive fallout that eventually spread all over Europe.
Chernobyl nuclear reactor plant, built at the banks of Pripyat river of Ukraine , had four reactors, each capable of producing 1,000 MWs of electric power.
On the evening of April 25 th 1986 , a group of engineers, planned an electrical engineering experiment on the Number 4 Reactor. With their little knowledge on Nuclear physics, they thought of experimenting how long turbines would spin and supply power to the main circulating pumps following a loss of main electrical power supply.
Following is an image of the Chernobyl nuclear power plant.
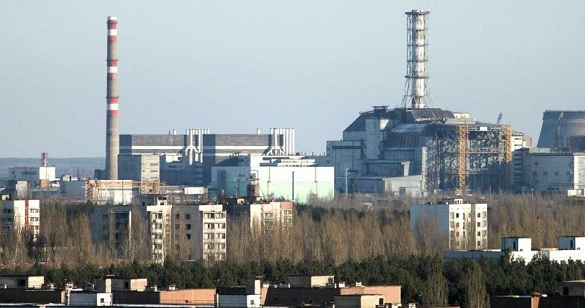
What Led to the Disaster?
Let us now see what led to the disaster.
The reactor unit 4 was to be shut down for routine maintenance on 25 April 1986. But, it was decided to take advantage of this shutdown to determine whether, in the event of a loss of station power, the slowing turbine could provide enough electrical power to operate the main core cooling water circulating pumps, until the diesel emergency power supply became operative. The aim of this test was to determine whether cooling of the core could continue in the event of a loss of power .
Due to the misconception that this experiment belongs to the non-nuclear part of the power plant, it was carried out without a proper exchange of information between the testing department and the safety department. Hence the test started with inadequate safety precautions and the operating personnel were not alerted to the nuclear safety implications of the electrical test and its potential danger.
The Experiment
According to the test planned, the Emergency Core Cooling System (ECCS) of the reactor, which provides water for cooling the reactor core, was shut down deliberately.
For the test to be conducted, the reactor has to be stabilized at about 700-1000 MW prior to shut down, but it fell down to 5000 MW due to some operational phenomenon. Later, the operator working in the night shift committed an error, by inserting the reactor control rods so far. This caused the reactor to go into a near-shutdown state, dropping the power output to around 30 MW.
Since this low power was not sufficient to make the test and will make the reactor unstable, it was decided to restore the power by extracting the control rods, which made the power stabilize at 200 MW. This was actually a violation to safety law, due to the positive void co-efficiency of the reactor. Positive void coefficient is the increasing number of reactivity in a reactor that changes into steam. The test was decided to be carried out at this power level.
Actually, the reactors were highly unstable at the low power level, primarily owing to the control rod design and the positive void coefficient factors that accelerated nuclear chain reaction and the power output if the reactors lost cooling water.
The following image shows the reactor 4 where the experiment was conducted. This picture was taken after everything was restored.
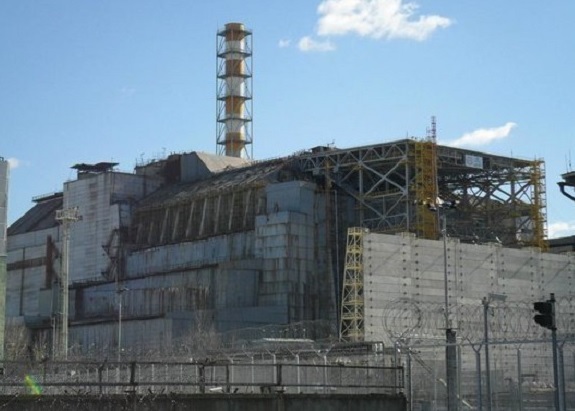
At 1:23, on April 26 th 1986, the engineers continued with their experiment and shut down the turbine engine to see if its inertial spinning would power the reactor’s water pumps. In fact, it did not adequately power the water pumps and without the cooling water the power level in the reactor got surged.
The water pumps started pumping water at a slower rate and they together with the entry to the core of slightly warmer feed water, may have caused boiling (void formation) at the bottom of the core. This, along with xenon burn out, might have increased the power level at the core. The power level was then increased to 530 MW and continued to rise. The fuel elements were ruptured and lead to steam generation, which increased the positive void coefficient resulting in high power output.
The high power output alarmed the engineers who tried to insert all the 200 control rods, which is a conventional procedure done in order to control the core temperature. But these rods got blocked half the way, because of their graphite tip design. So, before the control rods with their five-meter absorbent material, could penetrate the core, 200 graphite tips simultaneously entered the core which facilitated the reaction to increase, causing an explosion that blew off the 1,000-ton heavy steel and concrete lid of the reactor, consequently jamming the control rods, which were halfway down the reactor. As the channel pipes begin to rupture, mass steam generation occurred as a result of depressurization of the reactor cooling circuit.
As a result, two explosions were reported. The first one was the initial steam explosion. Eventually, after two to three seconds, a second explosion took place, which could be possibly from the build-up of hydrogen due to zirconium-steam reactions.
All the materials such as Fuel, Moderator and Structural materials were ejected, starting a number of fires and the destroyed core was exposed to the atmosphere. In the explosion and ensuing fire, more than 50 tons of radioactive material were released into the atmosphere, where it was carried by air currents. This was 400 times to the amount of radioactive materials released at the time of Hiroshima bombing.
Fatal Effects of the Disaster
The Chernobyl Nuclear Power Plant disaster in Ukraine, is the only accident in the history of commercial nuclear power to cause fatalities from radiation.
There were many fatal effects due to the radiation released. A few of the effects are listed below −
Two workers had died. One immediately got burnt to ashes after the accident, while the other was declared dead at the hospital within few hours of admission.
28 emergency workers and staff died within 4 months of the accident due to the thermal burns and the radiation effect on their bodies.
This accident created 7,000 cases of thyroid cancer.
Acute radiation syndrome (ARS) was diagnosed in 237 people, who were on-site and involved in cleaning up
The land, air and ground water were all contaminated to a great extent.
The direct and indirect exposure to radiation led to many severe health problems such as Downs Syndrome, Chromosomal Aberrations, Mutations, Leukemia, Thyroid Cancer and Congenital Malfunctions, etc.
A number of plants and animal faced destruction as after-effect.
Skip to content
Your browser does not support JavaScript, or it is disabled.Please check the site policy for more information.
National Report
- Korean Peninsula
- Around Asia
- Manga & Anime
The Asahi Shimbun
Deterrence could heighten risk of nuclear warfare, study suggests
By TAKASHI OGAWA/ Staff Writer
April 5, 2024 at 17:27 JST
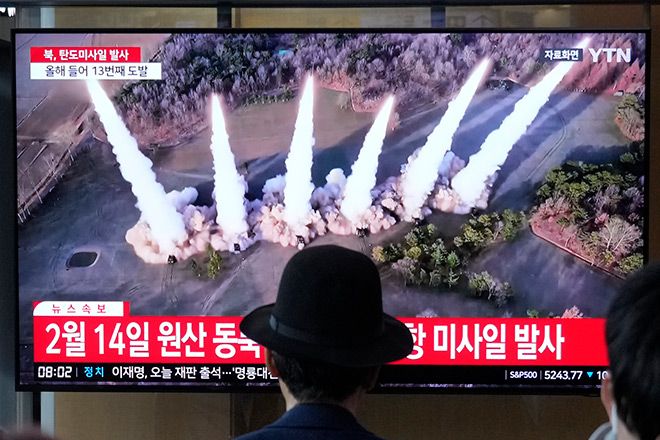
Rather than ensuring peace will prevail, the notion of nuclear deterrence could in certain situations in an Asian context heighten the risk of nuclear warfare erupting, and possibly going global, according to an international study.
This is the finding of a three-year simulation of various scenarios studied by experts at Nagasaki University’s Research Center for Nuclear Weapons Abolition (RECNA), the U.S. think tank Nautilus Institute for Security and Sustainability and the Asia-Pacific Leadership Network for Nuclear Non-Proliferation and Disarmament.
The results were announced at an April 3 news conference.
Noting that the objective of deterrence could backfire, the project group called on governments to take other measures to improve their national security environment.
Nuclear strategy experts from the United States, Russia, China and South Korea gathered to discuss 30 possible cases in which nuclear weapons might be used in Northeast Asia.
Research focused on five cases that involved different nations as well as various types of nuclear weapons.
Projections for fatalities were made for each of the five cases based on population density and architectural characteristics. The projections factored in things like the bomb blast, heat rays and radioactive fallout over the medium- and long-terms.
One case involved a first strike by North Korea followed by a response from the United States. The projection was for 11,000 fatalities.
The experts projected 220,000 fatalities if terrorists used a nuclear weapon in Japan.
A third case concerned a first strike by the United States followed by retaliatory action by North Korea using nuclear weapons on Japanese industrial facilities and China also becoming involved. This scenario envisaged 18 nuclear weapons being used, with fatalities in the range of 2.1 million.
The study found that a nuclear conflict based on regional issues, such as ones involving North Korea or Taiwan, could within a matter of hours or days escalate into a global nuclear war.
It concluded that efforts to strengthen and improve deterrence had conversely made it more difficult to obtain regional security.
Among the proposals to avoid a nuclear catastrophe were dialogue between the United States and China regarding the viability of their nuclear strategies as well as a new framework to further develop the Comprehensive Test Ban Treaty.
Related News
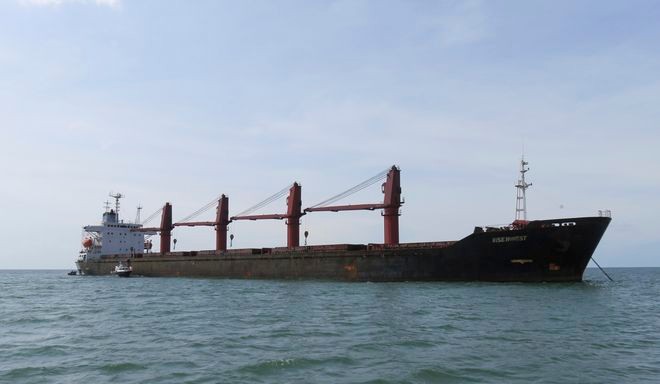
U.S. seizes North Korean ship amid tense moment in relations
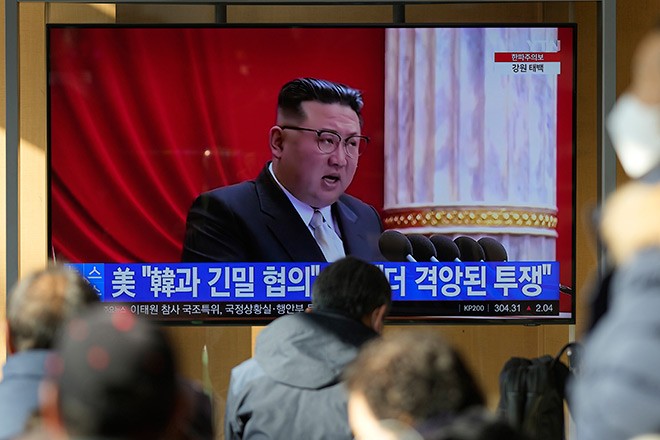
North Korea’s weapons programme defies COVID outbreak, reaches ‘uncharted territory’
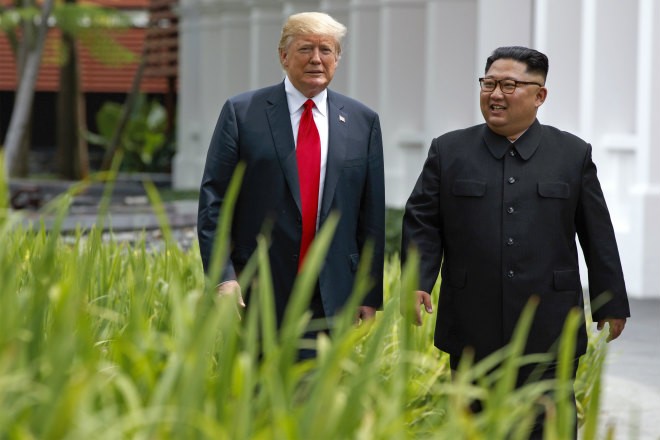
3 years later, Trump still lacks clear strategy to deal with China
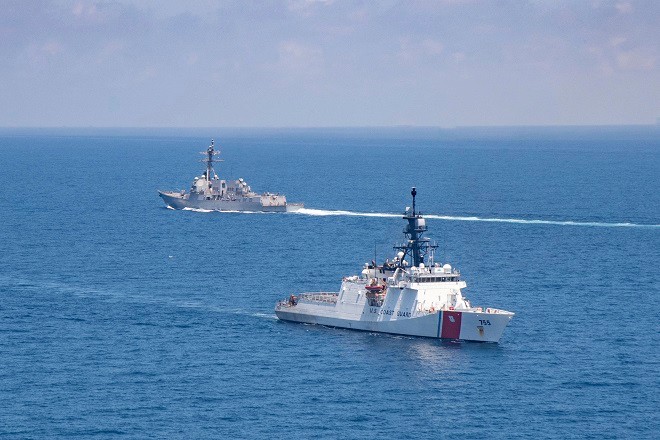
NKorea slams US for supporting Taiwan in nod to ally China
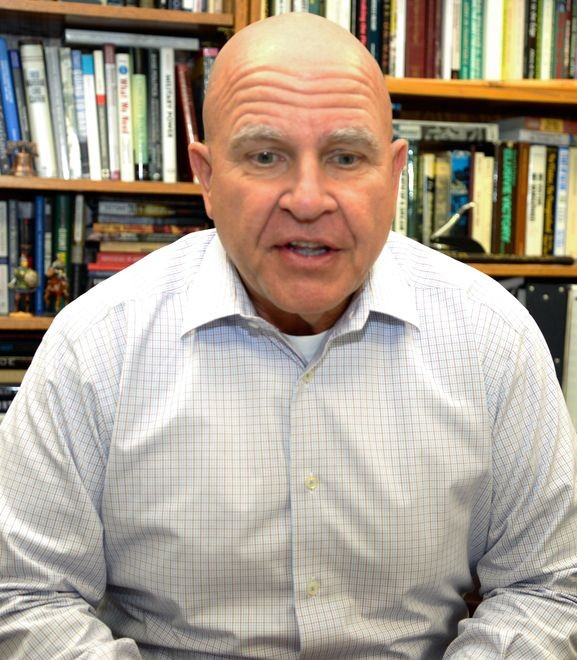
INTERVIEW: McMaster says tariffs on China should stay until behavior changes
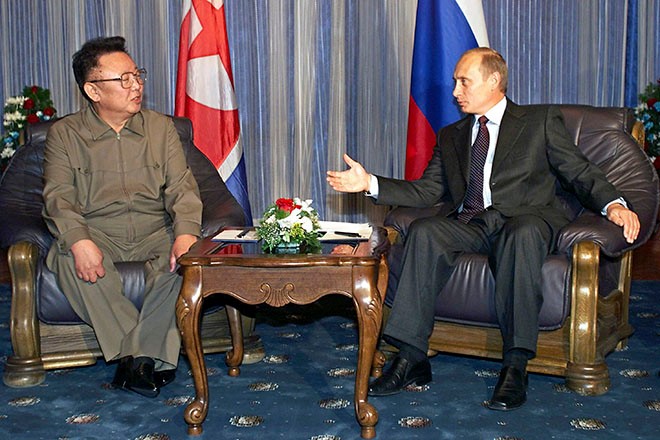
Kim visit adds twist to Russo-N. Korean relations since war
Trending Now
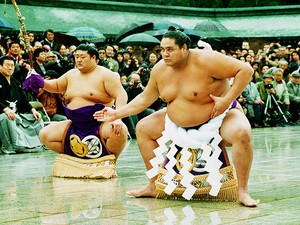
Slush avalanche cascades down Mt. Fuji during heavy rainstorm
April 12, 2024
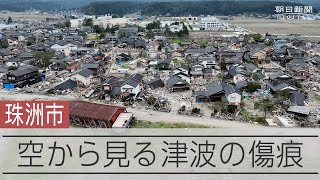
Collapsed houses left uncleared 100 days after Noto earthquake

Okinawa visitors witness Shuri-jo restoration work in progress
April 8, 2024
Recommended

Stories about memories of cherry blossoms solicited from readers

Cooking experts, chefs and others involved in the field of food introduce their special recipes intertwined with their paths in life.

A series based on diplomatic documents declassified by Japan’s Foreign Ministry

A series on the death of a Japanese woman that sparked a debate about criminal justice policy in the United States

A series about Japanese-Americans and their memories of World War II

Here is a collection of first-hand accounts by “hibakusha” atomic bomb survivors.
Learning English
- Asahi Weekly
In-house News and Messages
- The New York Times
BACK TO TOP
- Site Policy
- Transmission of user information to external service providers(利用者情報の外部送信)
Copyright © The Asahi Shimbun Company. All rights reserved. No reproduction or republication without written permission.

IMAGES
VIDEO
COMMENTS
Chernobyl disaster, accident at the Chernobyl nuclear power station in the Soviet Union in 1986, the worst disaster in nuclear power generation history. ... whether from years of experience gained by working on that content or via study for an advanced degree. They write new content and verify and edit content received from contributors.
The April 1986 disaster at the Chernobyl a nuclear power plant in Ukraine was the product of a flawed Soviet reactor design coupled with serious mistakes made by the plant operators b. It was a direct consequence of Cold War isolation and the resulting lack of any safety culture. The accident destroyed the Chernobyl 4 reactor, killing 30 ...
As the crisis deepened, Prime Minister Naoto Kan secretly instructed Shunsuke Kondo, chairman of the Japan Atomic Energy Commission (AEC), to draw up a worst-case scenario for the nuclear accident. This contingency scenario was submitted to the prime minister on March 25, 2011. 1 It projected that the crisis could deepen in the following manner:
This case study discusses the causes, consequences and implications of the nuclear disaster at Tokyo Electric Power Company's (TEPCO's) Fukushima Dai-ichi nuclear power plant that was triggered by a massive 9.0 magnitude earthquake and subsequent tsunami waves on March 11, 2011. There are two essential questions: First, "How could it have come so far?" Japan is rightfully considered a ...
The IAEA's Incident and Emergency Centre (IEC) received information from the International Seismic Safety Centre at approximately 08:15 Vienna Time concerning an earthquake with a magnitude of 9.0 near the east coast of Honshu, Japan's main island. This was followed by an accident at the Fukushima Daiichi Nuclear Power Station, which was ...
IAEA, Vienna, 2008 (ISSN 0074-1884; STI/PUB/1312; ISBN 978-92--110807-4) On 26 April 1986, the Number Four reactor at the Chornobyl Nuclear Power Plant in what then was the Soviet Union during improper testing at low-power, resulted in loss of control that led to an explosion and fire that demolished the reactor building and released ...
Following a major earthquake, a 15-metre tsunami disabled the power supply and cooling of three Fukushima Daiichi reactors, causing a nuclear accident beginning on 11 March 2011. All three cores largely melted in the first three days. The accident was rated level 7 on the International Nuclear and Radiological Event Scale, due to high ...
The Fukushima nuclear accident was a major nuclear accident ... (similar to unit 1) assumed the worst-case scenario and prepared for a LOC incident. However, when a team was sent to investigate the status of the RCIC of unit 2 the following morning (02:55), they confirmed that the RCIC was operating with the PCV pressure well below design ...
S.1. CAUSES OF THE FUKUSHIMA DAIICHI ACCIDENT (Study Charge 1) NAS's examination of the Fukushima Daiichi accident is provided in Chapters 3 and 4 of this report. Chapter 3 describes the March 11, 2011, Great East Japan Earthquake and tsunami and their impacts on Japanese nuclear plants. Chapter 4 describes the accident at the Fukushima Daiichi plant, including the accident time line, key ...
Current status and issues of Fukushima nuclear disaster areas and victims after lifting of evacuation orders: a case study of Namie Town Kota Kawasaki Faculty of Symbiotic Systems Science, Fukushima University, Fukushima, Japan ABSTRACT The Fukushima Daiichi Nuclear Power Plant Accident caused by the Great East Japan ...
Chernobyl is a nuclear power plant in Ukraine that was the site of the worst nuclear accident in history when a routine test went horribly wrong on April 26, 1986. ... A 2011 study by the U.S ...
The IAEA's accident reports detail the circumstances and consequences of nuclear and radiological accidents. They provide a detailed analysis of the accident's causes, an account of the accident response, lessons learned and, where applicable, the IAEA-facilitated international assistance. The case-specific reports aim to strengthen safety by making lessons learned from an
The Chernobyl disaster began on 26 April 1986 with the explosion of the No. 4 reactor of the Chernobyl Nuclear Power Plant, near the city of Pripyat in the north of the Ukrainian SSR, close to the border with the Byelorussian SSR, in the Soviet Union. It is one of only two nuclear energy accidents rated at seven—the maximum severity—on the International Nuclear Event Scale, the other being ...
Summary of the Fukushima Daiichi Disaster. The earthquake on March 11, 2011, off the east coast of Honshu, Japan's largest island, reportedly caused an automatic shutdown of 11 of Japan's 55 operating nuclear power plants.5 Most of the shutdowns proceeded without incident. However, the plants closest to the epicenter, Fukushima and Onagawa ...
Abstract. This decision case study is based on the nuclear accident in Fukushima, Japan that began on March 11, 2011 and examines its status through September 2013. The seven parts of the case were originally developed for delivery over two 75-minute class periods. Day 1 focuses on the technical background of the accident, the effects on the ...
Nuclear plant accidents The abandoned city of Pripyat, Ukraine, following the Chernobyl disaster.The Chernobyl nuclear power plant is in the background. The world's first nuclear reactor meltdown was the NRX reactor at Chalk River Laboratories, Ontario, Canada in 1952.. The worst nuclear accident to date is the Chernobyl disaster which occurred in 1986 in the Ukrainian SSR, now Ukraine.
In "Why Power Companies Build Nuclear Reactors on Fault Lines: The Case of Japan," J. Mark Ramseyer, a visiting professor of Japanese legal studies at Harvard, notes that earthquakes of ...
What makes the TMI-2 accident such an interesting case study is the series of events which led up to the partial meltdown of the reactor core. ... and 970 million dollars, the cleanup of a nuclear disaster area is possible. Unlike the Soviet approach to the cleanup at the Chernobyl disaster site, where the reactor was encased in an enormous ...
For example, normal risk for a female without the exposure from Fukushima to develop thyroid cancer is 0.75%. A 70% increase to 0.75% only increases the risk to 1.25%.5 Given that any person's risk of developing cancer in their lifetime is over 30%, a potential absolute increase of 0.5% is statistically undetectable.
Case Study on Fukushima Nuclear Disaster. January 2023. Authors: Ashwin Poudel. VIT University.
Engineering Ethics - Chernobyl's Case Study. The Chernobyl disaster was nuclear accident that occurred at Chernobyl Nuclear Power Plant on April 26, 1986. A nuclear meltdown in one of the reactors caused a fire that sent a plume of radioactive fallout that eventually spread all over Europe. Chernobyl nuclear reactor plant, built at the banks ...
The experts projected 220,000 fatalities if terrorists used a nuclear weapon in Japan. A third case concerned a first strike by the United States followed by retaliatory action by North Korea ...