Impact of climate change on Indian agriculture: new evidence from the autoregressive distributed lag approach
- Published: 15 February 2024
- Volume 8 , pages 377–394, ( 2024 )

Cite this article
- Mohammad Azhar Ud Din ORCID: orcid.org/0000-0002-8708-4758 1 &
- Shaukat Haseen 1
134 Accesses
Explore all metrics
A Correction to this article was published on 27 February 2024
This article has been updated
Climate change constitutes one of the most critical challenges of the contemporary period and can affect various sectors of economies across the globe, the agricultural sector is not an exception. This study aimed to assess the impact of climate change on India’s agricultural sector from 1990 to 2020. The autoregressive distributed lag (ARDL) approach was utilized to determine the short-run and long-run relationships between variables such as carbon dioxide emissions, temperature, energy utilization, and fertilizer consumption. The ARDL method and the Johansen and Juselius cointegration test both supported the existence of a significant and long relationship among the selected variables. The estimated short- and long-run findings showed that carbon dioxide emissions (CO 2 ), temperature, and energy consumption affect agricultural yield positively and significantly. These findings have several implications for the Indian economy. With a large population dependent on agriculture, improved productivity can directly impact food security and rural income, consequently leading to the country’s overall economic development. Enhanced agricultural output due to these factors may potentially lead to surplus production, allowing India to export more agricultural produce. This can positively impact the country’s trade balance and generate revenue through exports.
This is a preview of subscription content, log in via an institution to check access.
Access this article
Price includes VAT (Russian Federation)
Instant access to the full article PDF.
Rent this article via DeepDyve
Institutional subscriptions

Similar content being viewed by others
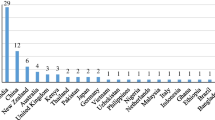
Climate-smart agriculture: adoption, impacts, and implications for sustainable development
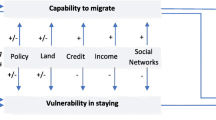
The impact of climate change on migration: a synthesis of recent empirical insights

Dynamic Approach to Study Relationship Among Carbon Dioxide Emissions, Urbanization, and Economic Growth in BRICS Countries
Data availability.
The data that support the findings of this study are available from the author upon reasonable request.
Change history
27 february 2024.
A Correction to this paper has been published: https://doi.org/10.1007/s41685-024-00332-y
Adams RM, Hurd BH, Lenhart S, Leary N (1998) Effects of global climate change on agriculture: an interpretative review. Climate Res 11(1):19–30
Article Google Scholar
Adamu MK, Negeso KD (2020) Effect of climate change on agricultural output in Ethiopia. J Eco Dev Environ People 9(3):6
Agba DZ, Adewara SO, Adama JI, Adzer KT, Atoyebi GO (2017) Analysis of the effects of climate change on crop output in Nigeria. Am J Climate Change 6(3):554–571. https://doi.org/10.4236/ajcc.2017.63028
Ahsan F, Chandio AA, Fang W (2020) Climate change impacts on cereal crops production in Pakistan: evidence from cointegration analysis. International Journal of Climate Change Strategies and Management 12(2):257–269. https://doi.org/10.1108/IJCCSM-04-2019-0020
Almazroui M, Saeed S, Saeed F, Islam MN, Ismail M (2020) Projections of precipitation and temperature over the South Asian countries in CMIP6. Earth Systems and Environment 4(2):297–320
Amponsah L, Kofi Hoggar G, Yeboah Asuamah S (2015) Climate change and agriculture: modelling the impact of carbon dioxide emission on cereal yield in Ghana. Agric Food Sci Res 2(2):32–38
Google Scholar
Baig IA, Chandio AA, Ozturk I, Kumar P, Khan ZA, Salam MdA (2022) Assessing the long- and short-run asymmetrical effects of climate change on rice production: empirical evidence from India. Environ Sci Pollut Res 29(23):34209–34230. https://doi.org/10.1007/s11356-021-18014
Bandara JS, Cai Y (2014) The impact of climate change on food crop productivity, food prices and food security in South Asia. Economic Analysis and Policy 44(4):451–465. https://doi.org/10.1016/j.eap.2014.09.005
Barnwal P, Kotani K (2013) Climatic impacts across agricultural crop yield distributions: an application of quantile regression on rice crops in Andhra Pradesh, India. Ecol Econ 87:95–109
Ben Zaied Y, Ben Cheikh N (2015) Long-run versus short-run analysis of climate change impacts on agricultural crops. Environ Model Assess 20(3):259–271. https://doi.org/10.1007/s10666-014-9432-4
Birthal PS, Khan T, Negi DS, Agarwal S (2014) Impact of climate change on yields of major food crops in India: implications for food security. Agric Econ Res Rev 27(2):145–155
Bouznit M, Aïssaoui R (2023) The impacts of climate change factors and innovative capabilities on food production in Algeria: evidence from ARDL model. Environ Dev Sustain. https://doi.org/10.1007/s10668-023-03627-w
Brown RL, Durbin J, Evans JM (1975) Techniques for testing the constancy of regression relationships over time. J Roy Stat Soc: Ser B (methodol) 37(2):149–163
Ceesay EK, Ben Omar Ndiaye M (2022) Climate change, food security and economic growth nexus in the Gambia: evidence from an econometrics analysis. Research in Globalization 5:100089. https://doi.org/10.1016/j.resglo.2022.100089
Chandio AA, Jiang Y, Rehman A, Rauf A (2020a) Short and long-run impacts of climate change on agriculture: an empirical evidence from China. International Journal of Climate Change Strategies and Management 12(2):201–221. https://doi.org/10.1108/IJCCSM-05-2019-0026
Chandio AA, Magsi H, Ozturk I (2020b) Examining the effects of climate change on rice production: case study of Pakistan. Environ Sci Pollut Res 27(8):7812–7822. https://doi.org/10.1007/s11356-019-07486-9
Chandio AA, Ozturk I, Akram W, Ahmad F, Mirani AA (2020c) Empirical analysis of climate change factors affecting cereal yield: evidence from Turkey. Environ Sci Pollut Res 27(11):11944–11957. https://doi.org/10.1007/s11356-020-07739-y
Chandio AA, Jiang Y, Fatima T, Ahmad F, Ahmad M, Li J (2022) Assessing the impacts of climate change on cereal production in Bangladesh: evidence from ARDL modeling approach. International Journal of Climate Change Strategies and Management. 14(2):125–147
Deressa T, Hassan R, Poonyth D (2005) Measuring the impact of climate change on South African agriculture: the case of sugarcane growing regions. Agrekon 44(4):524–542
Dickey DA, Fuller WA (1981) Likelihood ratio statistics for autoregressive time series with a unit root. Econometrica J Econometric Soc 49(4):1057–1072
Dumrul Y, Kilicaslan Z (2017) Economic impacts of climate change on agriculture: empirical evidence from ARDL approach for Turkey. Journal of Business Economics and Finance 6(4):336–347
Eckstein D, Künzel V, Schäfer L (2021) The Global Climate Risk Index 2021. Germanwatch, Bonn
Edoja PE, Aye GC, Abu O (2016) Dynamic relationship among CO 2 emission, agricultural productivity and food security in Nigeria. Cogent Economics & Finance 4(1):1204809. https://doi.org/10.1080/23322039.2016.1204809
Engle RF, Granger CW (1987) Co-integration and error correction: representation, estimation, and testing. Econometrica 55(2):251–276
Eregha PB, Babatolu JS, Akinnubi RT (2014) Climate change and crop production in Nigeria: an error correction modelling approach. International Journal of Energy Economics and Policy 4(2):297–311
Eshete ZS, Mulatu DW, Gatiso TG (2020) CO 2 emissions, agricultural productivity and welfare in Ethiopia. International Journal of Climate Change Strategies and Management 12(5):687–704. https://doi.org/10.1108/IJCCSM-07-2019-0046
Gul A, Xiumin W, Chandio AA, Rehman A, Siyal SA, Asare I (2022) Tracking the effect of climatic and non-climatic elements on rice production in Pakistan using the ARDL approach. Environ Sci Pollut Res 29(21):31886–31900
Guntukula R (2020) Assessing the impact of climate change on Indian agriculture: EVIDENCE from major crop yields. J Public Aff 20(1):e2040. https://doi.org/10.1002/pa.2040
Gupta A, Pathak H (2016). Climate change and Indian Agriculture: A Thematic Report of National Mission on Strategic Knowledge for Climate Change (NMSKCC) under National Action Plan on Climate Change (NAPCC)—Google Search (n.d.). Retrieved12 Jan 2023 from Google
Janjua PZ, Samad G, Khan N (2014) Climate change and wheat production in Pakistan: an autoregressive distributed lag approach. NJAS Wageningen Journal of Life Sciences 68:13–19. https://doi.org/10.1016/j.njas.2013.11.002
Johansen S (1988) Statistical analysis of cointegration vectors. J Econ Dyn Control 12(2/3):231–254
Johansen S, Juselius K (1990) Maximum likelihood estimation and inference on cointegration—with applications to the demand for money. Oxford Bull Econ Stat 52(2):169–210
Kaufmann RK, Snell SE (1997) A biophysical model of corn yield: integrating climatic and social determinants. Am J Agr Econ 79(1):178–190. https://doi.org/10.2307/1243952
Li X, Takahashi T, Suzuki N, Kaiser HM (2011) The impact of climate change on maize yields in the United States and China. Agric Syst 104(4):348–353. https://doi.org/10.1016/j.agsy.2010.12.006
Lili H, Shuwen N, Li M, Jing Q (2011) The empirical analysis of the relationship between agricultural economic growth and energy consumption in regions of China, computer distributed control and intelligent environmental monitoring (CDCIEM). 2011 International Conference on IEEE, pp 1418–1426
Mishra D, Sahu NC (2014) Economic impact of climate change on agriculture sector of coastal Odisha. APCBEE Proc 10:241–245
Nasrullah M, Rizwanullah M, Yu X, Jo H, Sohail MT, Liang L (2021) Autoregressive distributed lag (ARDL) approach to study the impact of climate change and other factors on rice production in South Korea. Journal of Water and Climate Change 12(6):2256–2270. https://doi.org/10.2166/wcc.2021.030
Nath PK, Behera B (2011) A critical review of impact of and adaptation to climate change in developed and developing economies. Environ Dev Sustain 13(1):141–162. https://doi.org/10.1007/s10668-010-9253-9
Noorunnahar M, Mila FA, Ila Haque FT (2023) Does the supply response of maize suffer from climate change in Bangladesh? Empirical evidence using ARDL approach. Journal of Agriculture and Food Research 14:100667. https://doi.org/10.1016/j.jafr.2023.100667
Pesaran MH, Shin Y (1998) An autoregressive distributed-lag modelling approach to cointegration analysis. Econom Soc Monogr 31:371–413
Pesaran MH, Shin Y, Smith RJ (2001) Bounds testing approaches to the analysis of level relationships. J Appl Economet 16(3):289–326. https://doi.org/10.1002/jae.616
Phillips PC, Perron P (1988) Testing for a unit root in time series regression. Biometrika 75(2):335–346
Praveen B, Sharma P (2020) Climate change and its impacts on Indian agriculture: an econometric analysis. J Public Aff 20(1):e1972. https://doi.org/10.1002/pa.1972
Raeeni AAG, Hosseini S, Moghaddasi R (2019) How energy consumption is related to agricultural growth and export: an econometric analysis on Iranian data. Energy Rep 5:50–53. https://doi.org/10.1016/j.egyr.2018.11.005
Raju G, Phanindra G (2018) Climate sensitivity on rice yield: evidence from Telangana. India 31:199
Rehman A, Chandio AA, Hussain I, Jingdong L (2019) Fertilizer consumption, water availability and credit distribution: major factors affecting agricultural productivity in Pakistan. J Saudi Soc Agric Sci 18(3):269–274. https://doi.org/10.1016/j.jssas.2017.08.002
Swart R, Robinson J, Cohen S (2003) Climate change and sustainable development: expanding the options. Climate Policy 3(sup1):S19–S40
Van Huong N, Minh Nguyet BT, Van Hung H, Minh Duc H, Van Chuong N, Do Tri M, Van Hien D, Van Hien P (2022) Economic impact of climate change on agriculture: a case of Vietnam. AgBioforum 24(1):1–12
Wang J, Vanga SK, Saxena R, Orsat V, Raghavan V (2018) Effect of climate change on the yield of cereal crops: a review. Climate 6(2):41
Warrick RA (1988) Carbon dioxide, climatic change and agriculture. Geogr J 154(2):221–233. https://doi.org/10.2307/633848
Warsame AA, Sheik-Ali IA, Ali AO, Sarkodie SA (2021) Climate change and crop production nexus in Somalia: an empirical evidence from ARDL technique. Environ Sci Pollut Res 28(16):19838–19850. https://doi.org/10.1007/s11356-020-11739-3
World Bank (2020) World development indicators. The World Bank Group, Washington, DC
Xiang X, Solaymani S (2022) Change in cereal production caused by climate change in Malaysia. Eco Inform 70:101741. https://doi.org/10.1016/j.ecoinf.2022.101741
Xiang H, Ch P, Nawaz MA, Chupradit S, Fatima A, Sadiq M (2021) Integration and economic viability of fueling the future with green hydrogen: an integration of its determinants from renewable economics. Int J Hydrogen Energy 46(77):38145–38162. https://doi.org/10.1016/j.ijhydene.2021.09.067
Yawson DO, Mulholland BJ, Ball T, Adu MO, Mohan S, White PJ (2017) Effect of climate and agricultural land use changes on UK feed barley production and food security to the 2050s. Land 6(4):74
Zhai S, Song G, Qin Y, Ye X, Lee J (2017) Modeling the impacts of climate change and technical progress on the wheat yield in inland China: an autoregressive distributed lag approach. PLoS ONE 12(9):e0184474
Download references
Acknowledgements
We express our profound gratitude to the anonymous reviewers for their invaluable suggestions, which significantly enhanced the quality of the paper.
The author(s) received no financial support for the research, authorship, and/or publication of this article.
Author information
Authors and affiliations.
Department of Economics, AMU, Aligarh, UP, India
Mohammad Azhar Ud Din & Shaukat Haseen
You can also search for this author in PubMed Google Scholar
Contributions
SH has written the introduction and literature part of the study. Methodology, data analysis has been written by MAUD. Conclusion was written jointly by SH and MAUD. All authors read and approved the manuscript.
Corresponding author
Correspondence to Mohammad Azhar Ud Din .
Ethics declarations
Competing interests.
The author(s) declare that there are no competing interest with respect to the research, authorship, and/or publication of this article.
Ethics approval
This original manuscript has not been submitted to another journal. The publication is free of fabrication or falsehoods.
Consent for publication
All authors read and approved the manuscript for submission.
Additional information
Publisher's note.
Springer Nature remains neutral with regard to jurisdictional claims in published maps and institutional affiliations.
The original online version of this article was revised due to the time span was published incorrectly under the abstract and corrected in this version.
About this article
Ud Din, M.A., Haseen, S. Impact of climate change on Indian agriculture: new evidence from the autoregressive distributed lag approach. Asia-Pac J Reg Sci 8 , 377–394 (2024). https://doi.org/10.1007/s41685-023-00327-1
Download citation
Received : 23 June 2023
Accepted : 08 December 2023
Published : 15 February 2024
Issue Date : June 2024
DOI : https://doi.org/10.1007/s41685-023-00327-1
Share this article
Anyone you share the following link with will be able to read this content:
Sorry, a shareable link is not currently available for this article.
Provided by the Springer Nature SharedIt content-sharing initiative
- Agriculture
- Climate change
- ARDL technique
JEL Classification
- Find a journal
- Publish with us
- Track your research
The Official Journal of the Pan-Pacific Association of Input-Output Studies (PAPAIOS)
- Open access
- Published: 04 January 2024
Economy-wide impact of climate smart agriculture in India: a SAM framework
- Ananya Ajatasatru 1 ,
- Vishnu Prabhu 1 ,
- Barun Deb Pal 2 &
- Kakali Mukhopadhyay ORCID: orcid.org/0000-0003-0691-1019 1 , 3
Journal of Economic Structures volume 13 , Article number: 4 ( 2024 ) Cite this article
2762 Accesses
1 Citations
7 Altmetric
Metrics details
In the context of climate change, the Indian agricultural sector treads in a certain duality between promoting food security in response to the increasing population, but at the same time in ensuring environmental sustainability, and sustained economic growth, especially in developing countries like India. The concept of Climate Smart Agriculture (CSA) emerged from the recognition of this duality. Using the Indian Social Accounting Matrix (SAM) 2017–18, the economy-wide effects arising out of agricultural interventions were estimated, keeping accord with the impacts on sectoral outputs and household incomes from the adoption of varying CSA interventions such as Conservation Agriculture, System of Rice and Wheat Intensification (SRI-SWI) and Natural Farming, fitting the three-pillared criterion of CSA—(1) Productivity (2) Adaptation and (3) Mitigation. Additionally, a shift in cropping patterns from Paddy and Wheat to less emission-intensive crops was also studied. Results show that SRI-SWI provides the highest economy-wide impacts while accounting for lower GHG and water footprint. Alternative crops such as Maize, Sorghum, and Millet have minimal increase in income and output effects while having lower water and carbon intensity compared to rice and wheat. The current study would sensitize policymakers to prioritize suitable policy and institutional measures for upscaling climate smart interventions in India.
1 Introduction
Agricultural sector plays a pivotal role in promoting food security in response to the increasing population. In 2020, it was estimated that nearly 690 million people, or 8.9% of the global population live in hunger (FAO 2020a , b ). This situation is expected to aggravate the need of producing 70% more food by 2050 to feed an estimated 9 billion people. Developing countries with close agrarian economic linkages are particularly more vulnerable to the effects of climate change and are headed towards severe livelihood challenges due to declining agricultural productivity and increasing food demand, culminating in income and food insecurity (Mendelsohn 2008 ; Fischer et al. 2005 ; Wheeler and Von Braun 2013 ). Calzadilla et al. ( 2013 ) predict by 2050, there will be an overall minimum loss in global welfare and GDP of about USD 268 billion and USD 265 billion respectively, due to a fall in agricultural production from the impacts of climate change. In a country like India, 54.6% of the total workforce is employed in the agriculture sector, contributing 17.8% to the country’s Gross Value Added (GVA) (FAO 2020a , b ),
The emissions from the agriculture sector are generated from production and post-farm processes, accounting for 18.4% of the total global greenhouse emissions in 2016 (Ritchie & Roser 2020 ). The GHGs N 2 O and CH 4 constitute a higher share of agricultural emissions with Global Warming Potential (GWP) of 34 and 298 times (IPCC 2013 ). Of the countries among the top ten GHG emissions from paddy cultivation, nine countries are located in Asia of which, India ranks second (Fig. 1 ).
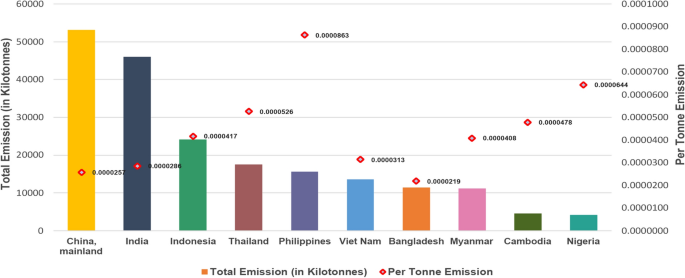
Top 10 GHG Emitting Countries from Paddy Cultivation (2010–19)
In the case of India, 14% of the cumulative CO 2 eq. Emissions were attributed to the agriculture sector (UNFCCC 2021 ). The category-wise agricultural emissions in India are presented in Fig. 2 .
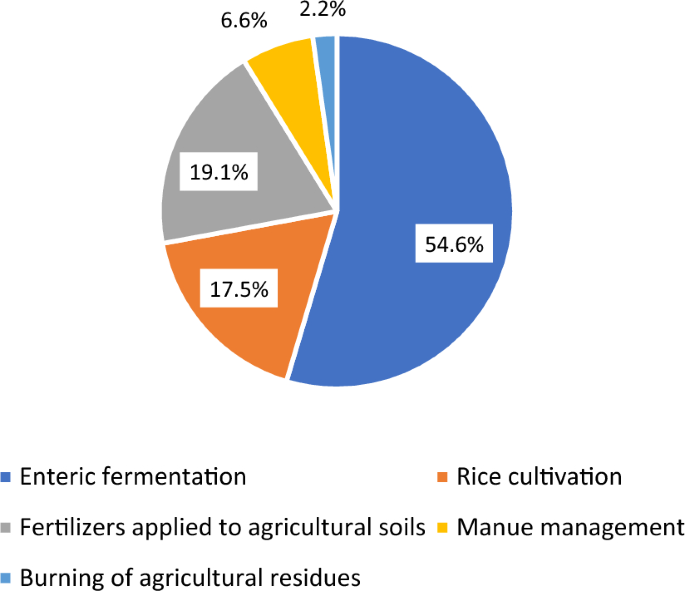
Category-wise CO 2 eq. Agricultural emissions in India 2016 (in percentage)
The agricultural GHG emission is primarily attributed to enteric emissions of livestock and cultivation of emission-intensive foodgrain crops such as rice and wheat, contributing 41% of the anthropogenic methane and 74% of nitrous oxide emissions (FAO 2020a , b ; Pathak Bhatia and Jain 2014 ; Kashyap and Agarwal 2020 ) (see Appendix 1 ). The CH 4 emissions constitute the highest share (54.6%) and N 2 O emissions are through the application of fertilizers to agricultural soils (19.1%). Rice cultivation also leads to CH 4 and N 2 O emissions, with a GWP of 467, which is 169% higher compared to wheat and maize (Linquist et al. 2012 ). In 2021, rice cultivation encompassed an area of 43 million hectares which was 34% of total foodgrain area coverage in the country (GOI 2022a , b , c ) and is the second highest rice producer in the world (Shahbandeh 2022 ). Along with the agriculture sector contributing significantly towards non-CO 2 emissions, it is also input-intensive, especially in energy, water, fertilizers, and pesticides. Indian agriculture accounts for 80% of the total freshwater consumption in the country (Dhawan 2017 ; Sen 2018 ). The agricultural water consumption is largely unregulated and inefficient (Dhawan 2017 ). This leads to the over-extraction of groundwater resources, thus making India the largest consumer of groundwater in the world, more than the USA and China combined (Jovial 2022 ). Globally, around 40% of irrigation is sourced from groundwater whereas in India it is well over 50%. The electricity consumption by the agriculture sector accounts for 17.35% share (CEA 2023 ), however, the agricultural power load is becoming an increasing challenge (PIB 2015 ). Between 2010 and 2021, agricultural power consumption increased by 80% (CEIC 2021 ) in the country.
At the policy and institutional levels, the Government of India has taken several steps that attempt to create sustainability in agricultural production systems. Initiatives such as the Paramparagat Krishi Vikas Yojana (organic farming) are aimed at reducing chemical fertilizer usage which is 209 kg/ha in India, compared to the world average of 146 kg/ha (World Bank 2022 ). The government has set a target of achieving 10% of the cultivated area into organic farmlands by 2025 (GOI 2016 ). Additional programs in tackling unsustainable agricultural practices have been initiated such as Pradhan Mantri Krishi Sinchayee Yojana (efficient irrigation), Neeranchal (watershed development), National Initiative on Climate Resilient Agriculture (NICRA), reduction in fossil fuel subsidies, and National Adaptation Fund (Praveen and Ramachandran 2020 ).
The government of India is also promoting millets as an alternative to other foodgrains such as wheat and rice. India is responsible for 20% of global millet production (GOI 2022a , b , c ). To this end, at the request of the government, the United Nations declared 2023 as the International Year of Millets. Davis et al. ( 2019 ) show that the substitution of rice in each district with alternative cereals leads to a significant decrease in blue water footprint while ensuring the availability of higher nutrient content such as protein (+ 1%), iron (+ 27%), and zinc (+ 13%). Furthermore, these alternative cereals have a significantly lesser GHG footprint as, unlike rice, they do not require continuous flooding conditions. While there aren’t any methane emissions compared to wheat, they require the same or lesser fertilizer inputs, thus curbing down the nitrogen emissions (Sah and Devakumar 2018 ). Simultaneously, ensuring judicious resource utilization and sustained economic growth is essential for a country’s long-term sustenance. The concept of Climate Smart Agriculture (CSA) emerged from the recognition of this duality of environmental sustainability and economic stability; proposed by FAO ( 2009 ) to address the impact of both the 2008 food price crisis and global warming on the agricultural sector. Even though FAO has set it to be context specific in accordance with local needs, the operationalization of CSA has so far also drawn heavily on conventional, top–down, technical solutions to achieve impact at scale.
The three objectives of CSA of productivity, adaptation, and mitigation can be pursued with a viable spectrum of varied options, ranging from agroforestry with strict adherence to organic practices to technologically intensive practices advocating for a higher level of mechanization and biotechnology. Lipper and Zilberman ( 2018 ) consider CSA to be a relatively new concept which attempts to integrate responses to climate change into sustainable agricultural development, hence it should be considered more of a guiding objective rather than a fixed prescriptive solution. The agenda of CSA is outcome oriented compared to agroecological approaches which are more method driven, while the latter is consistent with the former but not vice-versa. A better pathway to the CSA’s Sustainable Agricultural Intensification (SAI) has been raised to be Agroecological Intensification (AI), which is pro-organic and adverse to any form of mechanization or biotechnological push towards agriculture.
Mockshell and Kamanda ( 2018 ) identify both Conservation Agriculture (CA) and the System of Rice Intensification (SRI) under the purview of a blended view of sustainability, recognizing the synergies and tradeoffs between the two approaches to determine the best practices through an integrated approach. The overlap between CSA and Agroecology (AE) is at landscape and farm levels where, CSA is more about the policy, finance, and institutional aspects; being more influential at a national and global scale aimed at large transformational changes. Meanwhile, AE is more involved at the farm level in accordance with the specific agronomic behaviour of the biotope (Saj et al. 2017 ). The present study has considered four scenarios of varying practices under the ambit of CSA in consonance of AE along with considerations for promoting sustainable cropping patterns by assessing the emission impacts of the dominant foodgrain cropping systems in India.
1.1 Relevance of CSA in Indian rice–wheat-cropping system (RWCS)
The Indian agricultural sector has been considered vital towards country’s economy and food sovereignty and was also found to be the only sector that was resilient in the period of economic downturn, during the COVID-19 pandemic, with a positive growth rate of 3.4% in GVA (Kapil 2021 ). Although, among the myriad of problems in the agriculture sector; there have been persisting concerns over low productivity in Indian agricultural yield, which has been further exacerbated by the projections of uncertain adversities that may arise due to climate change. Simulating the relation between the effects of climate change on agriculture is often complex along with uncertain projections. This is due to wide variability in agro-climatic conditions, farming practices, and technologies among agronomically diverse regions of India. Yet most studies do predict the varying level of crop losses due to climate change impacts (Mall et al. 2006 ). A majority of studies that are concerned with simulating climate change impact delve primarily into cereals, pulses, and oilseeds, as they are regarded as the main sources of human and livestock calories globally for instilled food security.
The focus on rice and wheat cropping systems is of particular significance in the Indian context, as they constitute the staple of the Indian diet and household consumption basket. India is also the second largest producer of rice and wheat; accounting for 76% of the total foodgrain output and 59% of the area under foodgrains (GOI 2020 ). Birthal et al. ( 2014 ) predict that in the medium term (2035) there will be a decline in the yield, from 2.5 to 7.1% in rice and a decline of 0.5–8.3% in wheat, while in the long term (by 2100) a decline in yield of 5.9–15.4% in rice and 8.2–22% in wheat is to be expected.
Thus, the triple objective framework set by the CSA approach holds great relevance in instilling resilience. Therefore, practices such as CA have been identified to be particularly relevant in relation to smallholder farmers’ interests (Mizik 2021 ) which inherently adhere to the principles of AE, while also vying for the objectives set forth under CSA. One of the most promising CSA practices of SRI which initially faced apprehensions due to being perceived as unviable, particularly in developing countries due to labour scarcity and yield loss has seen a resurgence in adoption (Thakur and Uphoff 2017 ). This renewed emphasis on SRI practices is driven by its potential conservation of water and farmer experiences which is being reworked to be context-specific from an AE perspective. Similarly. in this study we have taken into account Zero Budget Natural Farming (ZBNF), a practice specific to India which adheres with the objectives set by CSA and also originates from smallholder community movement (Ghosh 2019 ).
1.2 Conservation agriculture
Conservation Agriculture (CA), is a holistic approach, characterized by numerous interactions among households, crops, and livestock, to create a sustainable farming system (Hobbs et al. 2008 ). The CA approach involves a wide range of practices that are part of its three main principles: (i) the minimization of soil disturbance from mechanical tillage, (ii) the maintenance of a permanent organic soil cover, and (iii) the diversification of crop species through crop rotation (Jat et al. 2020 ). The study by Aune ( 2012 ) shows CA achieves similar levels of yield as the conventional practices while having reduced emissions. Thus, our study has ascertained the economy-wide benefits of adopting CA beyond the farm household, while also qualifying the three-pillar criteria set by the CSA framework; through quantified water and GHG footprint reduction.
The practice of CA came of particular relevance in the context of the Rice–Wheat Cropping Systems (RWCS) of Indo-Gangetic Plains (IGP). The Indian IGP is spread over the states of Punjab, Haryana, Delhi, Uttar Pradesh, Bihar, and West Bengal, where RWCS is dominant, covering about 10 million Ha (Timsina and Connor 2001 ). Prior to the Indian green revolution, rice cultivation was quite limited to pockets of high quality but low productivity Basmati rice (Prasad and Nagarajan 2004 ). This invited opportunity for the cultivation of the high-yielding variety rice cultivars such as IR-8 in the non-traditional areas of the IGP. Footnote 1 This pattern of RWCS soon took over in large areas, particularly in the upper IGP due to it having lesser associated risk and high market value, replacing other foodgrains, oilseeds, and pulses, although, this pattern of cropping has created several issues pertaining to water scarcity, associated Global Warming Potential (GWP) and loss in diversity and resilience in crop varieties.
The introduction of CA in the IGP emerged towards addressing the delayed Rice–Wheat cropping cycle, which arises from drained soil fertility post-water intensive Rice cropping period resulting in planting delays for Wheat. Initially, the idea of CA was instilled in form of Zero-Tillage Wheat through the use of mechanized inputs such as seed drillers with efficient fertilizer delivery mechanism. These initial adoption of Zero-Tillage Wheat practices, paved the way for technological developments among the Indian manufacturers through innovations such as fixed openers, happy seeder technology and other mechanisations that allow for Zero Tillage cropping for other crops such as maize, legumes, mustard and sunflower. The recent progress to further enhance the CA approach has resulted in the substitution from traditional puddled transplanted rice to Zero-Tillage Direct-Seeded Rice (DSR-ZT). This form of farmer-participatory research led to the wider spread and rapid adoption of ZT wheat (Hobbs et al. 2019 ). Currently, about 1.5 million Ha is under partial conservation agriculture (Jat et al. 2014 ). The practice of CA has been widely recognized under CSA due to it providing yield enhancements, resource efficiencies, emission savings, and resilience in case of uncertain rainfall conditions (Kassam et al. 2015 ; Michler et al. 2019 ; Bhattacharyya et al. 2015 ).
1.3 System of crop intensification
The system of crop intensification emerged as derivations of SRI principles to other crops such as wheat, finger millet, maize, and sugarcane, also varied types of oilseeds, legumes, and even vegetables primarily in African and Asian countries (Abraham et al. 2014 ; Thakur and Uphoff 2017 ). The four principles by which SRI has been classified consistently are: (1) Early, quick, and healthy plant establishment; (2) reduced plant density; (3) improved soil conditions; and (4) controlled water application. While these set of practices are constant, the means of implementing them may vary suiting the farmer’s socioeconomic needs and agroecological context. The practices associated with the system of crop intensification often are found to be labour saving upon mechanization of certain processes involved in SRI-SWI such as land-leveling, weeding, and transplanting. (Rana et al. 2017 ; Saxena et al. 2019 ).
The basic premise of SRI lies in a management-oriented innovation that is aligned towards increasing productivity through optimal management of plants, soil, water, and nutrients. Footnote 2 The initial adaptation constraints to SRI were over concerns of labour scarcity along with risks of yield loss which were witnessed during the on-farm experimentations in Madagascar (Barrett et al. 2004 ). The labour intensive nature of SRI is context specific depending upon the process of farmer adaptation towards implementation of the core tenets of SRI. Footnote 3
In India, SRI initially got promoted primarily in the southern states. Footnote 4 Although, at present there aren’t any specific government data on area coverage under SRI but Gupta et al. ( 2021 ) report about 3–4 million hectares under SRI, adopted primarily by the small and marginal farmers.
Similar to SRI, the main practices for SWI are management-oriented which are interventions for root development and of intensive care. The primary motivations behind these adoptions of SRI principles for other crops have been driven by the success stories of farmers facing resource constraints but witnessing higher yields from adopting SRI principles in other crops. SWI was first tested in 2006, by farmers associated with the People’s Science Institute (PSI). The pilot project on SWI started with 40 farmers from 25 villages in the states of Himachal Pradesh and Uttarakhand, which found positive yield growth. In the successive three years, the practice got spread to other states of Madhya Pradesh, Bihar, Punjab, Odisha, Uttar Pradesh, and Chhattisgarh (PRADAN 2008 ; Biswas and Das 2021 ).
1.4 Zero budget natural farming
ZBNF aims at the complete elimination of chemically induced fertilizers and pesticides leading to a significant reduction in input costs, leading up to zero external financing while aiming at increased crop yield (Biswas 2020 ). The four pillars of ZBNF are given to be: (1) Natural, chemical-free seed treatment to retain micro-organisms in the soil, preferably under a poly-cropping system. (2) Natural, organic tonic preparation for retaining nutrients in the soil on the root level, with complete elimination of fertilizers. (3) Circulation of air and conservation of water and moisture within the soil and reduce the need for soil tillage, and soil aeration. (4) Integration of livestock with crops for biological and economic synergies is recommended (Korav, Dhaka, Chaudhury, and Mamatha 2020 ).
Though, differences have been cited between Natural farming and Organic farming mainly in the usage of vermicomposting, manure or the total expenditure where organic farming was found to be costlier, both promote chemical-free agricultural techniques. Overall, there is an increased labor requirement due to elaborate set of timely interventions, yet there’s also higher environmental benefits and cost savings. The ZBNF movement extends beyond CA by opposing use of any external inputs and synthetic fertilizers (Palekar 2006 ). Currently, as per varying estimates, ZBNF practices in the southern states of Karnataka and Andhra Pradesh are approximately 700,000 ha. while pilot initiatives have been undertaken in the states of Himachal Pradesh, Maharashtra, Odisha, and Chattishgarh.
The practice is primarily oriented and has been receptive to groups of small, marginal, and tribal farmers (Khadse et al. 2018 ). The attempt here has been towards transformation rather than adjustments in existing practices, hence its knowledge-intensive nature being a potential barrier towards wider adoption. The success of the ZBNF movement has been through the persevering social capital focused on relations of trust; reciprocity and exchange; common rules; norms and sanctions and connectedness (Bharucha et al. 2020 ).
Given this backdrop, the objective of our study is to estimate economy-wide effects arising out of agricultural interventions that fit with the three-pillared criterion of CSA—(1) Productivity, (2) Adaptation, and (3) Mitigation, while being considerate of the local niches, finding their origin either in community driven movements or on-farm experiments. The purpose of studying the economy-wide effect of Climate Smart Agriculture (CSA) is to ascertain the impact on the economy upon scaling particular agricultural intervention. Also, it portrays the impact of additional yield gain on the household incomes and the associated sectors, which in turn help in priority setting and country-wide policy investment planning. This is in cognizance of the first criterion of CSA productivity. Further, in lieu of the second and third criterion pertaining to adaptation and mitigation, our study has also taken into account the water savings (for assessing the second criterion; adaptation) and reductions in GWP (for assessing the third criterion; mitigation) of CSA practices. To calculate direct and indirect water usage, based on per tonne production, water coefficients were estimated based on the water footprints calculated by Mekonnen and Hoekstra ( 2011 ). Similarly, the respective CO2¬eq. coefficients were collected from multiple studies. The direct and indirect intensities have been further calculated based on methodology set by Perman et al. ( 2011 ). Apart from changes in practice and technological interventions, we have also considered changes in cropping patterns primarily viable substitutions in the dominant RWCS of India, with other foodgrain crops that aren’t emission intensive.
The subsequent Sect. 2 provides detailed information on the Methodology and Scenario development adopted for this study to evaluate Climate Smart Agriculture approaches. Section 3 provides the results from the scenarios followed by Discussion in Sect. 4 and Conclusion and Policy recommendation in Sect. 5 .
2 Materials and methods
2.1 social accounting matrix for macroeconomic policy.
Social Accounting Matrix (SAM) is an accounting framework in form of a square matrix that portrays the flow of funds in an economy through cells that represent transactions from column accounts to row accounts. The structure of SAM is generally composed of seven types of accounts; activities, commodities, factors of production, households, government, savings and investment (S-I), and the rest of the world (Breisinger, Thomas, and Thurlow 2009 ).
Several SAM-based studies have been undertaken in the past towards capturing the role of agriculture as a driver of growth in the overall economy. Further, the agricultural sector is of particular significance in many developing nations as it accounts for a substantial part of the economy and its role in improving household incomes (Havinga et al. 1987 ; Townsend and McDonald 1998 ; Pyatt and Round 1977 ). The focus of these studies has been at analyzing the interdepencies between the agricultural sector and the rest of the economy or towards estimating the impacts and distributive effects of policies. While being one of the first papers to take into account the aspects of technical changes in agricultural sectors and in consequence, their macroeconomic effects have been studied by Khan and Thorbecke ( 1989 ). Their study over the SAM multipliers analyses the policy outcomes of technology choices between “traditional” and “modern” techniques by disaggregating the types of commodity in relation to the differing production techniques, in the context of the economy of Indonesia.
In the Indian context, one of the first attempts at building a SAM was by Sarkar and Subbarao ( 1981 ), although prior studies pertaining to sectoral linkages had already been undertaken using an Input–Output approach (Hazari 1970 ; Hashim 1971 ). While a recent study by Mythili and Harak ( 2012 ) emphasizes the importance of agricultural sector in it’s income-generating potential, even if the Indian economy has gone through a structural transformation in the post-liberalization era. The service sector transition has become prominient over the years, although the employment growth in service sector has been lagging. The study also further accentuates, the missing link between agriculture and service sector, due to which the spillover effects have been limited from the latter.
There has not been any studies for India that use the SAM framework to assess the direct and indirect, sectoral and household impacts arising out of changes in agricultural practices. To this end, our study has taken into account the economywide impact of agroecological approaches towards agricultural development, while keeping the pillars of CSA intact. In this study, we have considered both the changes in technology and existing practices, along with propositions for shifting into alternative foodgrain cropping patterns, while meeting the three-pillared objectives of CSA. Therefore, the focus here is not only on increasing crop yields but also on its effects on household income patterns along with wider sectoral changes and also the associated resource savings (in terms of Water and GHG footprints). By taking into consideration the climate change ramifications from agricultural practices, our study is also aligned with the Sustainable Development Goals of ‘Taking Action against Climate Change (Goal 13). The usage of the SAM framework, hence provides a macro view of considered propositions, in contrast to most studies which are often at the farm level.
2.2 Methodological framework of SAM model
In this study, the 2017/18 Social Accounting Matrix for India published by the International Food Policy Research Institute (henceforth IFPRI-SAM) has been used (Pal et al. 2020 ). The 2017–18 IFPRI-SAM constitutes 112 sectors composed of 39 sectors belonging to agriculture and allied activities, 18 sectors relating to agriculture-based processing activities, 24 manufacturing sectors, 4 mining sectors, 3 utilities sector, 1 construction sector and 23 service sectors. The factors inputs are classified into labor, capital, and land. The labor has been classified into rural and urban, with a further division based on education level. Capital has been divided into 4 types: crop, live animal, mining, and other financial capital. The household has been divided into rural farm households, rural non-farm households, and urban households with five quintiles of income level (Pal et al. 2020 ). The IFPRI 2017–18 SAM has 5 endogenous accounts (Activities, Commodities, Factors, Enterprises, and Households) and 4 exogenous accounts (Government, Taxes, Savings-Investments, and Rest of the World) (IFPRI-SAM framework provided in Table 8 in the Appendix 2). Using IFPRI-SAM database as baseline of our study, we have followed the following steps in Table 1 to fulfill our objectives of this study.
Although the above table describes in detail various technical steps involved in this study, the SAM multiplier model needs special attention. Therefore, in the following paragraph we have described the SAM multiplier model and its various components in lieu of our objective of this study.
In this study, we have adopted SAM multiplier model described in the study by Breisinger, Thomas, and Thurlow, ( 2009 ). The SAM structure involved in that study is similar to the 2017–18 IFPRI-SAM structure. The SAM multiplier model dervied by Breisinger et al. ( 2009 ) is given below.
where, \(Z-NX1\) vector of output endogenously determined using multiplier model. ‘ N ’ is number of endogenous accounts. \(M- NXN\) coefficient Matrix, \(E - NX1\) Vector of Exogenous Variables.
\(I=NxN\) identity matrix.
In the above equation \({(I-M)}^{-1}\) is the multiplier matrix that describes the linkages effect on endogenous variable due to any exogenous shock. Therefore, it is worth noting that the multiplier impact depends on value of the coefficient matrix \(M\) . Further the selection of endogenous and exogenous variables largely depends on the research question the study concerns. In this study, we have considered government transactions, gross fixed capital formation, change in stock, direct and indirect taxes, and the rest of the world transactions as exogenous accounts to estimate SAM multiplier model.
The multiplier model derived above does not include climate smart crop cultivation activity account. Again, as the IFPRI-SAM has \(112\) activity output, \(112\) commodity output, 1 trade and transport margin, 13 types of value-added income, income of 15 types of households, together of them accounts \(253\) endogenous variables to be estimated using this SAM multiplier model. Hence, dimensions of \(Z\) , and \(E\) vectors in the multiplier model have \(253\) rows and the matrix \(M\) is a square matrix of dimension \(253x253.\) Again, the \(M\) matrix contains various components, and this is described in the Table 2 .
The \(M\) matrix presented in the above table has 8 non-zero components. The blank part of the above table represents zero elements of \(M\) matrix which implies no direct relation between the components. However, they have an indirect relationship due to the circular flow of income and inter-industry linkages. The multiplier matrix \({(I-M)}^{-1}\) captures both the indirect and direct relationship between the endogenous variables in SAM multiplier model. Thus, each component of multiplier matrix \({(I-M)}^{-1}\) has its own economic significance. For example, the components ( \(FAC X ACT\) ) record the impact on activity-wise gross value added due to any exogenous shock into the economy.
However, the above-described multiplier model does not consider environmental impacts. Therefore, we have incorporated impact on water and emission footprint in this multiplier model and the modified equations are as follows.
\(GHG\) = total GHG emissions of the economy,
\(g\) = The emission coefficients of respective activities (wherein, \(g\) is a column vector with direct emission per unit output of each activity sector, while for the other endogenous accounts is zero)
\(BLUWAT\) = total blue water use
\(w\) = water use intensity in agriculture sector (wherein, \(w\) is a column vector with water use per unit output of each associated agriculture activity sector, while for the other non-agriculture activity sectors and the endogenous accounts the value is zero)
Finally, as discussed in step 7 in the earlier table, we have incorporated different levels of adoption corresponding to different CSA scenarios described above. Results of the SAM multiplier model corresponding to above scenarios are described in the following section.
2.3 Intervention scenarios
The original Rice and Wheat sectors have been split to add two additional modified Rice and Wheat sectors where the technological interventions have been carried out and compared with their generic counterparts. The modified Rice and Wheat sectors, initially have the same input and value-added coefficients as the aggregated sectors. But towards simulating the effects of various intervention scenarios the input and value-added coefficients have been modified accordingly. Finally, to satisfy the Hawkins–Simon condition (implying that the column sum of coefficient matrix for activity column-commodity row being equal to 1), the changes in the intermediate inputs and value-added labour have been adjusted accordingly with the value-added capital. The cost of adoption of newer technology necessary to justify the intervention scenarios is considered to be the additional capital in all three scenarios.
The economy-wide effects of the intervention scenarios have been analyzed for land shift under the new intervention crop sector at 2.5, 5, and 10% of the total land coverage of the respective crops.
The SAM multiplier model thus developed has been applied to simulate under three distinct scenarios and the detail about them is given in Table 3 .
However, prior to solving the SAM multiplier model corresponding to various climate-smart agriculture scenarios, we solved that model without such interventions and considered it as a baseline scenario. Therefore, under the baseline scenario, farmers are following the traditional method of crop cultivation that includes puddled transplanated paddy and direct-seeded wheat cultivation.
On the other hand, in scenario 1; the practice of Conservation Agriculture (CA) has been applied to both Rice and Wheat sectors, under the practice of Direct Seeded Rice (DSR) and Zero Tillage (ZT) Wheat.
In scenario 2, the extensively studied practice of SRI, along with nascent emerging SWI has been undertaken.
In scenario 3, the recent drive towards Zero Budget Natural Farming (ZBNF) has been taken into consideration. India has set a target of achieving 10% of the cultivated area to be organic in nature by 2025 (GOI 2021a , b ). ZBNF technology, even after its differences with organic farming promotes chemical-free technologies as established above. In this scenario, 10% of the total rice and wheat cultivated area is assumed to be achieved through ZBNF. The input structure changes in each of the aforementioned scenarios are presented in Table 4 .
Further, scenario 4 has been considered where an overall shift in final demand from Rice and Wheat to alternative foodgrains such as Maize, Sorghum, and Millet has been considered. A modest 10% rice and wheat cultivation area is substituted with maize, sorghum, and millet sectors, which comparatively require the same or lesser input requirements in terms of fertilizer, labour, and water requirements. Additionally, in scenario 4 a comparison has been made of the multiplier effects and outcomes of the alternative foodgrains in relation to the traditional Rice and Wheat sectors. There are no changes in technological coefficients in this scenario but rather a comparison of the multiplier effects of the varying sectors.
In this section, the results from the intervention scenarios are discussed, where, the impact of technology-related scenarios is presented first, followed by the results from the alternative substitution scenario.
3.1 Economy-wide effects
3.1.1 technological intervention scenarios.
The aggregate effects, due to an increase in exogenous final demand for Rice and Wheat have been given in Table 5 . The multiplier effect or the economy-wide impact using the SAM model does not take into consideration the cost of adoption of these technologies in agricultural practices, but only the resulting effects in macroeconomic outcomes due to changes in key inputs into the sectors.
The results indicate the highest output, GDP, and income changes in the case of SRI-SWI. The associated higher yields are a major driver in case of SRI-SWI practice compared to the DSR-ZT and ZBNF scenarios (Table 5 ).
Furthermore, it is observed that the highest income is generated among the rural farming households and also the highest quintile among the urban households (Table 6 ). The additional GDP increase in scenario 1; DSR-ZT, is 2.40 billion due to Rice and 2.42 billion due to Wheat. Similarly in scenario 2; SRI-SWI, the GDP multiplier is 2.77 billion due to Rice and 2.75 due to Wheat. Lastly, we see for scenario 3; ZBNF, there is GDP multipliers are 2.75 billion for Rice and 2.58 for Wheat. Hence, ZBNF gives the second highest collective GDP increase along with the highest income and output multipliers among the three scenarios. These effects are directly associated with yield enhancement and labour changes in the three scenarios. Furthermore, the pertinent reason behind high output associated with SRI-SWI, could be also from increased requirements from machinery sector which further has several backward linkages in manufacturing sectors.
The simulated effects of the same final demand deliveries but the introduction of varying interventions in Rice and Wheat sector into the SAM were estimated, with a shift in the area for 2.5, 5, and 10, for three of the scenarios. The top five output changes occurred among the sectors represented in Table 6 . Sectoral output increase is transmitted primarily into sectors such as Raw Milk, Wholesale & Retail Trade, and Land Transport in all three scenarios. Sectors such as Raw Milk and Other Vegetables are closely linked to all crop sectors since most of the farming households also grow subsistence vegetable crops and possess cattle and livestock both for consumption and commercial purposes. Raw Milk is among the top sectors due to the presence of cattle among the RWCS farmers. Furthermore, wheat particularly is accounting for among the top backward linkages into the output of the Cattle and Raw Milk sector. The Wholesale & Retail Trade and Land Transport sectors are among the top sectors due to their strong backward linkages in both Rice and Wheat sectors as facilitators of the supply chain. Furthermore, in the case of both SRI-SWI and DSR-ZT, other manufacturing sectors and other business services are also among the top five sectors, which could be due to associated higher machinery requirements or higher cohesion with certain agro-processing and services sectors with an increase in agricultural output. Moreover, the presence of Cotton Yarn, among the top five sectors, in the case of SRI-SWI and ZBNF is primarily driven by the Wheat sector’s backward and forward linkages with the Other Small Ruminants and Other Livestock, which in turn have strong forward linkages with Raw Cotton sector and then that, in turn, being major input into Cotton Yarn (Table 9 in Appendix 3). Sectors such as land transport, wholesale and retail trade have close linkages with the Rice and Wheat cropping systems, during the post-harvest activities.
The fall in labour requirements that has been induced in the case of SRI-SWI should be accounted for with an eventual shift of labour to other sectors. Hence, enhancing the productivity of existing labour can be explored rather than considering additional labour requirements. Therefore, practices of DSR-ZT, even though are of modest enhancements yet are more aligned with the patterns of a shift in the economy. Since they require lesser labour input with a shift into the usage of additional tools and machinery, which might be possible if subsidies are introduced for farm machinery with effective adoption incentives for the farmers, especially those belonging to the small and marginal landholding groups. Also, it is observed that even though the yield increase is the highest in case of SRI-SWI, the economy-wide effects of it is weaker due to the lowering of labour requirements which could have been considered rather as a shift into any of the other sectors to provide the eventual transition paths for the economy.
3.1.2 Shift towards alternative crops
In the case of Scenario 4, where a shift in alternative cereals has been considered, the effects of additional unit final demand on the factor input requirements (Labour, Land, Capital) and the consequent aggregate multiplier effects (Output, GDP, Income) are presented in Table 7 . The associated output increase in the case of Maize is the highest followed by Rice, Sorghum & Millets, and Wheat. The income and GDP increase is highest for Rice followed by Sorghum & Millets, Wheat, and lastly, Maize.
Maize, apart from having the highest increase in output has also comparatively lower labour and capital requirements than the other foodgrains, wherein Wheat has the maximum factor input requirements. About 90% of the total labour requirements are being fulfilled by the rural labour group, across the varied foodgrain crops. Hence, in consequence, the household factor incomes are accounted for majorly by the rural farming households constituting half of the factor incomes generated. Furthermore, given that Rice and Wheat have higher area allocation and production, it is not surprising that associated income and GDP generation are also highest among those sectors.
But given the differences are quite marginal, a shift from the Rice–Wheat cropping system can be encouraged, given the associated benefits of labour and emission savings from the other cropping patterns.
3.2 Resource and emission footprints
The water use and emission intensities were calculated on the basis of direct and total GHG and water footprints of the crop sectors along with the primary input sectors such as fertilizer, petroleum, and electricity sector (Mishra et al. 2021 ). The direct water footprints of the crop sectors were taken as per Mekonnen and Hoekstra ( 2011 ) estimates, while the water footprints of primary input sectors were based on multiple studies (Vasudha 2022 ), (Pardikar 2019 ) (GOI 2022a , b , c ) (CSE 2019 ) (Sun et al. 2018 ) (GOI 2021a , b ). Similarly, the GHG footprints of the crop and the primary inputs were determined in reference to multiple studies (Kashyap and Agarwal 2021 ; Maheswarappa et al. 2011 ; Vetter et al. 2017 ). The respective direct and total footprints of various foodgrains have been presented in Figs. 3 and 4 . It is observed that across the sectors, the percentage shares are to a large extent equally distributed among the varied cereal crops. Wheat has the highest share among the direct footprints, this is a primary cause of our consideration of the blue water footprints. Even though as per Mekonnen and Hoekstra ( 2011 ), rice would actually be much more water intensive but the primary share of it is accounted from green water, which is reliant on rainfall. Furthermore, the blue water footprints give a better picture of the establishment of irrigation requirements.
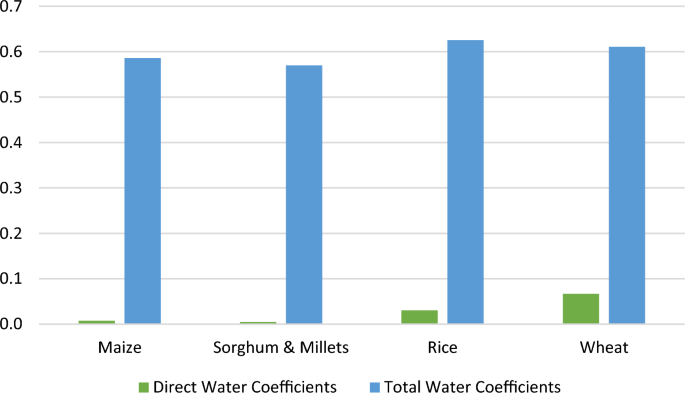
Direct and Total Blue Water Footprint for Cereals Sector (in BCM/Rs)
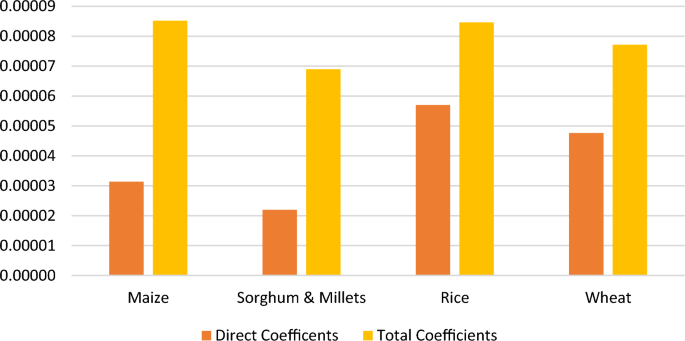
Direct and Total GHG Footprint of (CO2eq/Rs)
The Rice sector has higher water requirements; but since we have considered only the blue water usage, the wheat sector is dominating. Most of the rice fields are rainfed, while wheat is primarily grown on irrigated lands, hence accounting for a majority share. It is evident, that alternative foodgrains such as Maize and Sorghum & Millets, have lower direct water and carbon intensities, compared to Rice and Wheat sectors. Furthermore, Sorghum & Millets particularly have lower total GHG footprints indicating better suitability for substitution of both in terms of environmental sustainability and nutritional security.
The footprint estimation of the CSA practice interventions is presented in Figs. 5 and 6 .
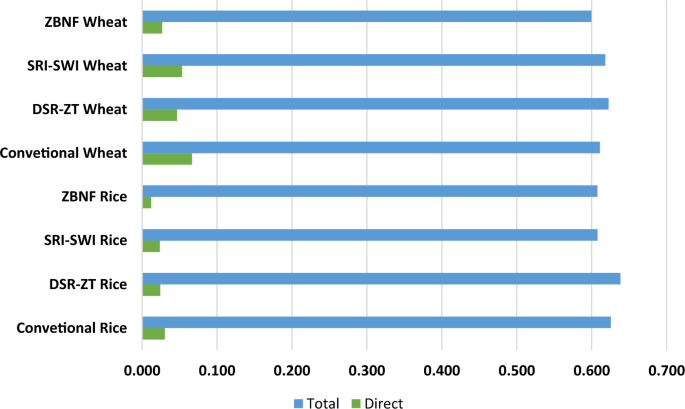
Blue Water Footprint among the Scenarios (in BCM/Rs)
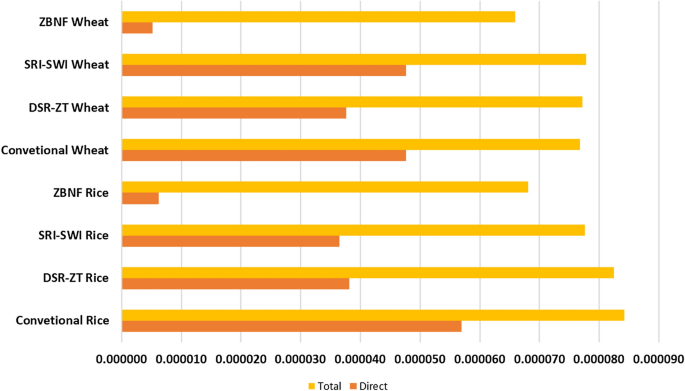
GHG footprint among the Scenarios (in CO2eq/Rs)
The total blue water footprints in the case of ZBNF Rice–Wheat and SRI-SWI Rice were found to be lesser compared to the conventional practice. Whereas, in case of DSR-ZT Rice–Wheat and SRI-SWI Wheat, the total blue water footprints are higher. Although, comprehensive water footprint accounting for each and every sector that has linkages with the RWCS may show significant differences. Yet, the use of an economy-wide framework for envisaging embedded resource footprints and savings is instrumental, even though the direct water requirements among the scenarios are lesser than the conventional practices, but not necessarily when accounted in terms of total water requirements. However, in the case of total carbon footprints, ZBNF Rice–Wheat has the highest reductions in terms of total GHG footprints compared to other scenarios. While, among other scenarios; the reduction in total GHG footprint in case of DSR-ZT Rice is minimal compared to conventional Rice, while being the same as conventional practice, in case of DSR-ZT Wheat. Furthermore, the essence of economy-wide footprints is revealed upon considering the marginal increase that is observed in the total carbon footprint from Wheat under SRI-SWI. Despite there being no consideration for the reduction of direct emissions in this scenario, the overall total footprint is higher than the conventional practices due to higher input demands.
4 Discussion
The results show that a significant difference is observed when it comes to input usage, household incomes, output, and emission intensities when there is the adoption of differing agroecological consonant CSA practices. The changes in income from varied adoption measures are especially relevant for rural farm households. The reductions in water and emissions certainly are of great importance in relevance to the adaptation and mitigation goal from the CSA framework, but reductions or savings in direct footprints need not always translate into similar outcomes upon consideration of total footprints which are primarily associated with sectoral linkages. Furthermore, in our study, all three intervention scenarios can be considered as example cases of blended sustainabilities between SAI and AI.
A balanced approach is required towards the implementation of such CSA interventions for it to be consonant with agroecology, over rigid enforcement of a certain set of guidelines. CA (DSR-ZT) and SRI-SWI in practice are not averse to mechanization or external outputs as long as the core principles of the intervention are understood in the context of their implementation.
ZBNF certainly provides higher environmental benefits when compared to CA (DSR-ZT) and SRI-SWI directly. Yet, the intertemporal limitations of the SAM framework are to be considered in this case, as the transition to a ZBNF scenario is of exigency but not of immediacy since such contrasting changes to the agricultural sector in practice require wider knowledge, dissemination, and adoption support. The negligence of such considerations may lead to a systemic economy-wide crisis. The case of Srilankan agricultural reforms is a prime example of such good-intentioned yet not-so-practical approaches to policy intervention. An abrupt shift to organics resulted in a drop in yields, which caused a surge in food prices that transmitted throughout the system causing marked inflation in the economy (Pandey 2021 ).
One of the main limitation of the current study has been the calculation of the economic benefits of water and GHG footprint through the existing SAM framework for water resources accounting for the costs associated with irrigation charges. Therefore, economic accounting of resource savings is certainly going to play a major role given the introduced scarcities due to climate change. Similarly, due to the lack of an environmentally-extended SAM accounts, the economic benefits of emissions reduction have not been considered in the present study. Furthermore, the agricultural sector in India is already considered to be stagnant and plagued by low labour productivity.
Hence, the considered ZBNF scenario with an increased labour requirement might not be plausible in terms of increased labour demands. Rather, with constrained labour, an increased productivity scenario has to be considered. The linkages found between the crop and livestock sectors are of importance since they fit the ZBNF criterion—greater livestock integration with farming practices. Meanwhile, CA (DSR-ZT) presents an ideal scenario, fitting the needs of the Indian economy which can be adopted given the suitability of context upon which the resulting labour reductions from the adoption of CA (DSR-ZT), can be absorbed in other productive sectors. Similarly, SRI-SWI also promises greater labour savings, yet as often found in literature, the initial period is certainly labour intensive, as the core essence of SRI-SWI is through effective knowledge transfer in the management of cropping cycles; while the increase in capital requirements can be addressed through appropriate policy measures at the initial stages of transition.
Likewise, scenario 4 which proposes a shift into alternative cereals, is also of greater priority in the Indian context. Apart from the factor requirement efficiencies, additional perspectives of nutrition, climate, and environment also align with the shift to coarse cereals (Davis et al. 2019 ). The water footprint efficiency presented by the alternative cereals was found to be significant with the given shift from Kharif to Rabi season for the cereals and is indicative of the shift from monsoon to irrigated farming practices. Hence, the pressure on the surface and groundwater has increased over the years (Kayatz et al. 2019 ). These developments come from the Green Revolution period in India, which resulted in a transition from coarse cereal production into primarily Rice–Wheat cropping system over large areas of production. These transformations were then considered of priority, towards achieving food security and self-sufficiency.
Achievement of food security has to be paralleled with nutritional security, which India is currently lacking. India is estimated to have 14% of its population undernourished, even though it ranks high among the largest producers of agricultural output. Several studies have been undertaken towards resolving this nutrition crisis. It has been found that even though the calorific value of coarse cereals might be at par or lesser than Rice and Wheat, yet they provide the required nutritional diversity (DeFries et al. 2018 ; Puranik et al. 2017 ). Also, the continuance of resource-intensive forms or Rice–Wheat cropping systems for higher yield without undue forbearance has also resulted in unsustainable resource and environmental pressures.
Hence, the current study has attempted towards comprehending the effects of such interventions which can fit the overall three-pillared objectives set under CSA, while originating from on-field experiments.
5 Conclusion and policy recommendations
In this study, we assessed the economy-wide impacts of three different agricultural intervention scenarios. The changes in farmer’s practices were considered in the first three scenarios, where the economy-wide effects of the adoption of CA (DSR-ZT), SRI-SWI, and ZBNF were considered. It was seen that while the adoption of the practices prescribed by the SRI-SWI movement provided the highest changes across the economy, ZBNF has the lowest water and GHG footprint compared to conventional practices. Yet, there are associated increased machinery requirements in the case of SRI-SWI and an entirely redesigned cropping pattern and outlook to agricultural practices in the case of ZBNF. In comparison, even though CA (DSR-ZT) has a lesser economic multiplier effect when compared to ZBNF and SRI-SWI, it has lesser labour requirements with additional capital investment requirements towards the funding of mechanized inputs but is relatively easy to adopt as found in literature when compared to SRI-SWI. SRI Footnote 5 being a knowledge-intensive innovation, the requirement of skilled manpower has been the major constraint, even after considerable institutional backing and civil society participation. Several state governments have made attempts at encouraging crop intensification on-farm experiments through extension programmes by the state agricultural universities and Krishi Vigyan Kendras (KVKs). Also, central institutions such as the National Bank For Agriculture And Rural Development (NABARD) have been partnering with NGOs towards the spread of SRI practices in states such as Andhra Pradesh, Assam, Bihar, Chhattisgarh, Jharkhand, Maharashtra, and Karnataka. Lastly, while ZBNF provides both economic and environmental benefits, the associated tradeoffs are again with the period of transition from one practice to the other. There are recommendations for a shift into alternative cropping patterns to foodgrains such as Maize, Sorghum, and millet, since economically they are having the same or enhanced income and output effects when compared with Wheat.
Furthermore, the recommendation towards the application of CA beyond the DSR-ZT scenario is certainly of relevance, when it comes to a shift to alternative cereals such as Maize, Sorghum, and Millets. A reversion in the trend towards the production of these coarse cereals themselves is not only limited to securing the nutritional security of the population but also has implications for environmental sustainability which can be further enhanced through CA-based cropping systems (Davis et al. 2019 ). This shall ensure retention of the lost trend to coarse cereal production, in the currently Rice–Wheat dominated cropping patterns of IGP hence, ensuring greater health benefits while addressing the climate change aspect. Furthermore, these cereals are to be brought under the coverage of the Public Distribution System (PDS) akin to those established for Rice and Wheat, to ensure the consumption benefits of these crops are accessible to the poorer sections of the society. Hence, a combined shift into CA-based Rice–Maize cropping pattern is quite plausible. Such a transition aligns with the goals of assuring nutritional security while reducing emission and resource consumption levels. Although, certainly this shift in production has to be accompanied with a shift in dietary preferences and the creation of healthier lifestyle choices.
The choice between CA (DSR-ZT), SRI-SWI, and ZBNF is context-specific depending on varied factors of adoption. Since these practices have certain core guidelines while also being flexible to differing regional factors, effective implementation requires closer region-specific research studies. At the central level, the Ministry of Agriculture and Farmer’s Welfare has initiated the promotion of natural farming, under the Bharatiya Prakritik Krishi Paddhati (BPKP). Yet, a well-integrated approach towards addressing the state of natural farming is still lacking, even among the state-led initiatives; since, most of the flagship programmes are currently at a small scale, lacking cohesive macro-level intervention. Furthermore, the extensive certification process is expensive and cumbersome for small farmers. Also, the lack of risk coverage and low yield support for the farmers during the transition phase from traditional agriculture, pose yet another set of challenges toward the widescale adoption of ZBNF practices (Khurana and Kumar 2020 ).
Policy support is needed for capacity building and training for CA practices, similar to the self-organized pedagogical drive that was witnessed in Karnataka during the inception phase of ZBNF. An entirely different set of barriers hinders the scalability of CA; which demands a particular need to shift from a narrow goal of food security to livelihood security of the farmers, from a policy perspective. Bhan and Behera ( 2014 ), suggest for a “Lead Farmer Approach” to achieve such an extension. Furthermore, CA systems are often quite complex, hence greater linkages within the farming and research community are required for the effective tailored implementation of CA. In terms of policy support, credit and subsidy measures can be considered, along with higher support in terms of accessibility to CA equipment and maintenance. The practice of CA in India was primarily initiated in a mechanized form but that doesn’t associate strictly with conventional industrialized practices. Hence, greater AE norms are to be envisioned in carrying forth due development in the future of CA practices.
The framework of objectives set by the CSA approach has brought forth a resurgence in the discussions pertaining to food security in the advent of climate change. Yet, the practices that are being introduced under CSA have to be broadened from the three-pronged goals and need to take into account aspects of long-term productivity, environmental sustainability, agroecological biodiversity, food sovereignty, and national security. This is where the distinctions between the blended differences of SAI and AEI are important. Thus, the cohesion between the objectives of CSA and the practices identified under AE holds relevance towards striking a balance between multidimensional aspects of agriculture. Furthermore, an economy-wide framework is certainly of great significance in encompassing the linkage effects of varying interventions and their effects on various sectors and household groups. But, for future studies, inter-regional SAM might be of great use, which is region specific and is considerate of the agroecological variations.
Availability of data and materials
The datasets generated during and/or analyzed during the current study are available in the Harvard dataverse repository, [ https://doi.org/ https://doi.org/10.7910/DVN/NYVJX8]44 .]
This move was also in response to the introduction of improved variety of dwarf wheat cultivars of Sonara 64 and Lerma Rojo which led to a late sowing period for wheat.
Developed in 1980s by French Agronomist and Jesuit Father Henri de Laulanié, with his core motivation being towards instilling a sense of self-reliance by addressing the varied resource constraints of the peasant farmers of Madagascar (Prasad C. S., 2006; de Laulanié, 1993 ).
Since it is primarily a management-oriented innovation, the fluctuations in yields and resources are reliant on knowledge adapatation in practice during the transition phase. Therefore, even though there might be immediate increases in per kg labour requirements in the short term, the new methods and practices is witnessed to be labour saving (Thakur & Uphoff 2017 ).
It started under the extension projects in 2002–03, by the state agricultural universities of the two states of Tamil Nadu and Andhra Pradesh. Tamil Nadu Agricultural University initiated experiments with a modified SRI practice, that used three of the SRI principles of single seeding, wider spacing and use of weeder. It was found, that even though there wasn’t initially any differences in yield but significant savings in water usage was witnessed (Thiyagarajan 2002 ).
As Prasad ( 2006 ) notes in his book, the evolution of SRI in India can be traced in two parallels; firstly, through developments in on-station experiments of research and extension departments, primarily in the southern states and secondly, in the narrative of SRI as a civil society innovation through activities of civil society organisations and NGOs such as PRADAN (Professional Action Development Action Network) and TATA Trusts.
Abbreviations
Agroecology
Agroecological intensification
Billion cubic meters
Climate smart agriculture
- Conservation agriculture
Commodities
Direct seeded rice-zero tillage
Factor income
Greenhouse gases
Global warming potential
Krishi vigyan kendras
International Food Policy Research Institute
Indo-gangetic plains
Public distribution system
Rice wheat cropping system
- Social accounting matrix
System of wheat intensification
System of rice intensification
Sustainable agricultural intensification
Trade and transport margins
- Zero budget natural farming
Abraham B, Araya H, Berhe T, Edwards S, Gujja B, Khadka RB, Verma A (2014) The system of crop intensification: reports from the field on improving agricultural production, food security, and resilience to climate change for multiple crops. Agric Food Secur 3(1):1–12. https://doi.org/10.1186/2048-7010-3-4
Article Google Scholar
Abrol, I. P., Gupta, R. K., & Malik, R. K. (2005). Conservation agriculture: status and prospects. Centre for Advancement of Sustainable Agriculture. Retrieved from https://www.worldcat.org/title/conservation-agriculture-status-and-prospects/oclc/607659932
Aune JB (2012) Conventional, organic and conservation agriculture: production and environmental impact. In: Lichtfouse E (ed) Agroecology and strategies for climate change. Springer, Dordecht
Google Scholar
Barrett CB, Moser CM, McHugh OV, Barison J (2004) Better technology, better plots, or better farmers? Identifying changes in productivity and risk among Malagasy rice farmers. Am J Agr Econ 86(4):869–888
Bhan S, Behera UK (2014) Conservation agriculture in India-problems, prospects and policy issues. Int Soil Water Conserv Res 2(4):1–12
Bharucha ZP, Mitjans SB, Pretty J (2020) Towards redesign at scale through zero budget natural farming in Andhra Pradesh, India. Int J Agric Sustain 18(1):1–20. https://doi.org/10.1080/14735903.2019.1694465
Bhatia A, Aggarwal PK, Jain N, Pathak H (2012) Greenhouse gas emission from rice- and wheat-growing areas in India: spatial analysis and upscaling. Greenh Gases: Sci Technol 2(2):115–125. https://doi.org/10.1002/ghg.1272
Bhattacharyya R, Das TK, Sudhishri S, Dudwal B, Sharma AR, Bhatia A, Singh G (2015) Conservation agriculture effects on soil organic carbon accumulation and crop productivity under a rice–wheat cropping system in the western Indo-Gangetic Plains. Eur J Agron 70(70):11–21. https://doi.org/10.1016/j.eja.2015.06.006
Birthal PS, Khan T, Negi DS, Agarwal S (2014) Impact of climate change on yields of major food crops in India: implications for food security. Agric Econ Res Rev 27(2):145–155
Biswas S (2020) Zero budget natural farming in India: aiming back to the basics. Int J Environ Clim Change 10(9):38–52. https://doi.org/10.9734/IJECC/2020/v10i930228
Biswas S, Das R (2021) System of wheat intensification (SWI). Agriculture & Food: E-Newsletter, Goundam
Brander, M., & Davis, G. (2012). Greenhouse gases, CO2, CO2e, and carbon: What do all these terms mean. Econometrica, White Papers. Retrieved from https://ecometrica.com/assets/GHGs-CO2-CO2e-and-Carbon-What-Do-These-Mean-v2.1.pdf
Breisinger C, Thomas M, Thurlow J (2009) Social accounting matrices and multiplier analysis: an introduction with exercises. International Food Policy Research Institute (IFPRI), Washington DC
Calzadilla A, Rehdanz K, Betts R, Falloon P, Wiltshire A, Tol RS (2013) Climate change impacts on global agriculture. Clim Change 120(1):357–374. https://doi.org/10.1007/s10584-013-0822-4
CEA (2023) All India electricity statistics. General review 2023. Central Electricity Authory, Ministry of Power, Government of India, New Delhi. https://cea.nic.in/wp-content/uploads/general/2022/GR_Final.pdf
CEIC. (2021). India Electricity: Consumption: Utilities: Agriculture. Retrieved from CEIC: https://www.ceicdata.com/en/india/electricity-consumption-utilities/electricity-consumption-utilities-agriculture#:~:text=India%20Electricity%3A%20Consumption%3A%20Utilities%3A%20Agriculture%20data%20was%20reported,at%20215%2C000.000%20GWh%20in%202021 .
CSE (2019) Fertilizer—sector performance. Centre for Science and Environment, New Delhi
Davis KF, Chhatre A, Rao ND, Singh D, Ghosh-Jerath S, Mridul A, DeFries R (2019) Assessing the sustainability of post-green revolution cereals in India. Proc Natl Acad Sci 116(50):25034–25041. https://doi.org/10.1073/pnas.1910935116
DeFries R, Chhatre A, Davis KF, Dutta A, Fanzo J, Ghosh-Jerath S, Smith MR (2018) Impact of historical changes in coarse cereals consumption in India on micronutrient intake and anemia prevalence. Food Nutr Bull 39(3):377–392. https://doi.org/10.1177/2F0379572118783492
Dhar S, Barah BC, Vyas AK, Uphoff N (2016) Comparing system of wheat intensification (SWI) with standard recommended practices in the northwestern plain zone of India. Arch Agron Soil Sci 62(7):994–1006. https://doi.org/10.1080/03650340.2015.1101518
Dhawan V (2017) Water and agriculture in India. German Asia-Pacific Business Association, Hamburg. https://www.oav.de/fileadmin/user_upload/5_Publikationen/5_Studien/170118_Study_Water_Agriculture_India.pdf
FAO. (2009). Food Security and Agricultural Mitigation in Developing Countries: Options for Capturing Synergies. Rome: Food and Agriculture Organization of the United Nations. Retrieved from https://www.fao.org/agrifood-economics/publications/detail/en/c/122847/
FAO. (2018). Climate-Smart Agriculture Case Studies 2018, Successful approaches from different regions. Rome: Food and Agriculture Organization of the United Nations. Retrieved from https://www.fao.org/3/CA2386EN/ca2386en.pdf
FAO. (2020). FAOSTAT Emissions Database. Retrieved from Food and Agriculture Organization of the United Nations (FAO): http://www.fao.org/faostat/en/#data/GT
FAO. (2020). The state of food security and nutrition in the world 2020. Retrieved from Food and Agriculture Organization of the United Nations: https://www.fao.org/3/ca9692en/online/ca9692en.html#chapter-Key_message
FAOSTAT. (2022). Food and Agriculture Organization of the United Nations (FAO). Retrieved from FAOSTAT Database: https://www.fao.org/faostat/
Fischer G, Shah MN, Tubiello F, Van Velhuizen H (2005) Socio-economic and climate change impacts on agriculture: an integrated assessment, 1990–2080. Phil Trans Royal Soc B Biol Sci 360(1463):2067–2083. https://doi.org/10.1098/rstb.2005.1744
Ghosh M (2019) Climate-smart agriculture, productivity and food security in India. J Dev Policy Pract 4(2):166–187. https://doi.org/10.1098/rstb.2005.1744
GOI (2016) Indian organic sector vision 2025. Government of India, New Delhi
GOI (2021) Indian petroleum and natural gas statistics 2020–21. Ministry of Petroleum and Natural Gas, Government of India, New Delhi
GOI (2022) International year of millets (IYoM)-2023. Ministry of Agriculture & Farmers Welfare, Government of India, New Delhi
GOI. (2021). Report of Task Force on Organic and Non-Chemical Farming. Recommendations on strategy to promote Organic and non-chemical farming in India. New Delhi: Department of Agriculture, Cooperation & Farmers Welfare, Ministry of Agriculture & Farmers Welfare, Government of India. Retrieved from http://ums.rvskvv.net/TaskForce/D_R_B.pdf
GOI. (2022). Agricultural Statistics at a glance 2021. New Delhi: Ministry of Agriculture & Farmers Welfare, Government of India. Retrieved from https://eands.dacnet.nic.in/PDF/Agricultural%20Statistics%20at%20a%20Glance%20-%202021%20(English%20version).pdf
GOI. (2022). Urea Pricing Policy Section. Retrieved from Department of Fertilizers, Government of India: https://fert.nic.in/urea-pricing-policy-section#:~:text=The%20MRP%20of%20urea%20is,%2FPSUs%2FCooperatives%20and%20Rs .
GOI. (2020). Agricultural Statistics at a Glance 2019. New Delhi: Government of India, Ministry of Agriculture and Farmers Welfare, Department of Agriculture, Cooperation and Farmers Welfare. Retrieved from https://eands.dacnet.nic.in/PDF/At%20a%20Glance%202019%20Eng.pdf
Gupta N, Pradhan S, Jain A, Patel N. (2021). Sustainable Agriculture in India 2021 – What we know and how to scale up. New Delhi: Council on Energy, Environment and Water. Retrieved from https://www.ceew.in/sites/default/files/CEEW-Sustainable-Agricultre-in-India-2021-ES-21May21.pdf
Hashim SR. (1971). Inter-linkages and the changes in the pattern of commodity flows in India: 1950–51 to 1959–60. Indian Economic Journal, 19(2), 187. Retrieved from https://www.proquest.com/openview/cf6c51eff7872e3d8a0f9398dba2eb7e/1?pq-origsite=gscholar&cbl=1819379
Havinga IC, Sarmad K, Hussain F, Badar G, Mohammad F (1987) A social accounting matrix for the agricultural sector of Pakistan [with Comments]. Pak Dev Rev 26(4):627–641
Hayden C, Round JI (1982) Developments in social accounting methods as applied to the analysis of income distribution and employment issues. World Dev 10(6):451–465. https://doi.org/10.1016/0305-750X(82)90002-X
Hazari BR (1970) Empirical identification of key sectors in the Indian economy. Rev Econ Stat 52(3):301–305. https://doi.org/10.2307/1926298
Heilig GK (1994) The greenhouse gas methane (CH 4): sources and sinks, the impact of population growth, possible interventions. Popul Environ 16(2):109–137. https://doi.org/10.1007/BF02208779
Hill, S. (1985). Redesigning the food system for sustainability. Alternatives: Global, Local, Political, 12(3), 32–36. Retrieved from https://www.researchgate.net/publication/285538508_Redesigning_the_food_system_for_sustainability
Hobbs PR, Sayre K, Gupta R (2008) The role of conservation agriculture in sustainable agriculture. Phil Trans Royal Soc B Biol Sci 363(1491):543–555. https://doi.org/10.1098/rstb.2007.2169
Hobbs P, Gupta R, Jat RK, Malik RK (2019) Conservation agriculture in the indogangetic plains of India: past, present and future. Exp Agric 55(2):339–357. https://doi.org/10.1017/S0014479717000424
IFPRI. (2020). Social Accounting Matrix for India 2017/18. doi: https://doi.org/10.7910/DVN/NYVJX8
IPCC (2013) Summary for policymakers. In: Stocker TF, Qin D, Plattner G-K, Tignor MM, Allen SK, Boschung J, Midgley PM (eds) Climate change 2013. The physical science basis. Summary for policymakers, technical summary and frequently asked questions. Intergovernmental Panel of Climate Change, Geneva
Jackson, M. T. (2009). Revitalizing the Rice-Wheat Cropping Systems of the Indo-Gangetic Plains: Adaptation and Adoption of Resource-Conserving Technologies in India, Bangladesh, and Nepal. Final Report. IRRI Ref. No. DPPC2007–100. Retrieved from https://pdf.usaid.gov/pdf_docs/Pdacp630.pdf
Jain N, Dubey R, Dubey DS, Khanna M, Pathak H, Bhatia A (2014) Mitigation of greenhouse gas emission with system of rice intensification in the Indo-Gangetic Plains. Paddy Water Environ, 12(3):355–363. https://doi.org/10.1007/s10333-013-0390-2
Jat ML, Singh B, Gerard B (2014) Nutrient management and Use efficiency in wheat systems of South Asia. Adv Agron 125:171–259. https://doi.org/10.1016/B978-0-12-800137-0.00005-4
Jat ML, Chakraborty D, Ladha JK, Rana DS, Gathala MK, McDonald A, Gerard B (2020) Conservation agriculture for sustainable intensification in South Asia. Nat Sustain 3(4):336–343. https://doi.org/10.1038/s41893-020-0500-2
Jovial N (2022) India uses more groundwater than US and China combined. The Week. https://www.theweek.in/theweek/specials/2022/08/13/india-uses-more-groundwater-than-us-and-china-combined.html
Kapil, S. (2021). Agri share in GDP hit 20% after 17 years: Economic Survey. Retrieved from Down to Earth: https://www.downtoearth.org.in/news/agriculture/agri-share-in-gdp-hit-20-after-17-years-economic-survey-75271
Kashyap D, Agarwal T (2020) Food loss in India: water footprint, land footprint and GHG emissions. Environ Dev Sustain 22(4):2905–2918. https://doi.org/10.1007/s10668-019-00325-4
Kashyap D, Agarwal T (2021) Carbon footprint and water footprint of rice and wheat production in Punjab, India. Agric Syst. https://doi.org/10.1016/j.agsy.2020.102959
Kassam A, Friedrich T, Derpsch R, Kienzle J. (2015). Overview of the worldwide spread of conservation agriculture. Field Actions Science Reports The Journal of Field Actions, 8. Retrieved from https://journals.openedition.org/factsreports/3966
Kayatz B, Harris F, Hillier J, Adhya T, Dalin C, Nayak D, Dangour AD (2019) “More crop per drop”: exploring India’s cereal water use since 2005. Sci Total Environ 673:207–217. https://doi.org/10.1016/j.scitotenv.2019.03.304
Khadse A, Rosset PM, Morales H, Ferguson BG (2018) Taking agroecology to scale: the zero budget natural farming peasant movement in Karnataka, India. J Peasant Stud 45(1):192–219. https://doi.org/10.1080/03066150.2016.1276450
Khan HA, Thorbecke E (1989) Macroeconomic effects of technology choice: multiplier and structural path analysis within a SAM framework. J Policy Model 11(1):131–156
Khurana, A., & Kumar, V. (2020). State of Organic and Natural Farming: Challenges and Possibilities. New Delhi: Centre for Science and Environment (CSE). Retrieved from https://cdn.cseindia.org/webinar/State-Organic-amit.pdf
Korav S, Dhaka AK, Chaudhury A, M S (2020) Review- zero budget natural farming a key to sustainable agriculture: challenges, opportunities and policy intervention. Indian J Pure Appl Biosci 8(3):285–295. https://doi.org/10.1878/2582-2845.8091
De Laulanié, H. (1993). Le système de riziculture intensive malgache. Tropicultura, 11(3), 110–114. Retrieved from http://www.tropicultura.org/text/v11n3/110.pdf
Laxmi, V., Erenstein, O., & Gupta, R. K. (2007). Impact of zero tillage in India's rice-wheat systems. International Maize and Wheat Improvement Center. Retrieved from https://www.researchgate.net/publication/46471795_Impact_of_Zero_Tillage_in_India's_Rice-Wheat_Systems
Lewis BD, Thorbecke E (1992) District-level economic linkages in Kenya: evidence based on a small regional social accounting matrix. World Dev 20(6):881–897
Linquist BA, Adviento-Borbo MA, Pittlekow CM, van Kessel C, van Groenigen KJ (2012) Fertilizer management practices and greenhouse gas emissions from rice systems: a quantitative review and analysis. Field Crop Res 135:10–21. https://doi.org/10.1016/j.fcr.2012.06.007
Lipper L, Zilberman D (2018) A short history of the evolution of the climate smart agriculture approach and its links to climate change and sustainable agriculture debates. In: Lipper L, McCarthy N, Zilberman D, Branca AS (eds) Climate smart agriculture: building resilience to climate change. Springer, Cham
Chapter Google Scholar
Maheswarappa HP, Srinivasan V, Lal R (2011) Carbon footprint and sustainability of agricultural production systems in India. J Crop Improv 25(4):303–322. https://doi.org/10.1080/15427520902969906
Mall RK, Singh R, Gupta A, Srinivasan G, Rathore LS (2006) Impact of climate change on Indian agriculture: a review. Clim Change 78(2):445–478. https://doi.org/10.1007/s10584-005-9042-x
Mekonnen MM, Hoekstra AY. (2011). National water footprint accounts: The green, blue and grey water footprint of production and consumption. Delft, Netherlands: Daugherty Water for Food Global Institute. Retrieved from https://digitalcommons.unl.edu/wffdocs/85/
Mendelsohn R (2008) The impact of climate change on agriculture in developing countries. J Natl Resour Policy Res 1(1):5–19. https://doi.org/10.1080/19390450802495882
Michler JD, Baylis K, Arends-Kuenning M, Mazvimavi K (2019) Conservation agriculture and climate resilience. J Environ Econ Manag 93:148–169. https://doi.org/10.1016/j.jeem.2018.11.008
Miller RE, Blair P (2009) Input-output analysis: foundations and extensions. Cambridge University Press, Cambridge
Book Google Scholar
Mishra JS, Poonia SP, Kumar R, Dubey R, Kumar V, Mondal S, Bhaskar S (2021) An impact of agronomic practices of sustainable rice-wheat crop intensification on food security, economic adaptability, and environmental mitigation across eastern Indo-Gangetic Plains. Fields Crops Res. https://doi.org/10.1016/2Fj.fcr.2021.108164
Mizik T (2021) Climate-smart agriculture on small-scale farms: a systematic literature review. Agronomy 11(6):1096. https://doi.org/10.3390/agronomy11061096
Mockshell J, Kamanda J (2018) Beyond the agroecological and sustainable agricultural intensification debate: is blended sustainability the way forward? Int J Agric Sustain 16(2):127–149. https://doi.org/10.1080/14735903.2018.1448047
Mythili G, Harak N (2012) Sectoral linkages, multipliers, and the role of agriculture. In: Dev SM (ed) India development report 2012–13. Oxford University Press, Oxford
Nayar, V., Ravichandran, V. K., Barah, B. C., & Uphoff, N. (2020). Sustainable SRI and rice production: Learnings from an irrigated agriculture management project in Tamil Nadu. Economic and Political Weekly, 55(2), 46–51. Retrieved from https://www.epw.in/journal/2020/2/special-articles/sustainable-sri-and-rice-production.html
Nirmala B, Tuti MD, Mahender Kumar R, Waris A, Muthuraman P, Parmar B, Vidhan Singh T (2021) Integrated assessment of system of rice intensification vs. conventional method of transplanting for economic benefit, energy efficiency and lower global warming potential in India. Agroecol Sustain Food Syst 45(5):745–766. https://doi.org/10.1080/21683565.2020.1868648
Pal BD, Pradesha A, Thurlow J (2020) 2017/18 Social Accounting Matrix for India. A Nexus Project SAM. International Food Policy Research Instittute, Washington DC. https://doi.org/10.2499/p15738coll2.134178
Palekar S. (2005). The philosophy of spiritual farming I, 2nd ed. Amravati, Maharashtra, India: Amravati: Zero Budget Natural Farming Research, Development & Extension Movement.
Palekar S. (2006). The principles of spiritual farming II. Amravati, Maharashtra, India: Zero Budget Natural Farming Research, Development & Extension Movement.
Pandey S. (2021). How Sri Lanka’s overnight flip to total organic farming has led to an economic disaster. Retrieved from The Print: https://theprint.in/world/how-sri-lankas-overnight-flip-to-total-organic-farming-has-led-to-an-economic-disaster/728414/
Pardikar, R. (2019). Thermal power plants in India using more water than permitted limit: RTI. Retrieved from Business Standard: https://www.business-standard.com/article/current-affairs/thermal-power-plants-in-india-using-more-water-than-permitted-limit-rti-119091300136_1.html
Pathak H, Aggarwal PK (2012) Low carbon technologies for agriculture: a study on rice and wheat systems in the Indo-Gangetic Plains. Indian Agricultural Research Institute, New Delhi
Pathak H, Bhatia A, Jain N (2014) Greenhouse gas emission from Indian agriculture: trends, mitigation and policy needs. Indian Agricultural Research Institute, New Delhi
Perman, R., Ma, Y., Common, M., Maddison, D., & McGilvray, J. (2011). Natural Resource and Environmental Economics (4th). Pearson. Retrieved from https://www.pearson.com/uk/educators/higher-education-educators/program/Perman-Natural-Resource-and-Environmental-Economics-4th-Edition/PGM848461.html
PIB. (2015). Fact Sheet on Agriculture Demand Side Management (AgDSM). Retrieved from Press Information Bureau, Ministry of Power, Government of India: https://pib.gov.in/newsite/PrintRelease.aspx?relid=128572
Pradan. (2008). Promoting SWI in the Mountain Farms. NewsReach. Retrieved from https://www.pradan.net/images/Media/sept_09.pdf
Pradhan, B., & Sahoo, A. (1996). Poverty in a linear modelling framework under alternative market regimes: a case study of rural India. Journal of Development Economics. Retrieved from https://idl-bnc-idrc.dspacedirect.org/bitstream/handle/10625/32519/117380.pdf?sequence=1
Prasad CS (2006) System of rice intensification in India; innovation history and institutional challenges. WWF International—ICRISAT Dialogue Project, Hyderabad
Prasad R, Nagarajan S (2004) Rice–wheat cropping system–food security and sustainability. Curr Sci 87(10):1334–1335
Praveen D, Ramachandran A (2020) The current policies and practices behind scaling up climate-smart agriculture in India. In: Venkatramanan V, Shah S, Prasad R (eds) Global climate change: resilient and smart agriculture. Springer, Singapore
Puranik S, Kam J, Sahu PP, Yadav R, Srivastava RK, Ojulong H, Yadav R (2017) Harnessing finger millet to combat calcium deficiency in humans: challenges and prospects. Front Plant Sci. https://doi.org/10.3389/2Ffpls.2017.01311
Pyatt G (1988) A SAM approach to modelling. J Policy Model 10(3):327–352
Pyatt G, Round JI (1977) Social accounding matrices for development planning. Rev Income Wealth 23(4):339–364. https://doi.org/10.1111/j.1475-4991.1977.tb00022.x
Pyatt G, Round JI (1979) Accounting and fixed price multipliers in a SAM framework. Econ J 89(356):850–873
Pyatt G, Round J (1985) Social accounting matrices: a basis for planning. World Bank, Washington DC
Rana L, Banerjee H, Ray K, Sarkar S (2017) System of wheat intensification (SWI)–a new approach for increasing wheat yield in small holder farming system. J Appl Natl Sci 9(3):1453–1464
Reay DS, Davidson EA, Smith KA, Smith P, Melillo JM, Dentener F, Crutzen PJ (2012) Global agriculture and nitrous oxide emissions. Nat Clim Chang 2(6):410–416. https://doi.org/10.1038/nclimate1458
Ritchie, H., & Roser, M. (2020). CO 2 and Greenhouse Gas Emissions. Retrieved from Our World in Data: https://ourworldindata.org/ghg-emissions-by-sector
Roland-Holst DW, Sancho F (1995) Modelling prices in a SAM structure. Rev Econ Stat 77(2):361–371
Round J (2003) Social accounting matrices and SAM-based multiplier analysis. In: Bourguignon F, Pereira da Silva L (eds) Techniques and tools for evaluating the poverty impact of economic policies. World Bank and Oxford University Press, Oxford
Sah D, Devakumar AS (2018) The carbon footprint of agricultural crop cultivation in India. Carbon Manag 9(3):213–225. https://doi.org/10.1080/17583004.2018.1457908
Saj S, Torquebiau E, Hainzelin E, Pages J, Maraux F (2017) The way forward: an agroecological perspective for climate-smart agriculture. Agr Ecosyst Environ 250:20–24. https://doi.org/10.1016/j.agee.2017.09.003
Sarkar H, Subbarao SV (1981) Social accounting matrix for India for 1980–81. National Council of Applied Economic Research, New Delhi
Saxena A, Upadhyay T, Rai D, Varshney AC, Saxena M (2019) System of wheat intensification study using hydroponically raised wheat seedling–a new approach towards farmer’s welfare. Int J Adv Agric Sci Technol 6(1):24–40
Sen S (2018) If 80% water consumption in India is for agriculture, why is it unregulated and inefficient? Observer Research Foundation. http://20.244.136.131/expert-speak/if-80-water-consumption-in-india-is-for-agriculture-why-is-it-unregulated-and-inefficient
Shahbandeh, M. (2022). Leading countries based on the production of milled rice in 2020/2021 (in million metric tons). Retrieved from Statista: https://www.statista.com/statistics/255945/top-countries-of-destination-for-us-rice-exports-2011/
Sun P, Elgowainy A, Wang M, Han J, Henderson RJ (2018) Estimation of U.S. refinery water consumption and allocation to refinery products. Fuel 221:542–557. https://doi.org/10.1016/j.fuel.2017.07.089
Suresh NS, Ravuri S, Bose A, Haritha H, Shanker A, Lakshmi PA (2020) Life Cycle Assessment of ZBNF and Non-ZBNF. A preliminary study in Andhra Pradesh. Centre for Study of Science, Technology and Policy, Bengaluru. https://apcnf.in/wp-content/uploads/2020/05/LIFE-CYCLE-ASSESSMENT-OF-ZBNF-AND-NON-ZBNF-A-STUDY-IN-ANDHRA-PRADESH.pdf
Thakur AK, Uphoff NT (2017) How the system of rice intensification can contribute to climate-smart agriculture. Agron J 109(4):1163–1182. https://doi.org/10.2134/agronj2016.03.0162
Thiyagarajan, T. M. (2002). Experiments with a Modified System of Rice Intensification in India. Assessments of the System of Rice Intensification (SRI): Proceedings of an International Conference held in Sanya. (pp. 137–139). Retrieved from http://sri.ciifad.cornell.edu/proc1/sri_30.pdf
Timsina J, Connor DJ (2001) Productivity and management of rice-wheat cropping systems: issues and challenges. Field Crop Res 69(2):93–132. https://doi.org/10.1016/S0378-4290(00)00143-X
Tirol-Padre A, Rai M, Kumar V, Gathala M, Sharma PC, Sharma S, Ladha J (2016) Quantifying changes to the global warming potential of rice wheat systems with the adoption of conservation agriculture in northwestern India. Agric Ecosyst Environ 219(219):125–137. https://doi.org/10.1016/j.agee.2015.12.020
Townsend RF, McDonald S (1998) Biased policies, agriculture and income distribution in South Africa: a social accounting matrix approach. J Stud Econ Econom. https://doi.org/10.1080/03796205.1998.12129119
UNFCCC (2021) India: third biennial report to the United Nations framework convention on climate change. United Nations Framework Convention on Climate Change, Bonn
Vasudha. (2022). Vasudha Power info hub. Retrieved from Vasudha Power: https://vasudhapower.in/
Vetter SH, Sapkota TB, Hillier J, Stirling CM, Macdiarmid JI, Aleksandrowicz L et al (2017) Greenhouse gas emissions from agricultural food production to supply Indian diets: Implications for climate change mitigation. Agric Ecosyst Environ 237:234–241. https://doi.org/10.1016/j.agee.2016.12.024
Wezel A, Soboksa G, McClelland S, Delespesse F, Boissau A (2015) The blurred boundaries of ecological, sustainable, and agroecological intensification: a review. Agron Sustain Dev 35(4):1283–1295. https://doi.org/10.1007/s13593-015-0333-y
Wheeler T, Von Braun J (2013) Climate change impacts on global food security. Science 341(6145):508–513. https://doi.org/10.1126/science.1239402
World Bank. (2022). Fertilizer consumption (kilograms per hectare of arable land) - India, World. Retrieved from The World Bank Group: https://data.worldbank.org/indicator/AG.CON.FERT.ZS?locations=IN-1W
Download references
Acknowledgements
We would like to express our gratitude to the participants of the 28th International Input Output Association conference, Langkawi,, Malaysia, (28 Aug–02 Sept, 2022) for their constructive comments and suggestions. Our sincere thanks to all the anonymous reviewers for their valuable comments and feedback.
This research did not receive any specific grant from funding agencies in the public, commercial, or not-for-profit sectors.
Author information
Authors and affiliations.
Gokhale Institute of Politics and Economics, Pune, India
Ananya Ajatasatru, Vishnu Prabhu & Kakali Mukhopadhyay
International Food Policy Research Institute (IFPRI), New Delhi, India
Barun Deb Pal
Department of Natural Resource Sciences, Agricultural Economics Program, McGill University, Macdonald Campus, 21,111 Lakeshore Road, Ste Anne de Bellevue, Montreal, QC, H9X3V9, Canada
Kakali Mukhopadhyay
You can also search for this author in PubMed Google Scholar
Contributions
All authors contributed to the study conception and design, material preparation, data collection, and analysis. All authors read and approved the final manuscript.
Corresponding author
Correspondence to Kakali Mukhopadhyay .
Ethics declarations
Competing interests.
The authors declare that they have no competing interests.
Additional information
Publisher's note.
Springer Nature remains neutral with regard to jurisdictional claims in published maps and institutional affiliations.
Agricultural methane arises majorly from livestock rearing through enteric fermentation and through, anaerobic decomposition from water-intensive paddy fields that follow the traditional continuous submergence practice of rice cultivation (Heilig 1994 ; Bhatia et al. 2012 ). Nitrous oxide is produced naturally from the microbes present in the soil, but with increase in usage of nitrogenous fertilizer application there is an excess for microbial conversion (Reay et al. 2012 ). The potency of these emissions is calculated in terms of CO 2 equivalent (CO 2 eq) or global warming potential (GWP), which is an index with CO 2 as base that indicates the number of times more warming the other GHGs cause over a given time horizon (generally taken to be 100 years); The Global Warming Potential (GWP) of CH 4 and N 2 O are 25 and 298 times higher, than that of CO 2 (Brander and Davis 2012 ).
2.1 Social accounting matrix of India 2017–18
The 2017–18 SAM created by the International Food Policy Research Institute (IFPRI), constitutes 112 sectors composed of 39 sectors belonging to agriculture and allied activities, 18 sectors relating to agriculture-based processing activities, 24 manufacturing sectors, 4 mining sectors, 3 utilities sector, 1 construction sector, and 23 service sectors. The factors’ inputs are classified into labor, capital, and land. The labor has been classified into rural and urban, with the further division based on education level. Capital has been divided into 4 types; crop, live animal, mining, and other financial capital. Household has been divided into rural farm households, rural non-farm households, and urban households (Pal et al. 2020 ). The SAM entries show transaction flows among the sectors, where the columns indicate the expenditures, while the rows represent the receiving sectors, respectively. Furthermore, among the SAM sectors, the division of sectors is based on the activity and commodity of the respective sectors. Additional two sectors were split towards introducing interventions in rice and wheat crops.
See Table 8
See table 9, rights and permissions.
Open Access This article is licensed under a Creative Commons Attribution 4.0 International License, which permits use, sharing, adaptation, distribution and reproduction in any medium or format, as long as you give appropriate credit to the original author(s) and the source, provide a link to the Creative Commons licence, and indicate if changes were made. The images or other third party material in this article are included in the article's Creative Commons licence, unless indicated otherwise in a credit line to the material. If material is not included in the article's Creative Commons licence and your intended use is not permitted by statutory regulation or exceeds the permitted use, you will need to obtain permission directly from the copyright holder. To view a copy of this licence, visit http://creativecommons.org/licenses/by/4.0/ .
Reprints and permissions
About this article
Cite this article.
Ajatasatru, A., Prabhu, V., Pal, B.D. et al. Economy-wide impact of climate smart agriculture in India: a SAM framework. Economic Structures 13 , 4 (2024). https://doi.org/10.1186/s40008-023-00320-z
Download citation
Received : 11 September 2022
Revised : 18 November 2023
Accepted : 06 December 2023
Published : 04 January 2024
DOI : https://doi.org/10.1186/s40008-023-00320-z
Share this article
Anyone you share the following link with will be able to read this content:
Sorry, a shareable link is not currently available for this article.
Provided by the Springer Nature SharedIt content-sharing initiative
- Climate change
- Organic farming
JEL Classification
This site uses cookies to optimize functionality and give you the best possible experience. If you continue to navigate this website beyond this page, cookies will be placed on your browser. To learn more about cookies, click here .
- Reference Manager
- Simple TEXT file
People also looked at
Original research article, climate smart agriculture technologies adoption among small-scale farmers: a case study from gujarat, india.
- 1 Department of Agricultural Extension, University of Agricultural and Horticultural Sciences, Shivamogga, Shimoga, India
- 2 Division of Agriculture and Natural Resources, University of California, Merced, Merced, CA, United States
In India, 78% of farmers are small and marginal, cultivating only 33% of the arable land but producing 50% of the food grain; their vulnerability to climate change poses a significant threat to the country’s food security. To enhance agricultural resilience, it is crucial to understand how these farmers perceive and integrate climate-smart technologies into their farming practices. A random sample of 240 farmers was selected for this study. An ex-post facto research design was employed to investigate farmers’ awareness of and adoption of CSAT and identify the significant variables influencing their decisions. The results indicate that approximately 74 per cent of farmers had low to medium awareness of CSAT, while around 83 per cent had low to medium adoption rates. Several factors were found to be significantly correlated with farmers’ awareness and adoption of CSAT, including education level, annual income, exposure to agricultural mass media, participation in extension programs, innovativeness, achievement motivation, risk orientation, and scientific orientation. Additionally, farmers faced various challenges in adopting CSAT, such as the high cost of inputs, limited knowledge about CSAT, and youth migration from rural areas. Based on the study’s findings, farmers emphasized the importance of involving them in decision-making processes related to the development of climate-smart technologies. They also highlighted the need for a timely supply of inputs and field visits to successful farms as effective means to promote awareness and adoption of CSAT. The comprehensive analysis of associated factors and empirical findings presented in this study will benefit private sector organizations, government extension agents, academics, and policymakers. By gaining insights into the determinants of CSAT adoption, these stakeholders can focus their efforts more effectively on promoting widespread adoption. Additionally, this study can inform policy decisions regarding the allocation of government resources to combat climate change.
Introduction
Nine billion people must be fed by 2050, which will require an additional 70 per cent more food production ( FAO, 2009 ; Godfray et al., 2010 ; Thomas, 2011 ). Global food security is increasingly threatened by climate change ( Hebbsale Mallappa and Shivamurthy, 2021 ; Salerno et al., 2021 ). Climate change has several consequences, including rising temperatures, more frequent and intense extreme weather events, water shortages, rising sea levels, ocean acidification, land degradation, altered ecosystems, and a decline in biodiversity ( Chand et al., 2015 ; FAO, 2017 ; Pathak et al., 2018 ; Raza et al., 2019 ; Hatfield et al., 2020 ; Weiskopf et al., 2020 ). The IPCC report, released in 2019, highlights the significant role of land degradation as a contributing factor to climate change. The report emphasizes that land degradation leads to increased greenhouse gas emissions and reduced carbon uptake rates, exacerbating the effects of climate change ( Shukla et al., 2019 ). These factors could seriously threaten agriculture’s ability to produce and feed the most vulnerable population (resource-poor small-scale farmers) and delay achieving sustainable development goals ( Vågsholm et al., 2020 ). Research organisations, educational institutions, line departments, NGOs, and policymakers must cooperate to reduce agriculture’s contributions to climate change (GHG emissions) and involve agriculture and allied sectors in finding solution for rapidly changing environmental conditions ( Smith et al., 2014 ).
Climate variability plays a crucial role in shaping food production and farmers’ income in Gujarat and Indian agriculture ( Khatri-Chhetri et al., 2016 ). Nearly 60 per cent of yield variability can be attributed to climatic fluctuations ( Lobell and Gourdji, 2012 ; Aryal et al., 2018 ; Kukal and Irmak, 2018 ). The impacts of climate change are evident in the sowing and crop duration ( Malhi et al., 2021 ), as well as the intensity and duration of heat and water stress experienced by agricultural systems ( Burke et al., 2015 ). Higher average temperatures lead to reduced radiation interception and biomass production, hampering crop growth ( Zhao et al., 2017 ). Additionally, above-optimal temperatures directly impact the crop physiological processes.
Gujarat, being an agriculturally diverse state in India, cultivates cotton, groundnut, rice, wheat, maize and millet as major crops. These crops are significantly impacted by climate change, leading to detrimental effects on yields and overall agricultural productivity ( Aryal et al., 2020 ). For instance, studies have shown that increased temperatures and changing rainfall patterns negatively affect cotton production, with a projected decline of up to 14 per cent in yield by 2050 ( Patel et al., 2015 ). Groundnut, another important crop, is highly sensitive to temperature and water stress, resulting in potential yield losses of 18–20 per cent under climate change scenarios ( Malhi et al., 2021 ). Wheat, a staple crop, faces reduced yields due to rising temperatures, with estimated losses of 4–16 per cent by 2050 ( Tesfaye et al., 2017a ). Similarly, millets, which are drought-tolerant crops, are also vulnerable to changing rainfall patterns and increasing temperatures, leading to possible yield reductions of 10–20 per cent ( Tiwari et al., 2022 ). These statistics emphasize the urgent need to implement climate change adaptation strategies and promote climate-resilient agricultural practices to safeguard the productivity and sustainability of the major cropping systems in Gujarat, Anand.
Climate-smart agriculture has demonstrated its efficacy in delivering tangible benefits to farmers. According to studies, the adoption of climate-smart practices can increase farmers’ incomes by up to 30 per cent and enhance crop yields by 20–30 per cent ( Musafiri et al., 2021 ). Moreover, the implementation of climate-smart techniques has the potential to reduce greenhouse gas emissions from agriculture ( Khatri-Chhetri et al., 2016 ) by approximately 1.5 gigatons of carbon dioxide equivalent per year ( Ouédraogo et al., 2019 ). Additionally, the improved soil management practices associated with climate-smart agriculture can enhance soil organic carbon content by 0.3–0.6 per cent annually, contributing to better soil health and nutrient availability ( Aryal et al., 2015 ; Khatri-Chhetri et al., 2016 ). These statistics highlight the substantial economic, environmental, and climate change adaptation advantages that can be achieved through the widespread adoption of climate-smart agriculture ( Holden et al., 2018 ).
The economic viability of the agricultural production system depends on the farmer’s capacity to acclimatise their farming structures in opposition to the ecological and financial stress and vagaries ( FAO, 2015a ; Ministry of Agriculture and Farmers’ Welfare, 2015 ). Adaptation strategies against climate change are essential for enhancing the supply of raw materials to attain economic security and to boost net farm revenue and the raw material supply from farming and allied businesses under the climate change regime ( Parajuli et al., 2019 ; World Bank, 2020 ; Gustafson et al., 2021 ). FAO has initiated eight action programs, such as (1) irrigation and drought management, (2) climate-resilient agricultural systems, (3) sustainable forest and land management, (4) towards effective fisheries sector, (5) improving food and livelihood security by the reducing methane emissions, (6) effective planning and allocation of funds to promote adaptation strategies towards climate change, (7) genetic diversity and climate change, and (8) saving food and avoiding waste ( FAO, 2015b ). CSAT enhances yield and socio-economic conditions that align with reducing GHG emissions. Hence, new farming approaches will be required to ensure food security in the face of future climate change ( IPCC, 2012 ; Philip and Leslie, 2014 ; Vinaya Kumar et al., 2017 ).
The farmers’ level of efficiency in realising net revenue and utilising resources towards mitigating climate change is based on their adaptation strategies, such as crop choice, crop diversification, efficient irrigation systems, and the introduction of livestock components ( Feliciano, 2019 ; World Bank, 2021 ). Land use and water resources have a significant impact on climate change in agriculture. There are various hurdles in mitigating climate change due to limited progress in drip irrigation, aerobic cultivation, and the use of drought-tolerant crop varieties with effective root systems, as well as the persistant burning of crop residues and the lack of tree planting in wastelands and unutilised cultivable lands ( Lulia, 2012 ; Patle, 2021 ).
Despite the potential benefits, the adoption of CSAT is very low in India and other developing countries. To increase the adoption of CSAT, it is essential to enhance the understanding of small and marginal farmers regarding adaptation and mitigation strategies for climate change. The rate of diffusion strategies used by the development departments significantly impacts the speed at which technology is accepted and adopted.
Additionally, a number of factors have been linked to the awareness and adoption—or non-adoption—of technologies ( Scott et al., 2008 ; Petronilla et al., 2016 ). Most studies have focused on one or two dimensions of household characteristics, asset base, and farm characteristics and their influence on the adoption of CSAT ( Kurgat et al., 2020 ; Ayat et al., 2022 ; Negera et al., 2022 ). However, the influence pattern of these factors is often complex and context-specific, depending on the location and the technologies. Although psychological and situational factors play a significant role in technology adoption, no studies have focus on these factors and their influence on the awareness and adoption of CSAT. Hence, the present study is novel in understanding the complex relationship between the socio-psychological factors and their influence on the awareness and adoption of CSAT.
The small-scale farmers in the study area are frequently affected by erratic rainfall, waterlogging problems, salinity problems, incorrect agronomic practices, and flash floods during August–September, which have led to a decrease in field crop yields, ultimately affecting farmer profits ( Shaw et al., 2005 ; Sivakumar and Stefanski, 2010 ; FAO, 2011 ; Mehta, 2019 ). Studying farmers’ concerns regarding knowledge, adoption, and barriers to adopting CSAT will be extremely helpful in analysing the needs and requirements of farmers. With this backdrop, the study focuses on answering the following questions and hypotheses.
1. What is the socio-economic and psychological profile of the farmers?
2. Are farmers aware of CSAT? If yes, then up to what extent are they aware of CSAT?
3. How well do farmers cope with changing climatic scenarios by adopting CSAT?
4. What personal, social, economic, and psychological characteristics influence the farmers’ awareness of and adoption of CSAT?
5. Are farmers facing any difficulties in the adoption of CSAT to mitigate the ill effects of climate change? If yes, what are their suggestions for promoting CSAT?
Hyphotheses: (H 0 ):
6. There is no significant relationship between the socio-economic and psychological profile of the farmers and their awareness of and adoption of Climate-Smart Agriculture Technologies (CSAT).
7. (H 0 ): Farmers do not face any difficulties in the adoption of CSAT to mitigate the ill effects of climate change.
Understanding the significance of the study lies in its potential to provide evidence-based recommendations and guidelines for policymakers, extension agents, and other stakeholders involved in agriculture and rural development. By identifying the factors that influence farmers’ awareness and adoption of CSAT, tailored interventions and support systems can be designed to enhance climate resilience in the agricultural sector. Furthermore, addressing the difficulties faced by farmers in adopting CSAT and incorporating their suggestions into strategies for promoting these technologies will ensure the relevance and effectiveness of future climate change mitigation initiatives.
This study’s findings have the potential to inform policy decisions and resource allocation, enabling targeted investments in climate-smart agricultural practices and technologies. By bridging the gap between scientific research and on-the-ground implementation, this research contributes to the broader goal of sustainable and resilient agriculture in the face of climate change. Ultimately, the significance of this study lies in its potential to facilitate transformative changes in agricultural practices, leading to improved food security, livelihoods, and environmental sustainability in Gujarat, India, and beyond.
Methodology
The investigation was conducted in Anand district (22.3299° N, 72.6151° E) of Gujarat, India. The primary crops in the district are cotton, groundnut, rice, wheat, and tobacco. Other important crops include banana, mango, lemon, papaya and other seasonal vegetables. The average size of land holdings is 0.96 Ha, and small and marginal farmers own about 30.12% of the total land area. Climate factors include temperature and precipitation, which vary greatly from season to season, with summers typically being hot and winters typically being cool. The mean maximum temperature ranges between 28.4°C during January to around 41.8°C during May, while the mean minimum temperatures fluctuate between 11.7°C during January and 27°C during June. The long-term average annual rainfall is about 799 mm. The majority of precipitation occurs between June and September during the southwest monsoon. The district has a substantial network of canals (Mahi Right Bank Canal Command Area), and it is their major source of irrigation.
For the study, the district’s Agriculture Officers (AOs) were consulted to assist in selecting talukas, and they were asked to suggest villages where farmers were partially or fully adopting CSAT. In order to choose 240 farmers from 16 villages for the study area, 15 farmers were randomly chosen from each of the selected villages. The investigation was carried out using the Ex-Post-Facto research design.
Operationalisation of dependent variables
In this study, awareness refers to the first-hand information obtained by farmers about the CSAT in the farming system. Awareness is essential because it motivates individuals to obtain further information and take action. It represents the first step in the process of adoption.
A schedule was developed to assess farmers’ awareness regarding CSAT. For this purpose, all relevant items about the CSAT were included, and the schedule was developed by referring to literature and consulting experts from multidisciplinary subjects of agriculture. The schedule consisted of 75 items with multiple choices, such as“Fully Aware,” “Partially Aware,” and “Not Aware.” A score of two was assigned if the farmer was fully aware of an item, a score of one if the farmer was partially aware, and a score of zero if the farmer was not aware. The total score for each respondent was calculated accordingly. Based on their awareness scores using the mean and standard deviation, the respondents were divided into three groups.
Adoption in this study referred to the investigation of CSAT into farmers’ farming practices. The technologies were selected from a package of practices and other literature reviews after discussions with subject matter specialists from Anand Agricultural University and the Gujarat state agriculture department. The scoring pattern for adoption was the same as mentioned in the awareness component.
The flow chat shows the relationship between climate change awareness, adoption of Climate-Smart Agriculture (CSA) practices, and farmers’ income ( Figure 1 ). It demonstrates the sequential steps involved, starting with increasing awareness about climate change and its impacts. From there, it shows farmers’ decision-making process regarding adopting CSA practices, which can include various sustainable techniques. The flowchart highlights how adopting CSA practices can impact farmers’ income through increased productivity and reduced production costs. It emphasizes the significance of climate change awareness, adoption and sustainable farming practices in promoting farmers’ income and resilience in the face of climate change challenges.
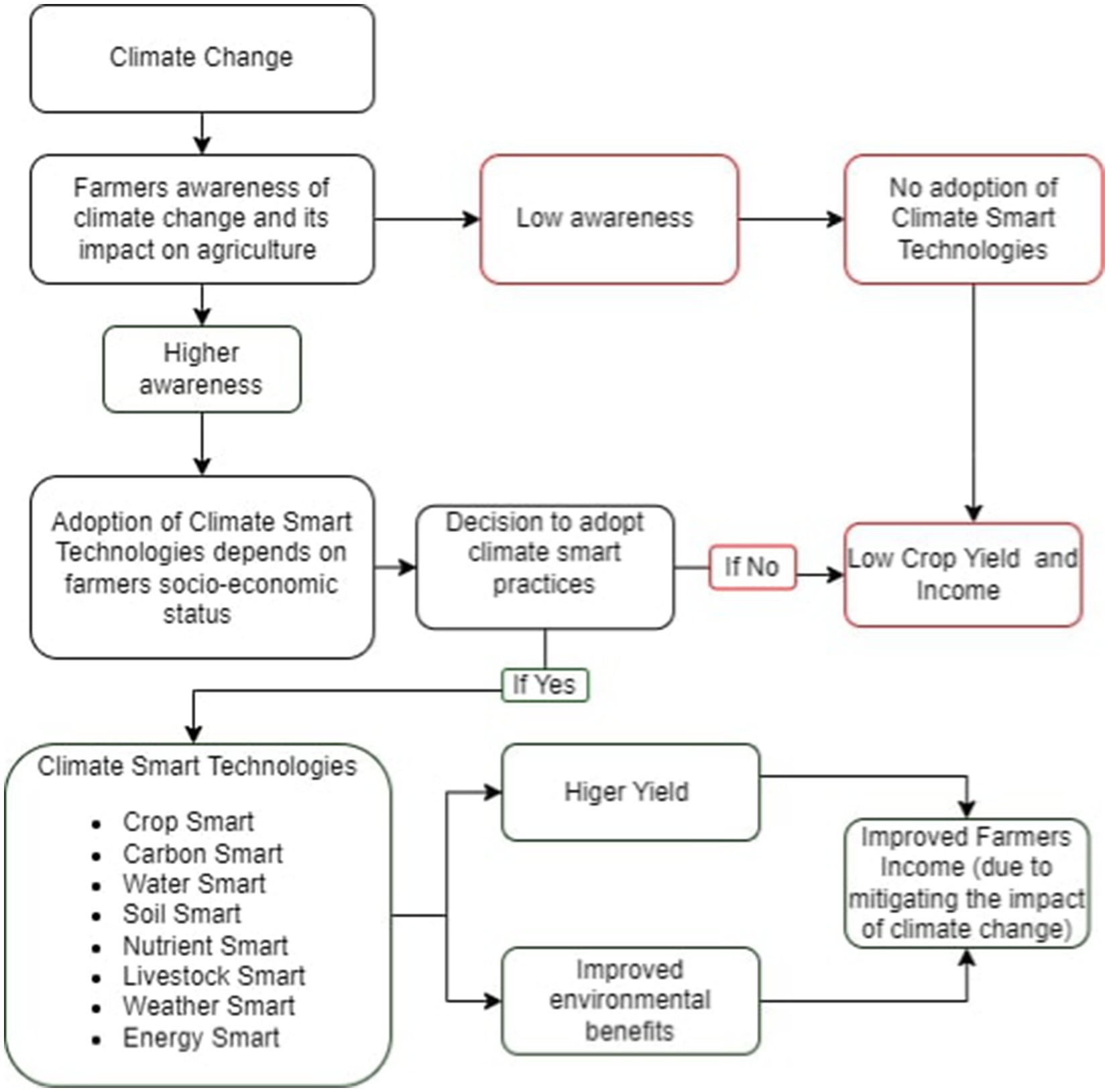
Figure 1 . Flowchart presenting the relation among climate change awareness, adoption, CSA practices and farmers’ income.
Survey data and analysis
A standardised schedule comprising all the components of CSA technologies was developed with the help of agricultural extension, agronomy, and soil science experts. The interview schedule was pre-tested in a non-sample area to identify any unclear questions, and necessary corrections were made to the final interview schedule thereafter. The data were collected through in-person interviews using a structured interview schedule to gather qualitative and quantitative information about CSA. During the household interview, the primary decision-maker for the family was questioned about several CSA traits, specifically regarding their adoption in their farming system. The collected data were analysed using appropriate statistical tools, i.e., descriptive statistics, Spearman correlation, regression, principal component analysis, and path analysis.
Path analysis
Path coefficient analysis ( Wright, 1921 ) was used to determine the direct and indirect effects of predictive factors’ on farmers’ awareness and adoption of CSAT. The path co-efficient method extends the conventional partial regression coefficient method. The path analysis was carried out using SPSS software, and a diagram was developed by Drawings.net software. Path effects were obtained by solving the simultaneous equations set up for this purpose using the correlation matrix and considering one variable ‘1’ to be influencing the other variable ‘1’. the simultaneous equation would be:
r yxi = P yxi r xixj 𝑥 p yxi
For i = 1, 2, 3, ………., n
For j = 1, 2, 3, ………., n
r yxi = Correlation coefficient between X i with Y,
Pyxi = Direct effect of X i variable to Y variable, and
∑ i . j = 1 n r xixj 𝑥 p yxi = Indirect effect of the independent variable to a dependent variable via., another independent variable.
Results and discussion
Socio-economic-psychological characteristics of the farmers.
The information in Table 1 shows the detailed profile of respondents from the study area. Table 1 demonstrates that two-thirds of respondents (65.40%) were in the old age group, followed by the middle-aged (32.90%) category and the young (1.7%). Regarding educational level, secondary education accounts for the majority of responses (39.20%), followed by higher-secondary education (22.50%), degrees and above (20%), and primary education (18.30%). A large percentage of respondents (almost 71%) have a high degree of agricultural experience. More farmers have families that range in size from four to eight persons, followed by small families (34.17%) and large families (15.83%). Approximately 61 per cent of respondents belong to a joint family. Sixty-one and a half per cent of farmers claimed to work in agriculture and animal husbandry, while 31.25 per cent claimed to be engaged solely in the agricultural sector.
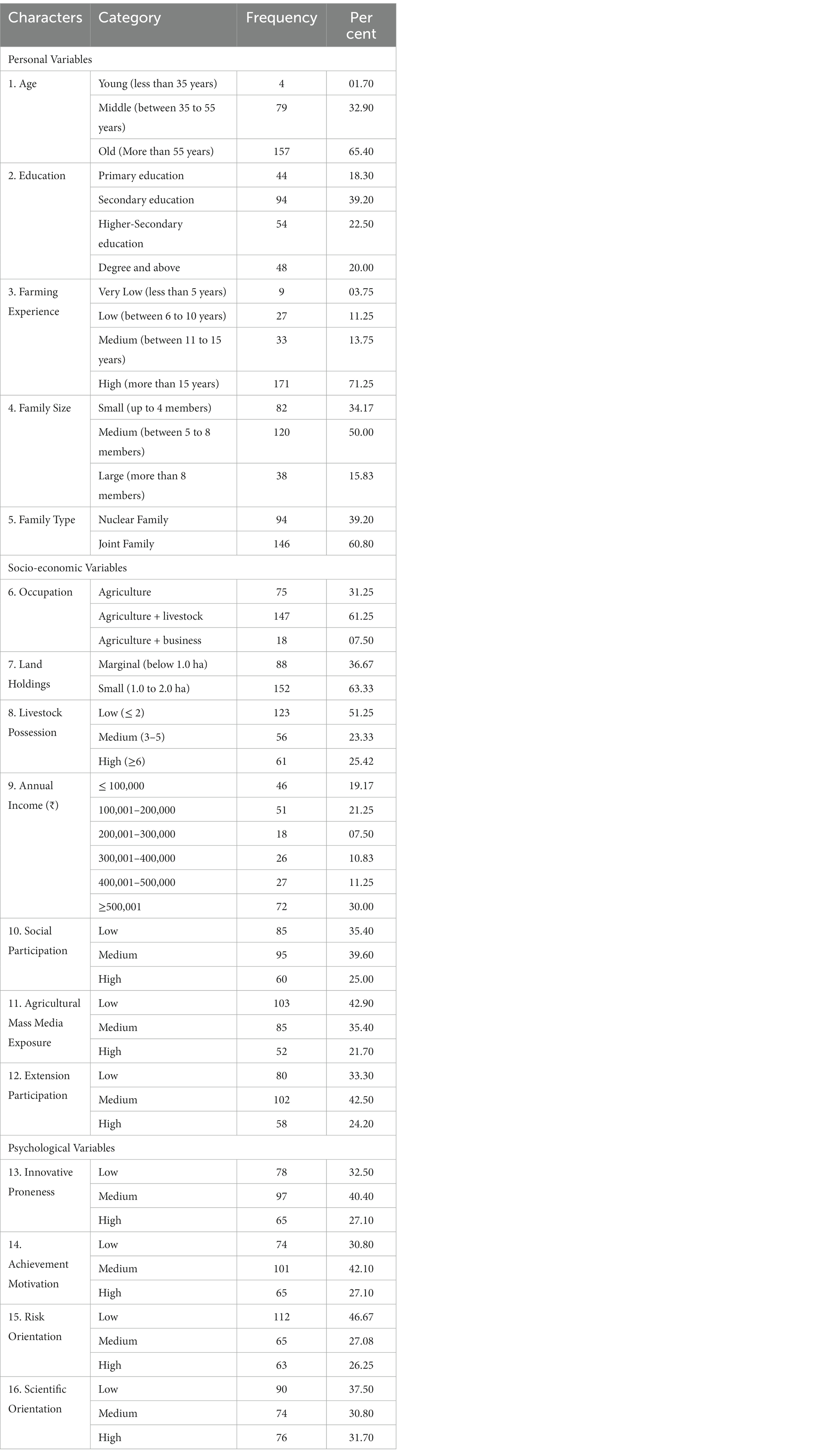
Table 1 . Personal, socio-economic and psychological characteristics of the farmers ( n = 240).
Table 1 shows that nearly two-thirds of the farmers (63.33%) are small farmers, followed by marginal farmers (36.37%). This could result from fragmented land ownership and the passing down of land from generation to generation. Over half of the respondents (51.25%) own low livestock, while high and medium livestock are owned by 25.42 per cent and 23.33 per cent of respondents, respectively.
Regarding annual income, 30 per cent of respondents are classified as high earners. Nearly two-fifths (39.60%) of respondents belong to a group with a medium degree of social participation. A higher percentage of respondents (42.90%) have low levels of exposure to agricultural media, followed by medium (35.40%) and high (21.70%) levels.
A little over two-fifths (42.50%) of the respondents have a medium level of engagement with extension services, followed by 33.30 per cent of farmers with a low level and 24.20 per cent with a high level. Two-fifths of respondents (40.40%) are classified as having a medium level of innovative proneness, followed by 32.50 per cent for low and 27.10 per cent for a high innovative proneness category.
Around 42 per cent of farmers have medium levels of achievement motivation, followed by 30.80 per cent with low and 27.10 per cent with high levels of achievement motivation. A higher percentage of farmers (46.67%) are low-risk-oriented and they also have a low level of scientific orientation (37.50%).
Psychological and economic factors significantly influence farmers’ awareness and adoption of CSAT ( Djufry et al., 2022 ; Kifle et al., 2022 ). However, the present study discovered that these factors, including personal, socio-economic, and psychological factors, fell into the low to medium range among the farmers. It is highly challenging to quickly improve the farmers’ financial situation without addressing these traits. Nonetheless, farmers can be taught and have their positive attitudes toward CSA technologies can be changed through adequate education or capacity-building programmes, which can lead to their decision to try and adopt the CSA technologies in their farming systems ( McNamara et al., 1991 ; Murage et al., 2015 ). Therefore, efforts in this regard must be undertaken to provide farmers with the tools they need to combat the adverse effects of climate change on their farms and livelihoods ( Tama et al., 2021 ).
Farmers’ awareness of CSAT
The data in Table 2 revealed that for the first component, crop smart, the majority of the respondents (92.50%) were aware of short-duration varieties, followed by high-yielding varieties (90.83%), disease-resistant varieties (83.75%), pest-resistant varieties (83.33%), and mixed cropping (65.83%). Thus, it is evident that the farmers in the area were well aware of the varieties of crops such as banana, wheat, and other seasonal vegetables.
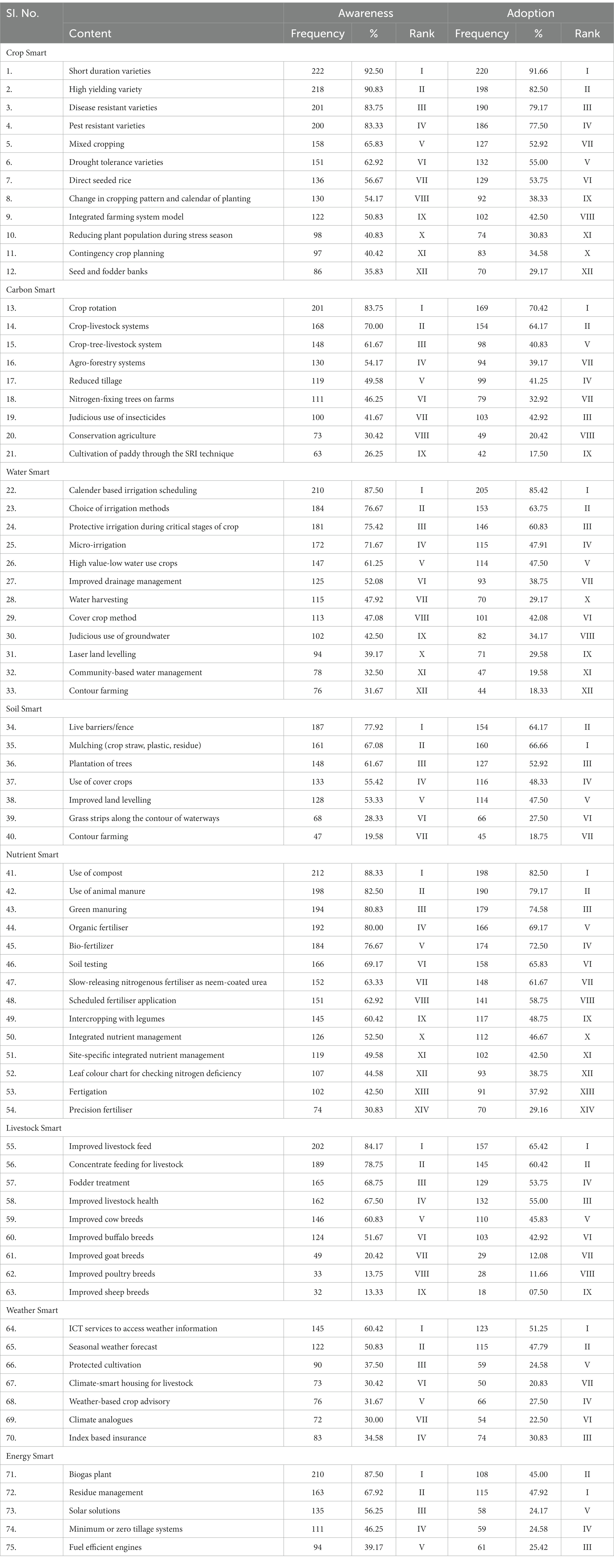
Table 2 . The farmers’ awareness and adoption of CSAT ( n = 240).
In the case of carbon smart, 83.75 per cent of the respondents acknowledged awareness of crop rotation awareness, followed by crop-livestock systems (70%), crop-tree-livestock systems (61.67%), agro-forestry systems (54.17%), and reduced tillage (49.58%).
According to the data in Table 2 regarding respondents’ awareness of water smart practices, most of the farmers are aware of irrigation scheduling, followed by the choice of irrigation methods (76.67%), protective irrigation during critical crop stages (75.42%), micro-irrigation (7.17%), and high-value-low water use crops (61.25%).
Table 2 shows that 77.92 per cent of farmers were aware of soil smart technologies in relation to the statement “live barriers/fences,” whereas 67.08 per cent were aware of mulching, 61.67 per cent were aware of planting trees, and 55.42 per cent were aware of using cover crops.
In terms of nutrient smart awareness, 88.33 per cent of respondents were aware of compost, 82.5 per cent were aware of animal manure, 80.83 per cent were aware of green manuring, 80 per cent were aware of organic fertilizer, and 76.67 per cent were aware that bio-fertilizer was used in climate-smart farming.
According to the information on livestock smart awareness in Table 2 , 84.17 per cent of farmers were aware of improved feed for livestock, followed by 78.75 per cent who were aware of concentrate feeding, 68.75 per cent who were aware of treating fodder, 67.50 per cent who were aware of improved livestock health, and 60.83 per cent who were aware of improved cow breed practices.
According to Table 2 , when it comes to being weather-smart, 60.42 per cent of respondents are aware of ICT services to access weather information, while 50.83 per cent are aware of for seasonal weather forecasts. In addition, 37.50 per cent are aware of protected cultivation, and 34.58 per cent are aware of index-based insurance.
In the energy-smart category, 87.50 per cent of the farmers are aware of biogas plants, followed by 67.92 per cent of the farmers are aware of residue management, 56.25 per cent are aware of solar solutions, and 46.25 per cent are aware of minimum or zero tillage systems.
It is logical to conclude from the above results that practices that are complex, highly skill-oriented and difficult to understand are least known to farmers ( Ravi and Ridhima, 2019 ; Muhammad and Marie, 2021 ). On the other hand, the practices that are simple, less costly, and have being practiced by their forefathers have higher awareness among farmers.
Adaptation strategies regarding CSAT
According to the findings in the Table 2 , high-yielding varieties have been adopted by 82.50 per cent of farmers, while disease-resistant varieties have been adopted by 79.17 per cent of respondents. Short-duration varieties have been adopted by 91.66 per cent of respondents, and pest-resistant varieties have been adopted by 77.50 per cent of respondents.
In the case of carbon smart, 70.42 per cent of the respondents have adopted crop rotation as an adaptation measures. Additionally, 64.17 per cent of farmers have adopted a crop-livestock system, 42.92 per cent have wisely used insecticides, 41.25 per cent have adopted reduced tillage, and 40.83 per cent have implemented a crop-tree-livestock system.
Regarding water-smart technologies, where 85.42 per cent of respondents have adopted calender-based irrigation scheduling, followed by 63.75 per cent who have used protective irrigation at crucial stages of the crop. Micro-irrigation has been adopted by 60.83 per cent of farmers, and high-value-low-water-use crop technologies have been adopted by 47,91 per cent of farmers.
In the case of soil-smart technologies, 66.66 per cent of farmers have adopted mulching, followed by 64.17 per cent who have adopted live barriers. Additionally, 52.92 per cent of them adopted tree planting, 48.33 per cent have adopted cover crops, and 47.50 per cent of farmers have adopted improved land leveling technologies in their farming systems.
Table 2 clearly indicates that compost technology has been adopted by 82.50 per cent of respondents in the case of nutrient smart technologies. Comparatively, 79.17 per cent have used animal manure, 74.58 per cent have adopted green manure, 72.50 per cent have used biofertiliser, and 69.17 per cent have adopted organic fertiliser. Regarding livestock-smart technologies, 65.42 per cent of the farmers have adopted improved livestock feed, 60.42 per cent have adopted concentrate feeding for livestock, 55 per cent have adopted improved livestock health management practices, and 53.75 per cent have adopted fodder treatment practices in their livestock-based farming systems.
Table 2 shows that 51.25 per cent of respondents have adopted ICT services to obtain weather data, followed by 47.79 per cent who have adopted seasonal weather forecasts, and 30.83 per cent who have adopted index-based insurance.
According to the information in Table 2 , around 48 per cent of the farmers have adopted residue management practices in their farming to manage the energy requirement, followed by 45 per cent who have adopted biogas plant technologies. Only 25.42 per cent of the respondents have adopted fuel efficient engines to meet the energy requirement in farming.
This kind of observation might be because farmers have resorted to using cost-effective and remunerative measures ( Sivabalan and Nithila, 2018 ; Ravi and Ridhima, 2019 ; Muhammad and Marie, 2021 ; Mujeyi et al., 2021 ). Furthermore, other reasons such as extension agencies might not have educated the farmers about the CSAT, or they might have neglected these particular technologies due to their high financial investment.
A considerable number of farmers have adopted biofertilisers, organic fertilisers, weed management, and improved varieties. This certainly indicates a gradual change in the affective domain of farmers towards using fewer chemical control measures.
Farmers’ overall awareness level and adoption of CSAT in their farming system
The results presented in Figure 2 indicate the levels of awareness and adoption of Climate-Smart Agriculture Technology (CSAT) among farmers. Approximately 39 per cent of the farmers exhibited a low level of awareness, while 42.50 per cent had a low level of adoption of CSAT in their farming systems. On the other hand, a medium level of awareness was observed in about one-third (34.58%) of the farmers, and 40.42 per cent fell into the medium category of adoption. Interestingly, only one fouth (26.25%) and less than one-fifth (17.08%) of the farmers demonstrated a high level of awareness and adoption of CSAT, respectively. The Chi-square value of 127.809 indicates a significant correlation between the awareness and adoption of CSA technology.
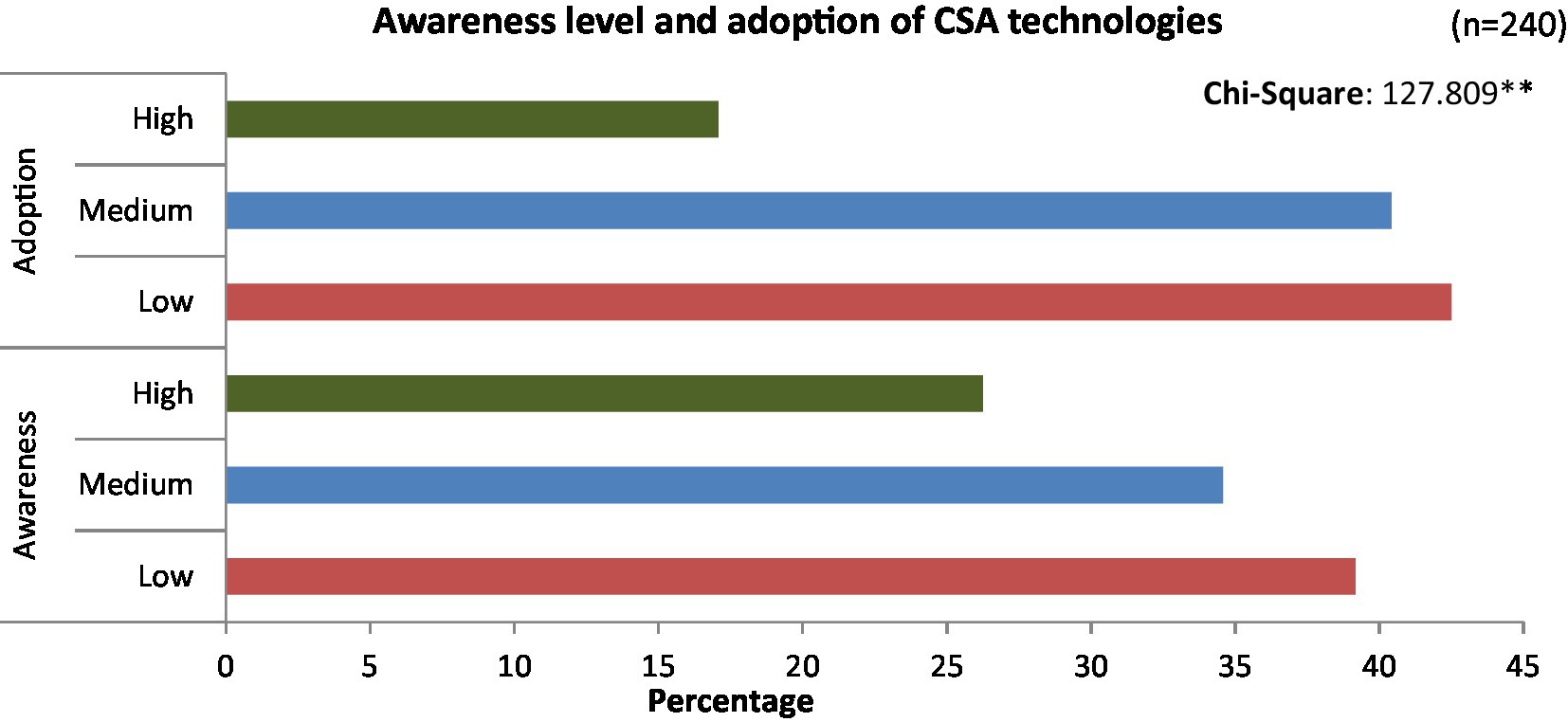
Figure 2 . Overall farmers’ awareness level and adoption of CSA technologies in their farming system. ** = significant at 0.01 per cent level.
Based on these findings, it is evident that there is room for improvement in enhancing farmers’ awareness and adoption of CSA technology. The results suggest that efforts should be made by the government, line departments, and universities to focus on increasing farmers’ awareness of CSAT. By doing so, farmers can develop a positive attitude towards CSA technology, which, in turn, will likely encourage active implementation of CSAT on their farms ( Aryal et al., 2018 ; Mwungu et al., 2018 ). This emphasis on awareness-building can lead to a more widespread and effective adoption of climate-smart agricultural practices, ultimately contributing to the sustainability and resilience of farming systems in the face of climate change.
Relationship between farmers’ overall awareness of CSA technologies and their socio-psychological factors
A correlation test was conducted to examine the relationship between farmers’ profile traits and their overall awareness of CSAT. The findings are presented in the Table 3 . Eight factors, namely education, annual income, exposure to agricultural media, participation in extension programmes, innovative proneness, achievement motivation, risk-taking, and scientific orientation,were positively and significantly related to farmers’ awareness levels at the 1 per cent level of significance. On the other hand, three factors, namely farming experience, family size, and family type,were negatively and significantly related to farmers’ awareness level at 1 per cent level of significance. Other factors had tangential connections to farmers’ awareness of CSA technologies.
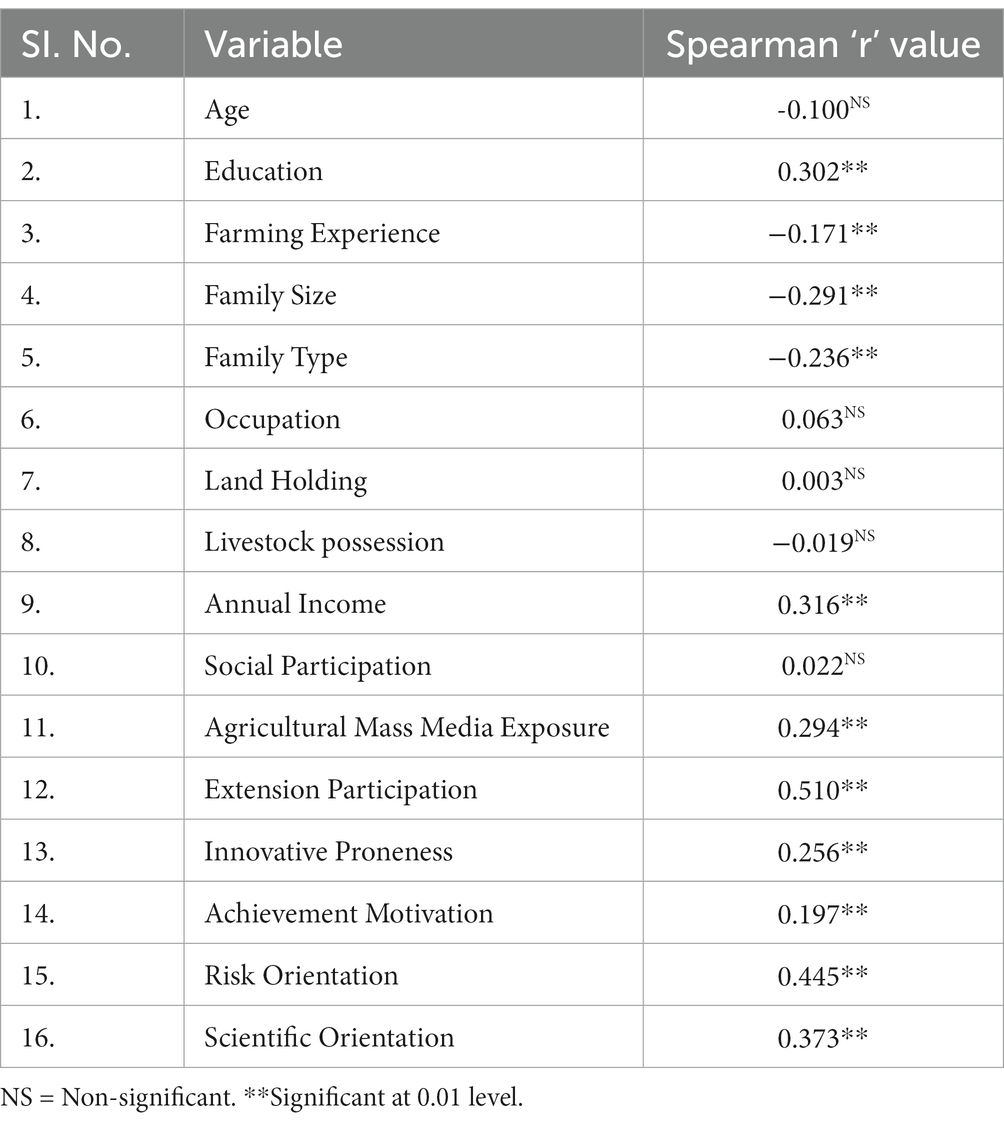
Table 3 . Correlation (r) between the profile of the farmers and awareness of CSA technologies ( n = 240).
Further, stepwise regression analysis was employed to determine the impact of the seven significantly associated variables on farmers’ awareness of CSAT (as shown in Table 4 ). The findings revealed that these seven factors explained 48.30 per cent of the variation in farmers’ CSAT awareness levels.
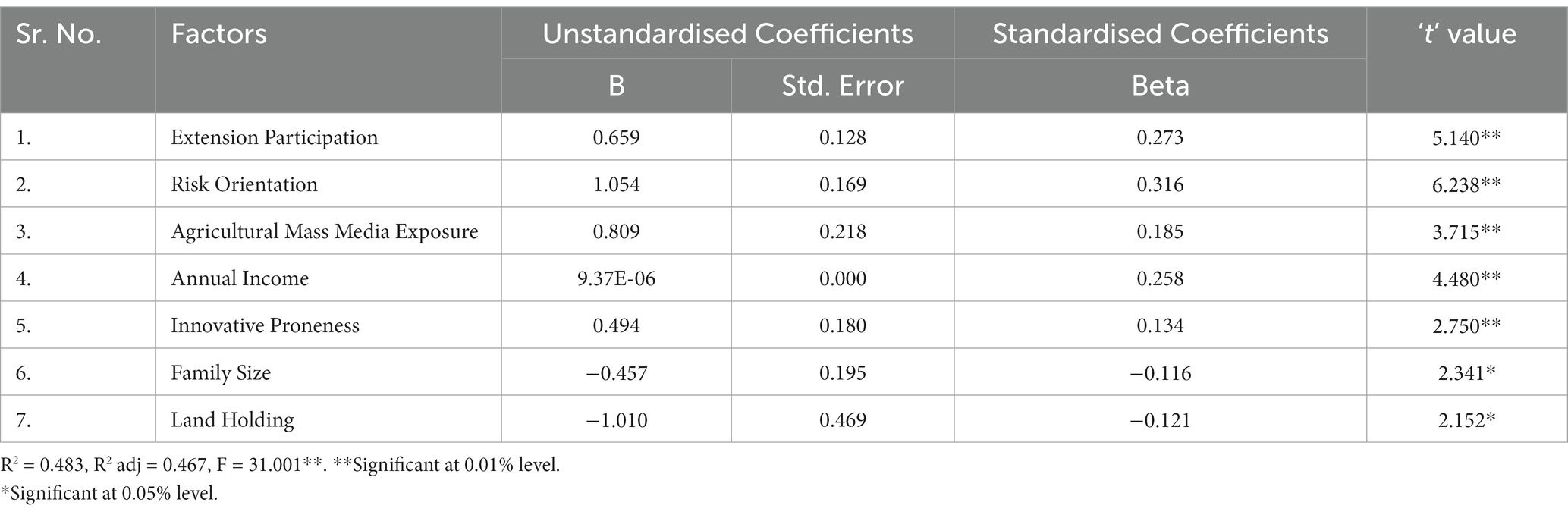
Table 4 . Regression analysis demonstrating the relative significance of profile characteristics of farmers in determining their awareness of CSAT ( n = 240).
The results emphasize the importance of considering farmers’ profile traits in efforts to enhance awareness of CSAT. By understanding the factors that influence farmers’ awareness levels, policymakers and development agencies can design targeted interventions and support mechanisms to promote the adoption of climate-smart agricultural practices and contribute to the sustainable development of farming systems ( Miheretu and Yimer, 2017 ; Chandio and Yuanshend, 2018 ; Mota et al., 2019 ).
Relationship between profile characteristics of farmers and their overall adoption of CSAT
The findings of the correlation analysis between the profile characteristics of farmers and their overall adoption level are presented in Table 5 . Among the 16 variables considered in the study education, occupation, annual income, social participation, exposure to agricultural media, participation in extension programmes, innovative proneness, achievement motivation, risk orientation, and scientific orientation were positively and significantly related to the adoption level at a 0.01 per cent level of significance. On the other hand, age, agricultural experience, family size, and family type were other factors that were negatively significant at a 1 per cent level of significance.
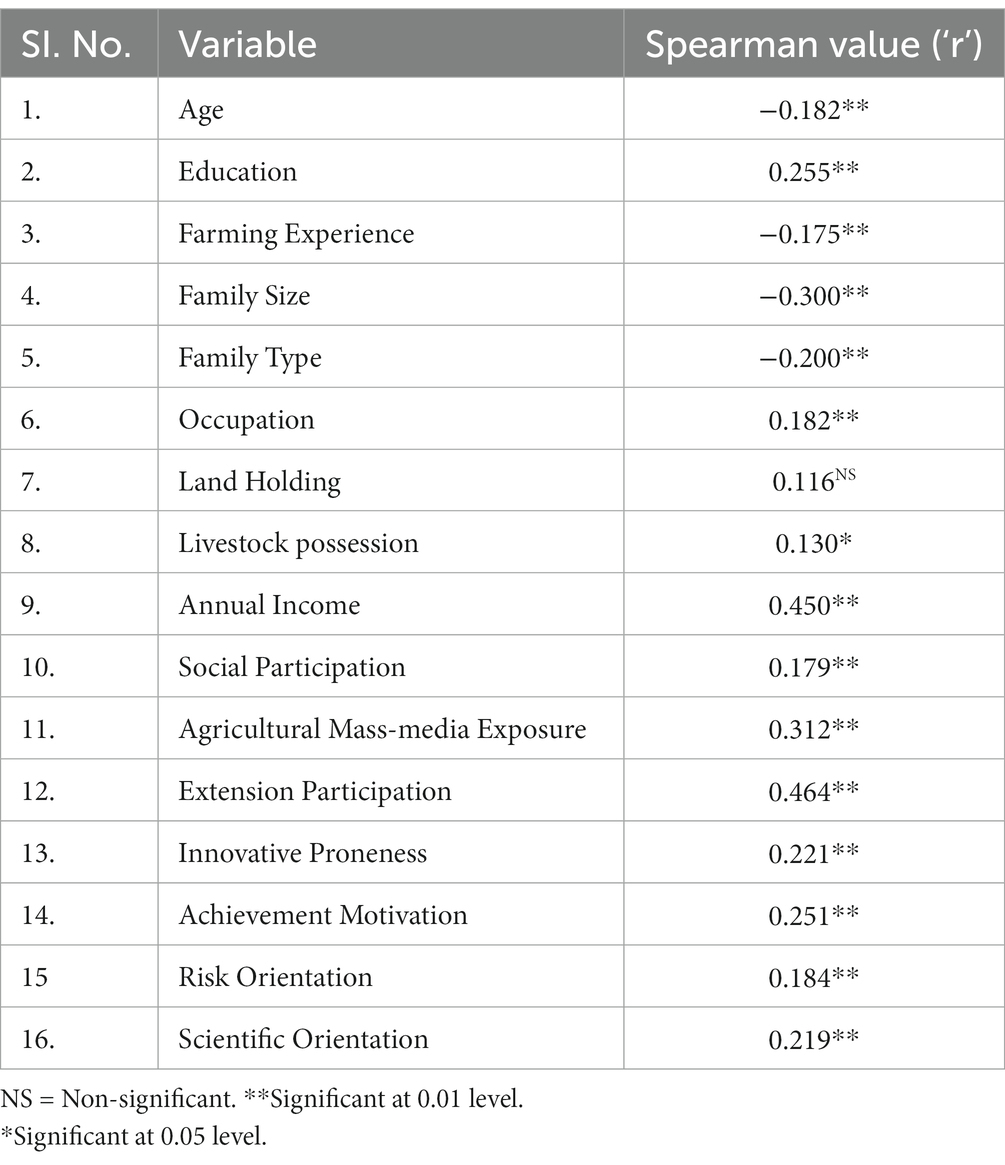
Table 5 . Correlation analysis between the profile of the farmers and the adoption of CSAT by farmers’ ( n = 240).
Additionally, stepwise regression analysis was conducted to determine the impact of these 10 significantly associated variables on farmers’ adoption of CSAT (as shown in Table 6 ). The findings revealed that out of the 10 factors, four factors explained 41.90 per cent of the variation in farmers’ adoption of CSAT.
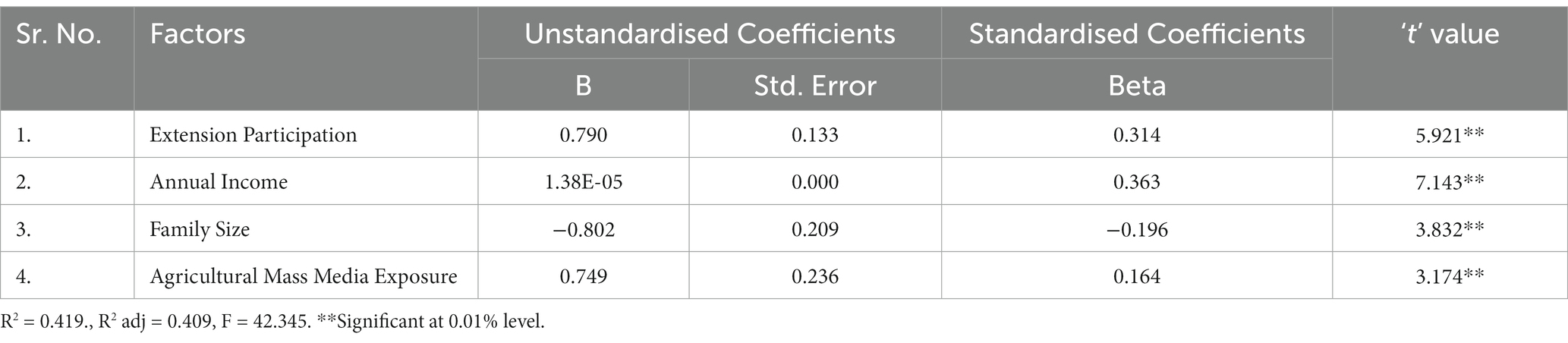
Table 6 . Regression analysis demonstrating the relative significance of profile characteristics of farmers in determining their adoption of CSAT ( n = 240).
Based on these findings, it is crucial for governments and other development agencies to prioritize efforts in enhancing the profile characteristics that are significantly linked to farmers’ adoption of CSAT. By focusing on improving education levels, creating job opportunities, increasing annual income, promoting social participation, enhancing exposure to agricultural media, facilitating participation in extension programs, and fostering characteristics such as innovative proneness, achievement motivation, risk orientation, and scientific orientation, the overall adoption of CSAT among farmers can be significantly improved. Additionally, attention should be given to addressing the negative correlations associated with age, agricultural experience, family size, and family type, as these factors hinder farmers’ adoption and need to be carefully considered in adoption promotion strategies ( Belay et al., 2017 ; Ouédraogo et al., 2019 ; Mujeyi et al., 2021 ).
The determinants of farmers’ awareness and adoption of CSAT
The process of selecting elements to include in a model is a crucial issue in understanding the relationship between variable groupings. To address subjectivity and other estimation problems in conventional analysis like regression, the use of Principal Component Analysis (PCA) can provide theoretically and statistically sound approach. PCA can also aid in understanding the regression equation. The analysis of the findings is presented in Table 7 .
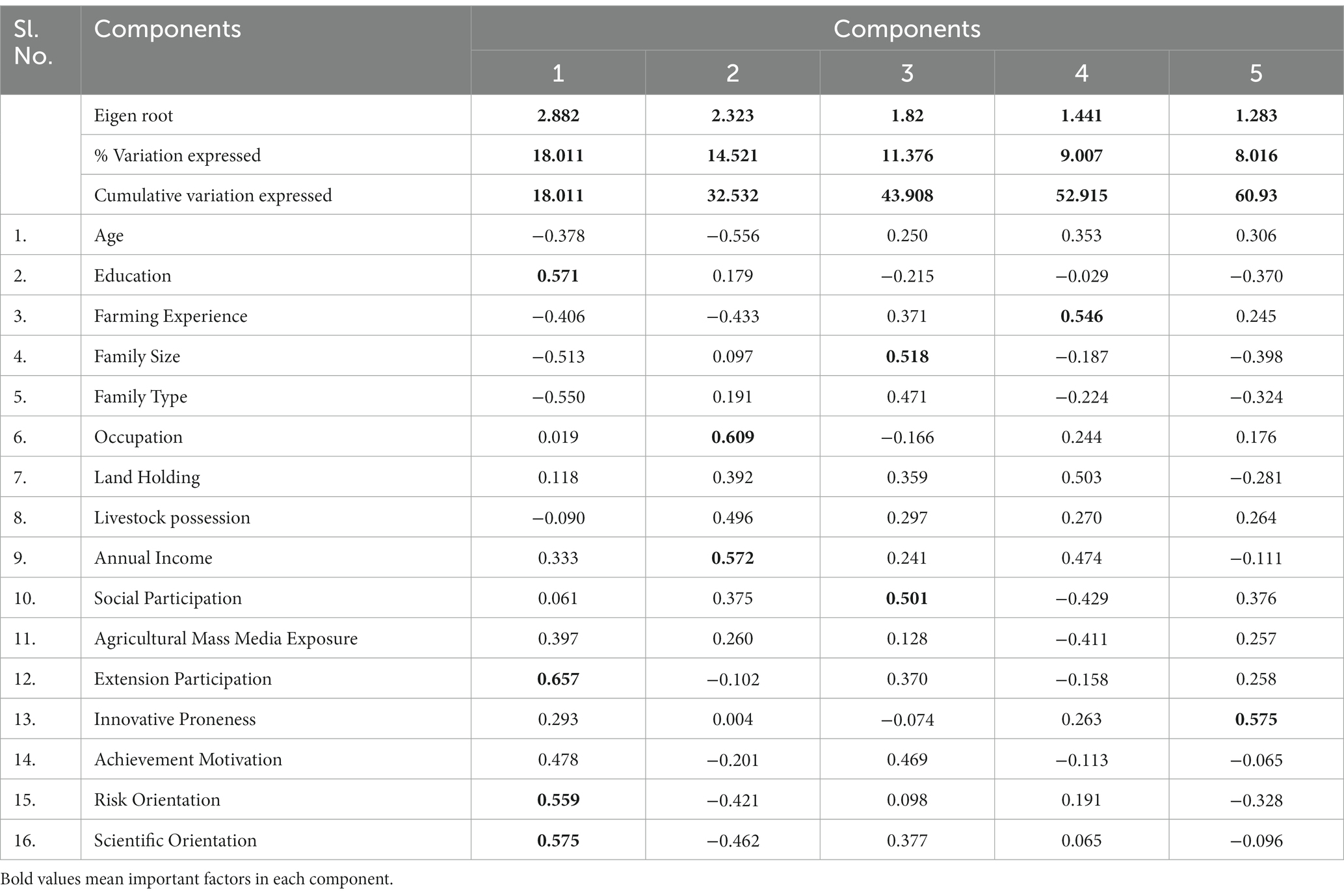
Table 7 . Contribution of factors on awareness and adaption of CSAT ( n = 240).
The analysis revealed that the first component accounts for more than 18 per cent of the variations in the possible combinations of the 16 variables. When combined, the five factors explain over 60 per cent of the overall variation. The first component implicitly demonstrates the relationship between elements related to CSA technology and psychological components. The examination of the second primary component highlights the significance of economic factors ( Abegunde et al., 2019 ; Mujeyi et al., 2019 ; Ouédraogo et al., 2019 ; Tran and Goto, 2019 ).
These findings highlight the importance of considering psychological and economic factors in promoting the adoption of CSA technology. Policymakers and development agencies should recognize the psychological aspects that influence farmers’ decision-making processes, such as attitudes, motivations, and risk perceptions. Additionally, they should address the economic factors that affect the feasibility and profitability of adopting CSA technology.
Path effects of farmers’ profile traits on their awareness and adoption of CSA technology in their farming system
According to the data presented in Tables 8 , 9 ; Figures 3 , 4 , involvement in extension programs had the greatest direct positive impact on farmers’ awareness of CSA technologies, followed by risk orientation and annual income. On the other hand, the adoption of CSA technologies was significantly influenced by extension contact, media exposure, and annual income. Landholding, farming experience, and social participation had the least direct impact on awareness of CSAT among the farmers. The findings suggests that factors such as family size, land ownership, and farming experience had the least direct effects on the adoption of CSA technologies by farmers.
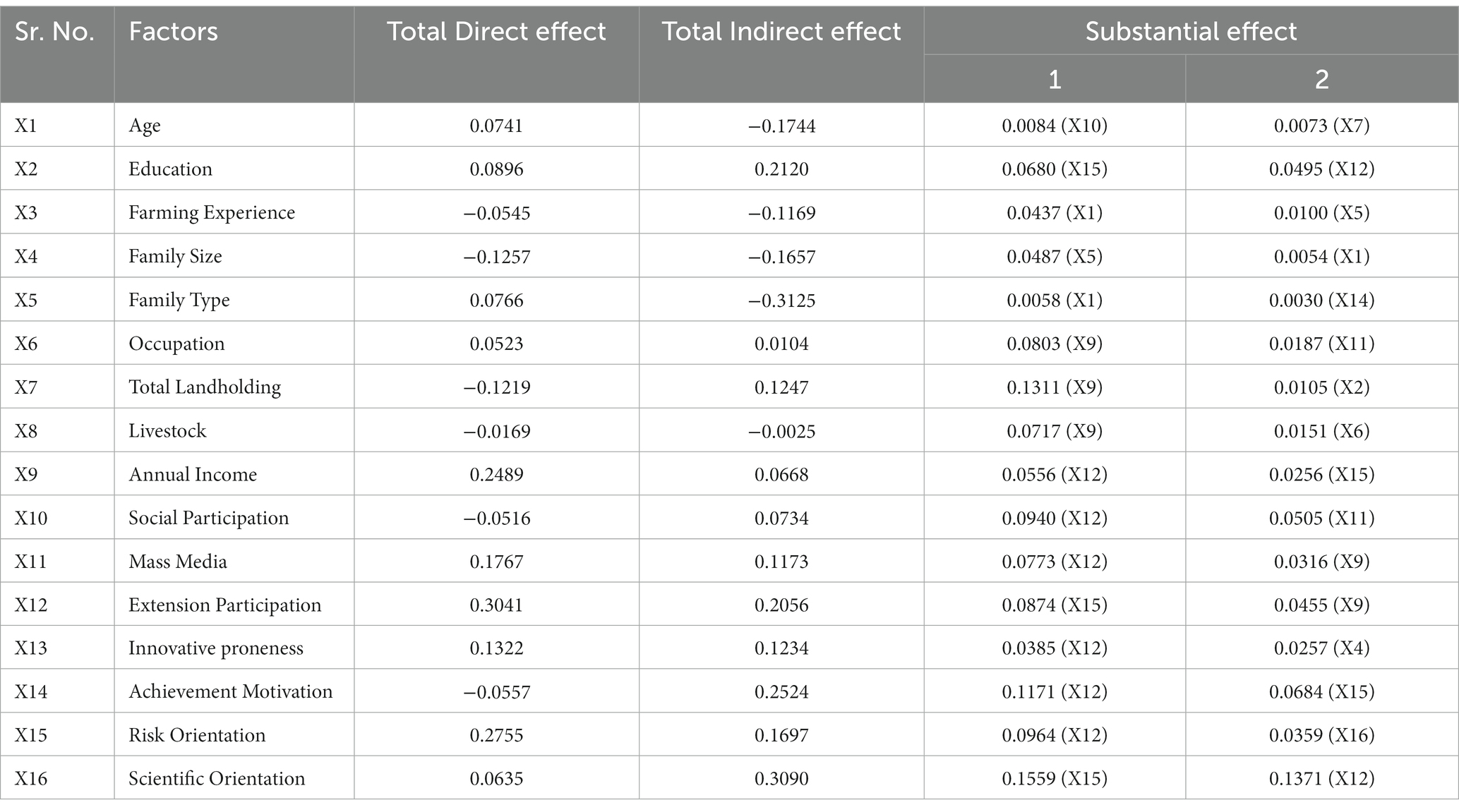
Table 8 . Path effect of selected characteristics of the farmers on awareness about CSA technologies ( n = 240).
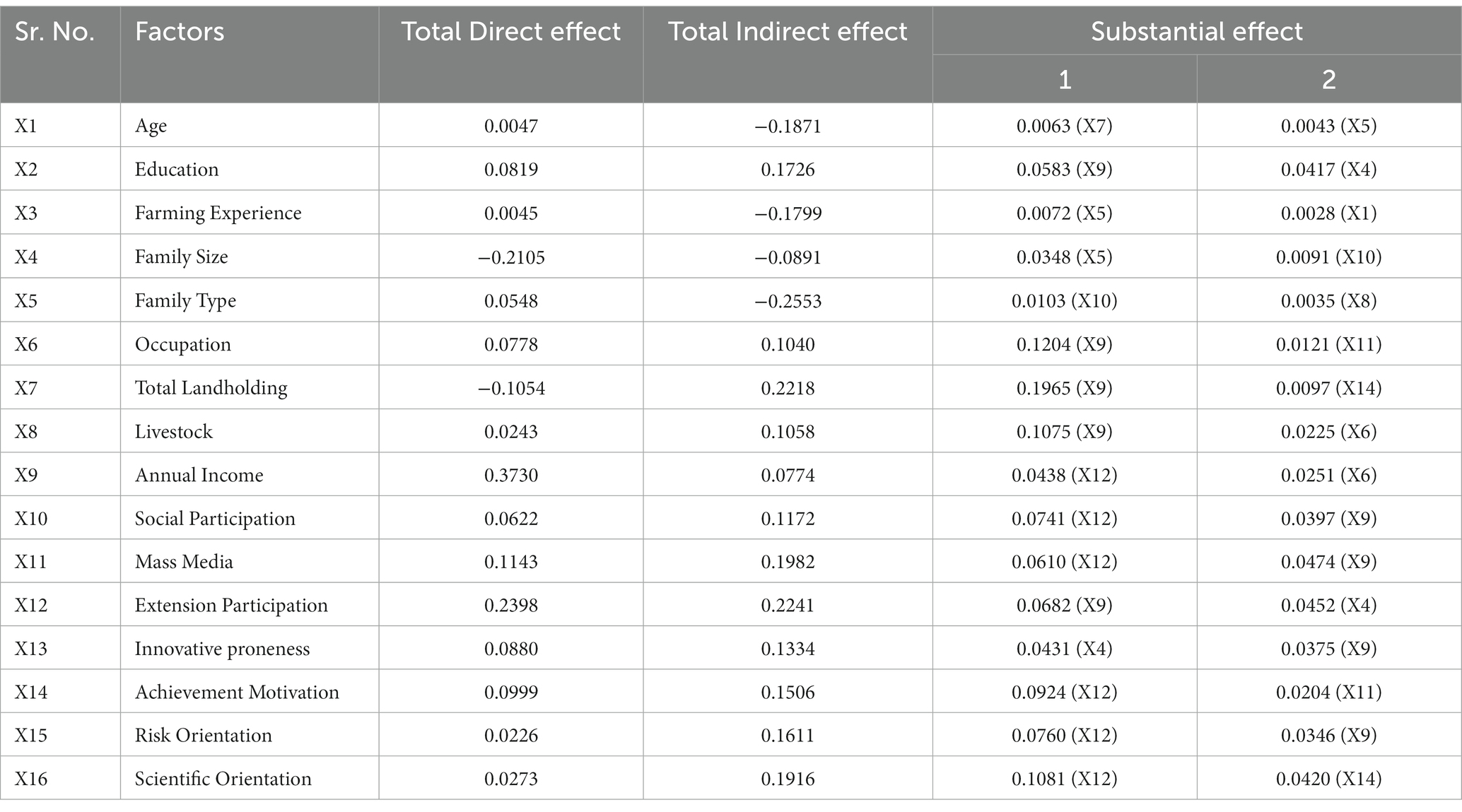
Table 9 . Path effect of a profile of the farmers on the adoption of CSA technologies ( n = 240).
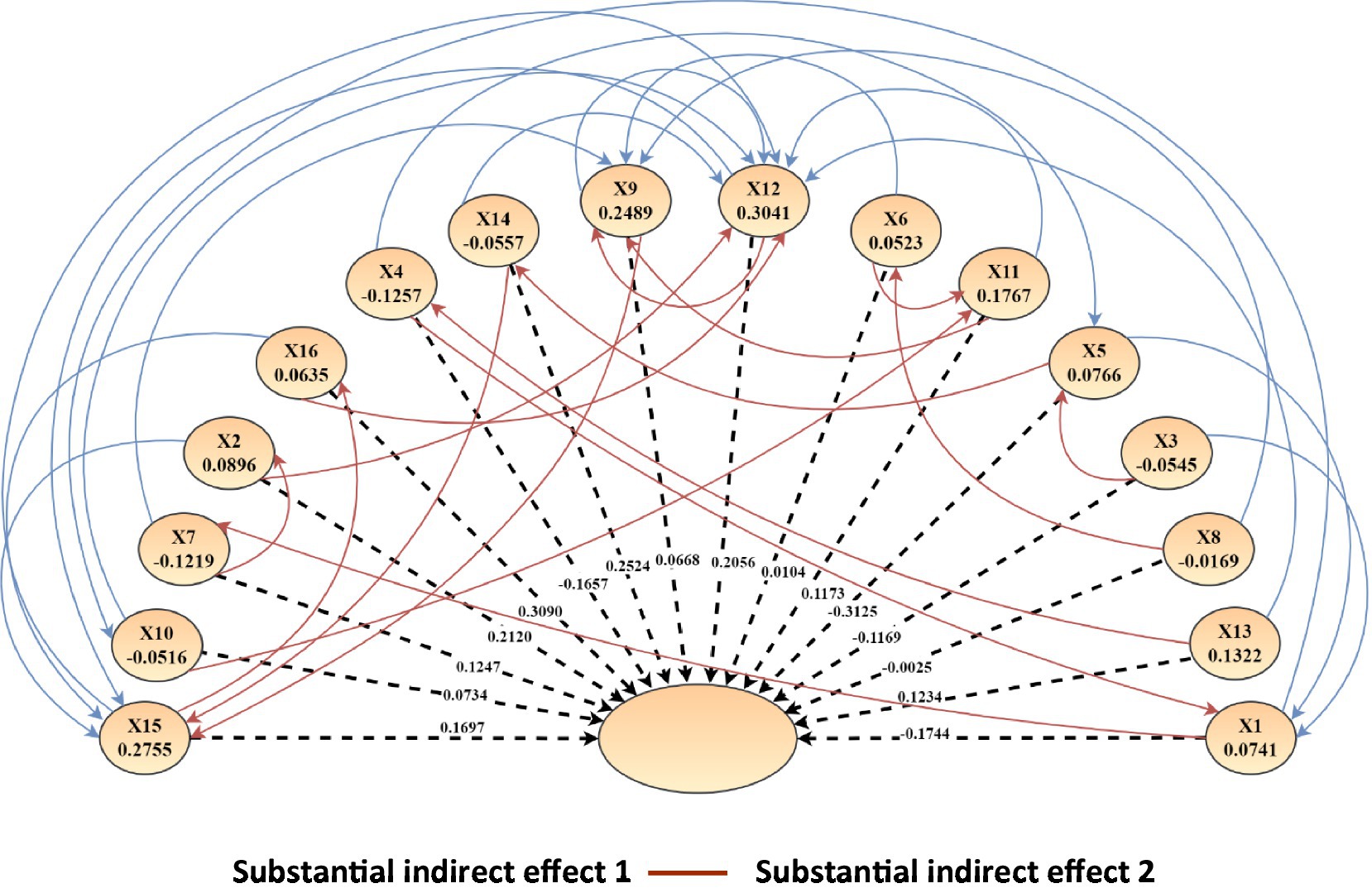
Figure 3 . Direct and Indirect effect of characteristics of the farmers on awareness about CSA technologies.
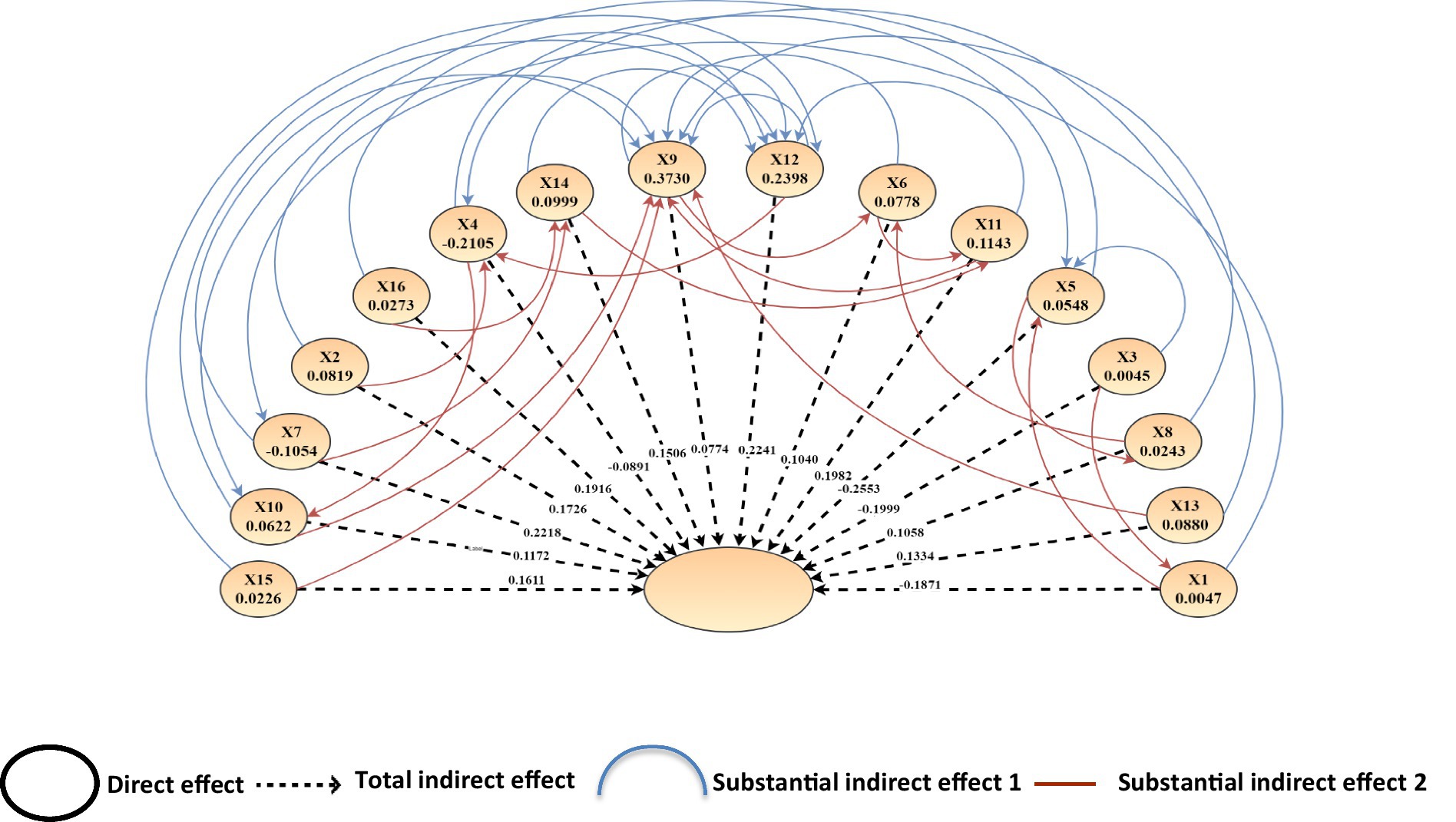
Figure 4 . Direct and Indirect effect of characteristics of the farmers on adoption of CSA technologies.
Tables 8 , 9 ; Figures 3 , 4 also revealed that scientific orientation, achievement motivation, and education were the key factors that had the greatest indirect positive effect on farmers’ awareness of CSA technologies. The adoption of CSA technology was found to have the strongest and, most favourable indirect effects on extension participation, land ownership, and scientific orientation.
The data further indicated that annual income, risk orientation, and scientific orientation had the most significant indirect effects on farmers’ awareness and adoption of CSA technologies ( Nyasimi et al., 2017 ; Tesfaye et al., 2017b ; Kurgat et al., 2020 ). To enhance farmers’ awareness and adoption of CSA technology, it is important to consider the magnitude of the direct and indirect effects of different factors and the mediator role they play. Policymakers and development agencies should prioritize efforts to increase farmers’ involvement in extension programs, improve access to agricultural media, and address income disparities.
Furthermore, promoting scientific orientation and achievement motivation through education and capacity-building initiatives can also have positive indirect effects on farmers’ awareness and adoption levels. Moreover, the path analysis demonstrates that although only a few variables directly influence the dependent variables of awareness and adoption, the overall effect is predominantly driven by the interrelated nature of these variables ( Marenya and Barrett, 2007 ). This highlights the complex and interconnected dynamics involved in shaping farmers’ awareness and adoption of CSA technologies.
Challenges faced by the farmers during the adoption of CSAT
Table 10 revealed that the majority of farmers (85.42%) reported that high input cost as the major restraining factor in the adoption of CSAT, followed by a lack of sufficient knowledge about the CSA technologies (75.42%), youth migration (78.50%), lack of awareness about climate change issues (70%), lack of farmers-friendly CSA technologies. These are the top five significant factors that limit farmers from adopting CSA technologies. Other constraints include the lack of legal and policy frameworks from the government (69.17%), uncertain returns (68.33%), absence of extension activities about CSA technologies (68.33%), lack of knowledge about adaptive practices of CSA (65.83%), poor information dissemination about the technologies (65.42%), non-availability of labour for the adoption of CSAT (65.00%), small landholding (64.58%), lack of access to credit (62.50%), absence of subsidies on planting materials (62.08%), delayed availability of inputs (61.67%), limited marketing access (59.58%), inadequate assistance from national and local authorities on climate-related issues (56.25%), lack of improved communication facilities (54.17%), lack of farmers’ organisations (49.58%), lack of necessary transportation facilities (47.08%), poor supply of uniform electricity (39.58%), and lack of irrigation facilities (39.17%).
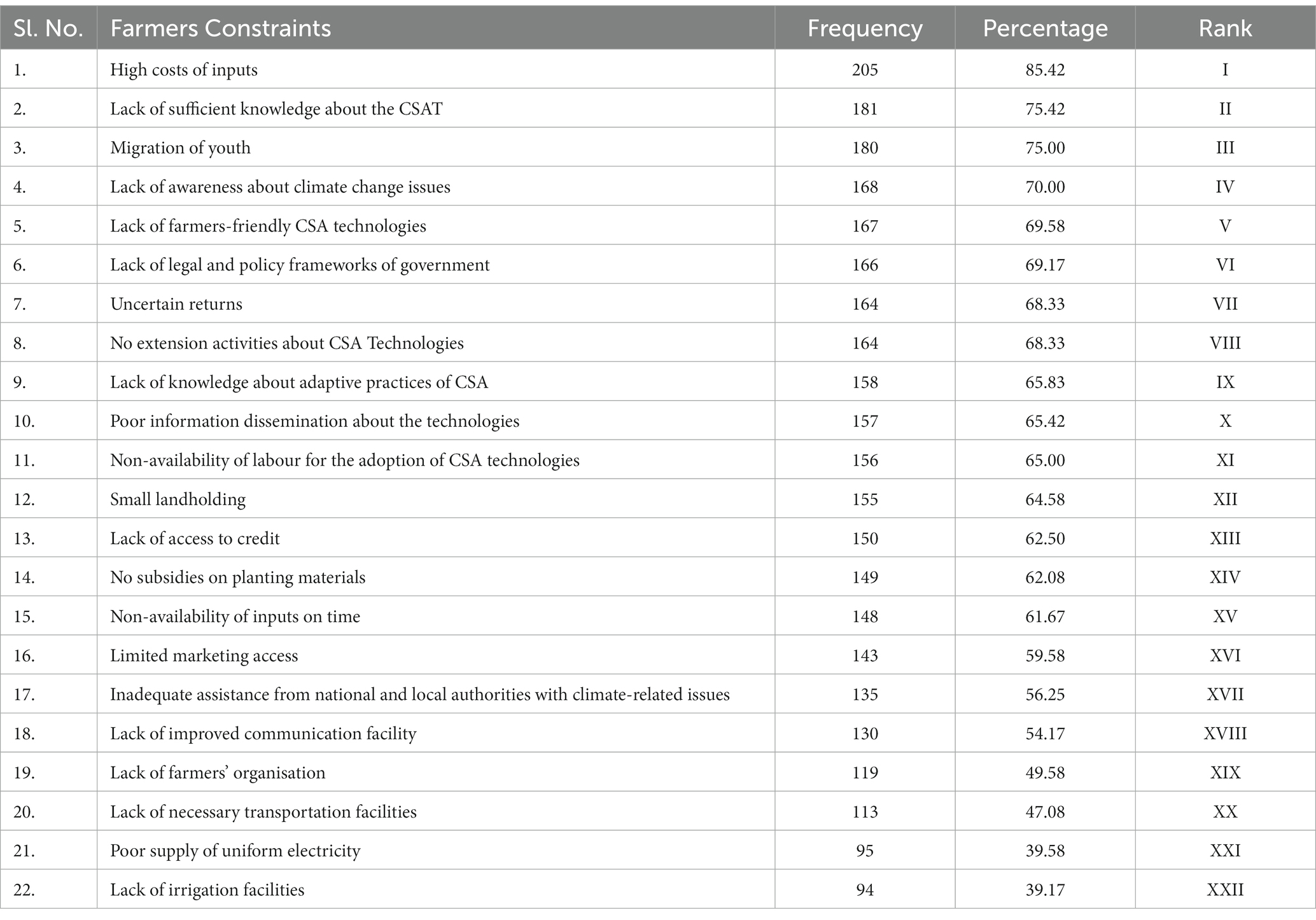
Table 10 . Challenges faced by the farmers in the adoption of CSAT ( n = 240).
These findings are consistent with previous studies conducted by Headey et al. (2014) , Long et al. (2016) , and Tsige et al. (2020) , indicating a consensus on the major constraints faced by farmers in adopting CSA technologies. To address these constraints and promote the adoption of CSA technologies, policymakers and development agencies should focus on several key areas. First, efforts should be made to reduce the input costs associated with implementing CSA practices. This can be achieved through targeted subsidies, access to affordable credit, and the provision of cost-effective CSA technologies.
Second, increasing farmers’ knowledge and awareness of CSA technologies through capacity-building programs, training workshops, and extension services is crucial. Providing farmers with the necessary information and skills empowers them to make informed decisions and overcome barriers related to knowledge gaps ( Ogato, 2014 ).
Third, addressing the issue of youth migration and attracting the younger generation to farming is vital. Creating favorable conditions, such as providing support for agricultural entrepreneurship, improving rural infrastructure, and offering incentives, can encourage youth involvement in farming and increase the adoption of CSA technologies.
Fourth, strengthening legal and policy frameworks related to CSA is essential. Clear regulations, supportive policies, and incentives can create an enabling environment for farmers to adopt sustainable agricultural practices.
Overall, understanding the key constraints reported by farmers in the adoption of CSA technologies is crucial for designing effective interventions. By addressing these barriers, policymakers and development agencies can facilitate the widespread adoption of CSA practices, leading to more resilient and sustainable agricultural systems.
Farmers’ suggestions to improve the adoption of CSAT
The results of Table 11 revealed that the majority of farmers (96.67%) believed that stakeholders should actively be involved in technological development. This was followed by the opinion that development organisations and line departments should ensure the availability of production inputs throughout the cropping season (87.08%). Other important factors mentioned were arranging visits to successful fields (83.75%), providing financial support for adoption and purchase of inputs (81.25%), demonstrating CSA technologies in villages (80.83%), and making improved crop variety seeds available in the village (77.08%).
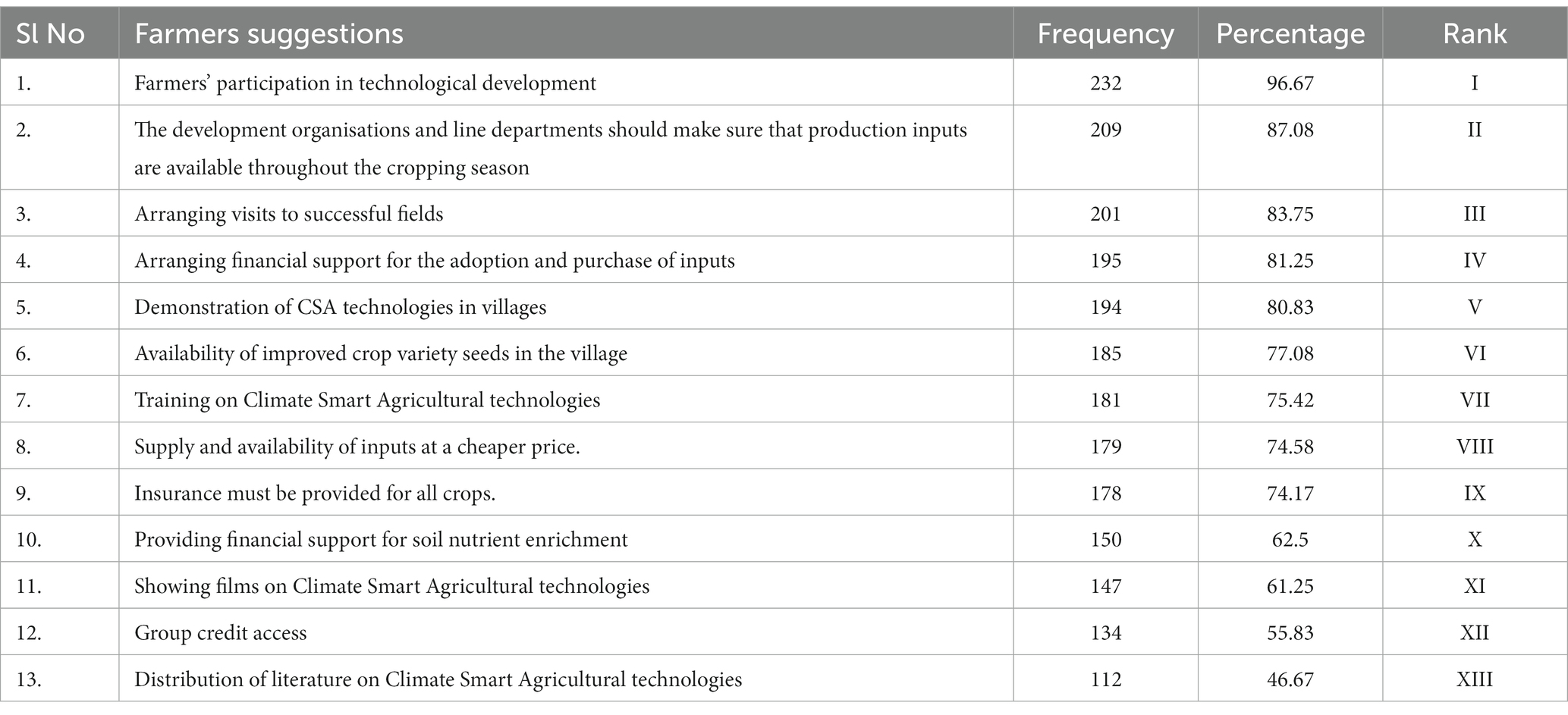
Table 11 . Farmers’ suggestions for greater adoption of CSAT ( n = 240).
These findings align with previous studies conducted by Jirata et al. (2016) , Abera et al. (2020) , and Hariharan et al. (2020) , suggesting a consensus among farmers regarding the importance of stakeholder involvement and the measures needed to promote the adoption of CSA technologies.
To effectively address the farmers’ perspectives and recommendations, it is crucial to raise awareness among the farming community about climate change and the advancements in CSA technologies. Farmers need to be informed and educated about the benefits and practices of CSA and the importance of sustainable land-use practices. Additionally, farmers should be encouraged to actively participate in technology development and decision-making processes, as their insights and experiences are vital for the successful implementation of CSA initiatives. It is particularly important to consider the specific requirements and challenges faced by small, marginal, and resource-poor farmers, who may require additional support and tailored approaches to ensure their inclusion in CSA programs.
1. The majority of farmers in the study area exhibit a high level of awareness and adoption of crop-smart practices, such as short-duration and high-yielding crop varieties, indicating their knowledge of improved agricultural techniques.
2. Farmers show relatively lower awareness and adoption levels in certain areas of climate-smart agriculture, such as energy-smart and weather-smart technologies. Continuous learning about CSAT, climatic information, and agro-advisory services should be prioritised for farmers, financial institutions, and input service providers. This will enhance farmers’ capacity to adapt to climate change while also changing their perspectives on climate-smart farming. Although, our study focused on India, the conclusions drawn can be applicable to other countries that seek to increase agricultural output while minimising the negative impact of climate change.
3. It is evident that governments and other development agencies should prioritize efforts to enhance the profile traits that are significantly linked to farmers’ awareness of CSAT. By focusing on improving education levels, increasing income opportunities, promoting exposure to agricultural media, facilitating participation in extension programs, and fostering characteristics such as innovative proneness, achievement motivation, risk-taking, and scientific orientation, the overall awareness of CSAT among farmers can be significantly improved. Additionally, attention should be given to addressing the negative correlations associated with farming experience, family size, and family type, as these factors hinder farmers’ awareness and need to be carefully considered in awareness-building initiatives.
4. Constraints hindering the adoption of CSA technologies include high input costs, lack of knowledge, youth migration, and limited awareness about climate change issues. Addressing these constraints, along with providing necessary support and resources, can encourage more farmers to adopt climate-smart agriculture practices.
5. Stakeholder involvement, support from development organizations and line departments, and the availability of production inputs are crucial factors for promoting the adoption of CSA technologies. Farmers emphasize the importance of financial support, field demonstrations, and access to improved crop variety seeds to facilitate the adoption process.
6. Principal Component Analysis (PCA) provides insights into the relationship between various factors and the overall variation in awareness and adoption of CSA technologies. Psychological components and economic factors are identified as significant contributors to farmers’ awareness and adoption levels, respectively.
These conclusions highlight the current state of awareness and adoption of climate-smart agriculture technologies among farmers, the factors influencing their decisions, and the constraints they face. By addressing these findings, policymakers and agricultural stakeholders can develop targeted interventions and support mechanisms to promote the widespread adoption of climate-smart agriculture practices.
Data availability statement
The raw data supporting the conclusions of this article will be made available by the authors, without undue reservation.
Ethics statement
Ethical review and approval was not required for the study on human participants in accordance with the local legislation and institutional requirements. Written informed consent for participation was not required for this study in accordance with the national legislation and the institutional requirements.
Author contributions
The conceptualisation and methodology are contributions from VH and TP. VH contributed software, validation, data collection, formal analysis, and written an original draft. TP assisted in review and editing. All authors contributed to the article and approved the submitted version.
This research was funded by Anand Agricultural University, Anand, Gujarat.
Acknowledgments
The authors gratefully acknowledge technical and financial support from the Anand Agricultural University, Anand, Gujarat. The authors are very much thankful to the Gujarat state of India farmers for their cooperation during the survey work.
Conflict of interest
The authors declare that the research was conducted in the absence of any commercial or financial relationships that could be construed as a potential conflict of interest.
Publisher’s note
All claims expressed in this article are solely those of the authors and do not necessarily represent those of their affiliated organizations, or those of the publisher, the editors and the reviewers. Any product that may be evaluated in this article, or claim that may be made by its manufacturer, is not guaranteed or endorsed by the publisher.
Abegunde, V. O., Sibanda, M., and Obi, A. (2019). Determinants of the adoption of climate-smart agricultural practices by small-scale farming households in king cetshwayo district municipality, South Africa. Sustainability 12:195. doi: 10.3390/su12010195
CrossRef Full Text | Google Scholar
Abera, W., Assen, M., and Budds, J. (2020). Determinants of agricultural land management practices among smallholder farmers in the Wanka watershed, northwestern highlands of Ethiopia. Land Use Policy 99:104841. doi: 10.1016/j.landusepol.2020.104841
Aryal, J. P., Rahut, D. B., Maharjan, S., and Erenstein, O. (2018). Factors affecting the adoption of multiple climate-smart agricultural practices in the indo-Gangetic Plains of India. Nat. Res. Forum 42, 141–158. doi: 10.1111/1477-8947.12152
Aryal, J. P., Sapkota, T. E. K. B., Jat, M. L., and Bishnoi, D. K. (2015). On-farm economic and environmental impact of zero-tillage wheat: a case of north-West India. Exp. Agric. 51, 1–16. doi: 10.1017/S001447971400012X
Aryal, J. P., Sapkota, T. B., Khurana, R., Khatri-Chhetri, A., Rahut, D. B., and Jat, M. L. (2020). Climate change and agriculture in South Asia: adaptation options in smallholder production systems. Environ. Dev. Sustain. 22, 5045–5075. doi: 10.1007/s10668-019-00414-4
Ayat, U., Shahab, E. S., and Harald, K. (2022). Determinants of farmers’ awareness and adoption of extension recommended wheat varieties in the Rainfed areas of Pakistan. Sustainability 14:3194. doi: 10.3390/su14063194
Belay, A., Recha, J. W., Woldeamanuel, T., and Morton, J. F. (2017). Smallholder farmers’ adaptation to climate change and determinants of their adaptation decisions in the central Rift Valley of Ethiopia. Agric. Food Secur. 6, 1–13. doi: 10.1186/s40066-017-0100-1
Burke, M., Hsiang, S. M., and Miguel, E. (2015). Global non-linear effect of temperature on economic production. Nature 527, 235–239. doi: 10.1038/nature15725
PubMed Abstract | CrossRef Full Text | Google Scholar
Chand, R., Raju, S. S., and Reddy, A. A. (2015). Assessing performance of pulses and competing crops based on market prices and natural resource valuation. J. Food Legumes 28, 335–340.
Google Scholar
Chandio, A. A., and Yuanshend, J. (2018). Determinants of adoption of improved Rice varieties in. Rice Sci. 25, 103–110. doi: 10.1016/j.rsci.2017.10.003
Djufry, F., Suci, W., and Renato, V. (2022). Climate smart agriculture implementation on coffee smallholders in Indonesia and strategy to accelerate. Land 11:1112. doi: 10.3390/land11071112
FAO (2009). High level expert forum - how to feed the world in 2050. Office of the Director, agricultural development economics division. Economic and social development department. Viale delle Terme di Caracalla, 00153 Rome, Italy.
FAO (2011). Climate change, water and food security. Rome, Italy: Sales and Marketing Group, Information Division, Food and Agriculture Organization of the United Nations. Available at: https://www.fao.org/3/i2096e/i2096e.pdf
FAO (2015a). Climate change and food security: Risks and responses. Rome, Italy: Sales and Marketing Group, Information Division, Food and Agriculture Organization of the United Nations. Available at: https://www.fao.org/3/i5188e/I5188E.pdf
FAO (2015b). The economic lives of smallholder farmers. An analysis based on household data from nine countries. Rome, Italy: Sales and Marketing Group, Information Division, Food and Agriculture Organization of the United Nations.
FAO (2017). Climate-smart crop production. Rome, Italy: Sales and Marketing Group, Information Division, Food and Agriculture Organization of the United Nations. Available at: https://www.fao.org/climate-smart-agriculture-sourcebook/production-resources/module-b1-crops/b1-overview/en/
Feliciano, D. (2019). A review on the contribution of crop diversification to sustainable development goal 1 “no poverty” in different world regions. Sustain. Dev. 27, 795–808. doi: 10.1002/sd.1923
Godfray, H. C. J., Beddington, J. R., Crute, I. R., Haddad, L., Lawrence, D., and Muir, J. F. (2010). Food security: The challenge of feeding 9 billion people. Science 327, 812–818. doi: 10.1126/science.1185383
Gustafson, D., Asseng, S., Kruse, J., Thoma, G., Guan, K., Hoogenboom, G., et al. (2021). Supply chains for processed potato and tomato products in the United States will have enhanced resilience with planting adaptation strategies. Nat. Food 2, 862–872. doi: 10.1038/s43016-021-00383-w
Hariharan, V. K., Mittal, S., Rai, M., Agarwal, T., Kalvaniya, K. C., Stirling, C. M., et al. (2020). Does climate-Smart Village approach influence gender equality in farming households? A case of two contrasting ecologies in India. Clim. Chang. 158, 77–90. doi: 10.1007/s10584-018-2321-0
Hatfield, J. L., Antle, J., Garrett, K. A., Izaurralde, R. C., Mader, T., Marshall, E., et al. (2020). Indicators of climate change in agricultural systems. Clim. Chang. 163, 1719–1732. doi: 10.1007/s10584-018-2222-2
Headey, D., Dereje, M., and Taffesse, A. S. (2014). Land constraints and agricultural intensification in Ethiopia: a village-level analysis of high-potential areas. Food Policy 48, 129–141. doi: 10.1016/j.foodpol.2014.01.008
Hebbsale Mallappa, V. K., and Shivamurthy, M. (2021). Factor influencing fishery-based farmers’ perception and their response to climate-induced crisis management. Environ. Dev. Sustain. 23, 11766–11791. doi: 10.1007/s10668-020-01141-x
Holden, S. T., Fisher, M., Katengeza, S. P., and Thierfelder, C. (2018). Can lead farmers reveal the adoption potential of conservation agriculture? The case of Malawi. Land Use Policy 76, 113–123. doi: 10.1016/j.landusepol.2018.04.048
IPCC (2012). Managing the risks of extreme events and disasters to advance climate change adaptation. A special report of working groups I and II of the intergovernmental panel on climate change [field, C.B., V. Barros, T.F. stocker, D. Qin, D.J. Dokken, K.L. Ebi, M.D. Mastrandrea, K.J. Mach, G.-K. Plattner, S.K. Allen, M. Tignor, and P.M. Midgley (eds.)]. Cambridge university press, Cambridge, UK, and New York, NY, USA, 582 pp.
Jirata, M., Grey, S., and Kilawe, E. (2016). Ethiopia climate-smart agriculture scoping study; FAO: Addis Ababa, Ethiopia, 2016.
Khatri-Chhetri, A., Aryal, J. P., Sapkota, T. B., and Khurana, R. (2016). Economic benefits of climate-smart agricultural practices to smallholder farmers in the indo-Gangetic Plains of India. Curr. Sci. 110, 1251–1256.
Kifle, T., Ayal, D. Y., and Mulugeta, M. (2022). Factors influencing farmers adoption of climate smart agriculture to respond climate variability in Siyadebrina Wayu District, Central highland of Ethiopia. Clim. Serv. 26:100290. doi: 10.1016/j.cliser.2022.100290
Kukal, M. S., and Irmak, S. (2018). Climate-driven crop yield and yield variability and climate change impacts on the U.S. great plains agricultural production. Sci. Rep. 8:3450. doi: 10.1038/s41598-018-21848-2
Kurgat, B. K., Lamanna, C., Kimaro, A., Namoi, N., Manda, L., and Rosenstock, T. S. (2020). Adoption of climate-smart agriculture Technologies in Tanzania. Front. Sustain. Food Syst. 4:55. doi: 10.3389/fsufs.2020.00055
Lobell, D. B., and Gourdji, S. M. (2012). The influence of climate change on global crop productivity. Plant Physiol. 160, 1686–1697.
Long, T. B., Blok, V., and Coninx, I. (2016). Barriers to the adoption and diffusion of technological innovations for climate-smart agriculture in Europe: evidence from the Netherlands, France, Switzerland and Italy. J. Clean. Prod. 112, 9–21. doi: 10.1016/j.jclepro.2015.06.044
Lulia, I. (2012). Climate-smart agriculture produces more food sustainably. International Atomic Energy Agency (IAEA), Division of Public Information. Vienna, Austria: Vienna International Centre. Available at: https://www.iaea.org/newscenter/news/climate-smart-agriculture-produces-more-food-sustainably
Malhi, G. S., Kaur, M., and Kaushik, P. (2021). Impact of climate change on agriculture and its mitigation strategies: a review. Sustainability 13:1318. doi: 10.3390/su13031318
Marenya, P. P., and Barrett, C. B. (2007). Household-level determinants of adoption of improved natural resources management practices among smallholder farmers in western Kenya. Food Policy 32, 515–536. doi: 10.1016/j.foodpol.2006.10.002
McNamara, K. T., Wetzstein, M. E., and Douce, G. K. (1991). Factors affecting peanut producer adoption of integrated pest management. Rev. Agric. Econ. 13, 129–139. doi: 10.2307/1349563
Mehta, Avantika (2019). Drought-Hit Gujarat Has Water For Factories, But Not For Farmers. New Delhi, India: Indiaspend. Available at: https://www.indiaspend.com/drought-hit-gujarat-has-water-for-factories-but-not-for-farmers/
Miheretu, B. A., and Yimer, A. A. (2017). Determinants of farmers’ adoption of land management practices in Gelana sub-watershed of northern highlands of Ethiopia. Ecol. Process. 6:19. doi: 10.1186/s13717-017-0085-5
Ministry of Agriculture and Farmers’ Welfare (2015). Doubling farmers’ income-volume XI. Empowering the farmers through extension. Department of Agriculture, cooperation and farmers’ welfare, Ministry of Agriculture & farmers’ welfare, Government of India. Available at: https://agricoop.gov.in/sites/default/files/DFI%20Volume%2011.pdf
Mota, A. A., Lachore, S. T., and Handiso, Y. H. (2019). Assessment of food insecurity and its determinants in the rural households in Damot Gale Woreda, Wolaita zone, sothern Ethiopia. Agric. Food Secur. 8, 1–11.
Muhammad, A. I., and Marie, J. (2021). Attitudes to climate change adaptation in agriculture – a case study of Öland, Sweden. J. Rural. Stud. 86, 1–15. doi: 10.1016/j.jrurstud.2021.05.024
Mujeyi, A., Mudhara, M., and Mutenje, M. J. (2019). Adoption determinants of multiple climate smart agricultural technologies in Zimbabwe: considerations for scaling-up and out. Afr. J. Sci. Technol. Innov. Dev. 12, 1–12.
Mujeyi, A., Mudhara, M., and Mutenje, M. (2021). The impact of climate smart agriculture on household welfare in smallholder integrated crop–livestock farming systems: evidence from Zimbabwe. Agric. Food Secur. 10:4. doi: 10.1186/s40066-020-00277-3
Murage, A. W., Midega, C. A. O., Pittchar, J. O., Pickett, J. A., and Khan, Z. R. (2015). Determinants of adoption of climate-smart push-pull technology for enhanced food security through integrated pest management in eastern Africa. Food Sec. 7, 709–724. doi: 10.1007/s12571-015-0454-9
Musafiri, C. M., Kiboi, M., Macharia, J., Ng'etich, O. K., Kosgei, D. K., Mulianga, B., et al. (2021). Adoption of climate-smart agricultural practices among smallholder farmers in Western Kenya: do socioeconomic, institutional, and biophysical factors matter? Heliyon 8:e08677. doi: 10.1016/j.heliyon.2021.e0867
Mwungu, C. M., Mwongera, C., Shikuku, K. M., Acosta, M., and Läderach, P. (2018). “Determinants of adoption of climate-smart agriculture Technologies at Farm Plot Level: an assessment from southern Tanzania” in Handbook of climate change resilience . ed. W. L. Filho (Springer International Publishing), 1–15.
Negera, M., Alemu, T., Hagos, F., and Haileslassie, A. (2022). Determinants of adoption of climate smart agricultural practices among farmers in bale-eco region, Ethiopia. Heliyon 8:e09824. doi: 10.1016/j.heliyon.2022.e09824
Nyasimi, M., Kimeli, P., Sayula, G., Radeny, M., Kinyangi, J., and Mungai, C. (2017). Adoption and dissemination pathways for climate-smart agriculture technologies and practices for climate-resilient livelihoods in lushoto, Northeast tanzania. Climate 5, 2–22. doi: 10.3390/cli5030063
Ogato, G. S. (2014). Biophysical, socio-economic, and institutional constraints for production and flow of cereals in Ethiopia. AJHE 3, 51–71.
Ouédraogo, M., Houessionon, P., Zougmoré, R. B., and Partey, S. T. (2019). Uptake of climate-smart agricultural technologies and practices: actual and potential adoption rates in the climate-smart village site of Mali. Sustainability 11:4710. doi: 10.3390/su11174710
Parajuli, R., Thoma, G., and Matlock, M. D. (2019). Environmental sustainability of fruit and vegetable production supply chains in the face of climate change: a review. Sci. Total Environ. 650, 2863–2879. doi: 10.1016/j.scitotenv.2018.10.019
Patel, H. R., Lunagaria, M. M., Karande, B. I., Yadav, S. B., Shah, A. V., Sood, V. K., et al. (2015). Climate change and its impact on major crops in Gujarat. J. Agrometeorol. 17, 190–193. doi: 10.54386/jam.v17i2.1003
Pathak, T. B., Maskey, M. L., Dahlberg, J. A., Kearns, F., Bali, K. M., and Zaccaria, D. (2018). Climate change trends and impacts on California agriculture: a detailed review. J. Agron. 8:25. doi: 10.3390/agronomy8030025
Patle, G. T. (2021). Climate smart water saving techniques for mitigation of climate change impacts in hill agriculture. Available at: https://www.thesangaiexpress.com/Encyc/2021/12/7/GT-PatleClimate-change-expected-to-intensify-the-demands-of-water-use-in-agriculture-as-climate-is.html
Petronilla, M., David, H., and Lyn, P. (2016). Factors contributing to adoption and use of information and communication technologies within research collaborations in Kenya. Inf. Technol. Dev. 22, 84–100. doi: 10.1080/02681102.2015.1121856
Philip, T., and Leslie, L. (2014). How does climate change Alter agricultural strategies to support food security? International food policy research institute (IFPRI). Washington, DC.
Ravi, S. P., and Ridhima, S. (2019). Implementing climate change adaptation: lessons from India’s national adaptation fund on climate change (NAFCC). Clim. Pol. 19, 354–366. doi: 10.1080/14693062.2018.1515061
Raza, A., Razzaq, A., Mehmood, S. S., Zou, X., Zhang, X., Lv, Y., et al. (2019). Impact of climate change on crops adaptation and strategies to tackle its outcome: a review. Plants 8:34. doi: 10.3390/plants8020034
Salerno, J., Stevens, F. R., Gaughan, A. E., Hilton, T., Bailey, K., Bowles, T., et al. (2021). Wildlife impacts and changing climate pose compounding threats to human food security. Curr. Biol. 31, 5077–5085.e6. doi: 10.1016/j.cub.2021.08.074
Scott, S. D., Plotnikoff, R. C., Karunamuni, N., Bize, R., and Rodgers, W. (2008). Factors influencing the adoption of an innovation: an examination of the uptake of the Canadian heart health kit (HHK). Implement. Sci. 3:41. doi: 10.1186/1748-5908-3-41
Shaw, R., Prabhakar, S. V. R. K., and Fujieda, A. (2005). Community level climate change adaptation and policy issues: A case study from Gujarat , India. Graduate School of Global Environmental Studies. Kyoto University, Japan, p 59
Shukla, P.R., Skea, J., Calvo Buendia, E., Masson-Delmotte, V., Pörtner, H. O., Roberts, D. C., et al. (2019). An IPCC special report on climate change, desertification, land degradation, sustainable land management, food security, and greenhouse gas fluxes in terrestrial ecosystems ; IPCC: Geneva, Switzerland, 2019.
Sivabalan, K. C., and Nithila, S. (2018). Farmers’ capacity strengthening and climate advisory services for combating climate change in India. J. Pharmacogn. Phytochem. 7, 179–182.
Sivakumar, M. V. K., and Stefanski, R. (2010). “Climate change in South Asia” in Climate change and food security in South Asia . eds. R. Lal, M. Sivakumar, S. Faiz, A. Mustafizur Rahman, and K. Islam (Dordrecht: Springer)
Smith, P., Bustamante, M., Ahammad, H. H., Clark, H., Dong, H., Elsiddig, E. A., et al. (2014). “Agriculture, forestry and other land use (AFOLU)” in Climate change 2014: Mitigation of climate change. Contribution of working group III to the fifth assessment report of the intergovernmental panel on climate change . eds. O. Edenhofer, R. Pichs-Madruga, Y. Sokona, E. Farahani, S. Kadner, and K. Seyboth, et al. (Cambridge, United Kingdom and New York, NY, USA: Cambridge University Press).
Tama, R. A. Z., Ying, L., Yu, M., Hoque, M. M., Adnan, K. M., and Sarker, S. A. (2021). Assessing farmers’ intention towards conservation agriculture by using the extended theory of planned behavior. J. Environ. Manag. 280:111654. doi: 10.1016/j.jenvman.2020.111654
Tesfaye, K., Kassie, M., Cairns, J., Michael, M., Stirling, C., Tsedeke Abate, M., et al. (2017a). “Potential for scaling up climate smart agricultural practices: examples from sub-saharan africa” in Climate change adaptation in Africa: Fostering resilience and capacity to adapt . eds. W. Filho, B. Simane, J. Kalungu, M. Wuta, P. Munishi, and K. Musiyiwa (Cham, AG: Springer International Publishing), 185–203.
Tesfaye, K., Zaidi, P., Gbegbelegbe, S., Boeber, C., Getaneh, F., Seetharam, K., et al. (2017b). Climate change impacts and potential benefits of heat-tolerant maize in South Asia. Theor. Appl. Climatol. 130, 959–970. doi: 10.1007/s00704-016-1931-6
Thomas, W. H. (2011). The global supply and demand for agricultural land in 2050: a perfect storm in the making? Am. J. Agric. Econ. 93, 259–275. doi: 10.1093/ajae/aaq189
Tiwari, A., Kesarwani, K., Sharma, A., Ghosh, T., Bisht, N., and Punetha, S. (2022). Drought stress in millets and its response mechanism. IntechOpen.
Tran, D., and Goto, D. (2019). Impacts of sustainability certification on farm income: evidence from small-scale specialty green tea farmers in Vietnam. Food Policy 83, 70–82. doi: 10.1016/j.foodpol.2018.11.006
Tsige, M., Synnevåg, G., and Aune, J. B. (2020). Gendered constraints for adopting climate-smart agriculture amongst smallholder Ethiopian women farmers. Sci. Afr. 7:e00250
Vågsholm, I., Arzoomand, N. S., and Boqvist, S. (2020). Food security, safety, and sustainability—getting the trade-offs right. Front. Sustain. Food Syst. 4:16. doi: 10.3389/fsufs.2020.00016
Vinaya Kumar, H. M., Shivamurthy, M., Govinda Gowda, V., and Biradar, G. S. (2017). Assessing decision-making and economic performance of farmers to manage climate-induced crisis in coastal Karnataka (India). Clim. Chang. 142, 143–153. doi: 10.1007/s10584-017-1928-x
Weiskopf, S. R., Rubenstein, M. A., Crozier, L. G., Gaichas, S., Griffis, R., Halofsky, J. E., et al. (2020). Climate change effects on biodiversity, ecosystems, ecosystem services, and natural resource management in the United States. Sci. Total Environ. 733, 137782–137718. doi: 10.1016/j.scitotenv.2020.137782
World Bank (2020). The adaptation principles: 6 ways to build resilience to climate change. Available at: https://www.worldbank.org/en/news/feature/2020/11/17/the-adaptation-principles-6-ways-to-build-resilience-to-climate-change
World Bank (2021). Climate-Smart Agriculture. Available at: https://www.worldbank.org/en/topic/climate-smart-agriculture
Wright, S. (1921). Correlation and Causation. J. Agric. Res. 20, 557–585.
Zhao, C., Liu, B., Piao, S., Wang, X., Lobell, D. B., Huang, L., et al. (2017). Temperature increase reduces global yields of major crops in four independent estimates. Proc. Natl. Acad. Sci. U. S. A. 114, 9326–9331. doi: 10.1073/pnas.1701762114
Keywords: agriculture, climate smart technologies, farmers, path analysis, socio-psychological factors
Citation: Hebsale Mallappa VK and Pathak TB (2023) Climate smart agriculture technologies adoption among small-scale farmers: a case study from Gujarat, India. Front. Sustain. Food Syst . 7:1202485. doi: 10.3389/fsufs.2023.1202485
Received: 08 April 2023; Accepted: 07 July 2023; Published: 26 July 2023.
Reviewed by:
Copyright © 2023 Hebsale Mallappa and Pathak. This is an open-access article distributed under the terms of the Creative Commons Attribution License (CC BY) . The use, distribution or reproduction in other forums is permitted, provided the original author(s) and the copyright owner(s) are credited and that the original publication in this journal is cited, in accordance with accepted academic practice. No use, distribution or reproduction is permitted which does not comply with these terms.
*Correspondence: Vinaya Kumar Hebsale Mallappa, [email protected]
This article is part of the Research Topic
Socio-Economic Evaluation of Cropping Systems for Smallholder Farmers – Challenges and Options

An official website of the United States government
The .gov means it’s official. Federal government websites often end in .gov or .mil. Before sharing sensitive information, make sure you’re on a federal government site.
The site is secure. The https:// ensures that you are connecting to the official website and that any information you provide is encrypted and transmitted securely.
- Publications
- Account settings
- My Bibliography
- Collections
- Citation manager
Save citation to file
Email citation, add to collections.
- Create a new collection
- Add to an existing collection
Add to My Bibliography
Your saved search, create a file for external citation management software, your rss feed.
- Search in PubMed
- Search in NLM Catalog
- Add to Search
Impact, adaptation, and mitigation of climate change in Indian agriculture
Affiliation.
- 1 Indian Council of Agricultural Research, New Delhi, 110001, India. [email protected].
- PMID: 36316531
- DOI: 10.1007/s10661-022-10537-3
Climate change poses serious risks to Indian agriculture as half of the agricultural land of the country is rainfed. Climate change affects crop yield, soil processes, water availability, and pest dynamics. Several adaptation strategies such as heat- and water stress-tolerant crop varieties, stress-tolerant new crops, improved agronomic management practices, improved water use efficiency, conservation agriculture practices and improved pest management, improved weather forecasts, and other climate services are in place to minimize the climatic risks. The agriculture sector contributes 14% of the greenhouse gas (GHG) from the country. Mitigation of GHG emission from agriculture can be achieved by changing land-use management practices and enhancing input-use efficiency. Experiments in India showed that methane emission from lowland rice fields can be reduced by 40-50% with alternate wetting and drying (AWD), growing shorter duration varieties, and using neem-coated urea according to soil health card (SHC) and leaf color chart (LCC). Dry direct-seeding of rice, which does not require continuous soil submergence, can reduce methane emission by 70-75%. Sequestration of carbon (C) in agricultural soil can be promoted with the application of organic manure, crop residues, and balanced nutrients. India has taken several proactive steps for addressing the issues of climate change in agriculture. Recently, it has also committed for reducing GHG emission intensity by 45% by 2030 and achieving net zero emission by 2070. The paper discusses the major impacts of climate change, potential adaptation, and mitigation options and the initiatives of Govt. of India in making Indian agriculture climate-smart.
Keywords: Climate smart agriculture; Conservation agriculture; Greenhouse gases; Soil management; Water management.
© 2022. The Author(s), under exclusive licence to Springer Nature Switzerland AG.
PubMed Disclaimer
Similar articles
- Potential soil organic carbon sequestration vis-a-vis methane emission in lowland rice agroecosystem. Das SR, Nayak BK, Dey S, Sarkar S, Chatterjee D, Saha S, Sarkar D, Pradhan A, Saha S, Nayak AK. Das SR, et al. Environ Monit Assess. 2023 Aug 26;195(9):1099. doi: 10.1007/s10661-023-11673-0. Environ Monit Assess. 2023. PMID: 37632560 Review.
- Mitigating greenhouse gas emissions from irrigated rice cultivation through improved fertilizer and water management. Islam SMM, Gaihre YK, Islam MR, Ahmed MN, Akter M, Singh U, Sander BO. Islam SMM, et al. J Environ Manage. 2022 Apr 1;307:114520. doi: 10.1016/j.jenvman.2022.114520. Epub 2022 Jan 20. J Environ Manage. 2022. PMID: 35066193
- Do soil conservation practices exceed their relevance as a countermeasure to greenhouse gases emissions and increase crop productivity in agriculture? Shakoor A, Dar AA, Arif MS, Farooq TH, Yasmeen T, Shahzad SM, Tufail MA, Ahmed W, Albasher G, Ashraf M. Shakoor A, et al. Sci Total Environ. 2022 Jan 20;805:150337. doi: 10.1016/j.scitotenv.2021.150337. Epub 2021 Sep 15. Sci Total Environ. 2022. PMID: 34543788
- Influence of rice varieties, organic manure and water management on greenhouse gas emissions from paddy rice soils. Win EP, Win KK, Bellingrath-Kimura SD, Oo AZ. Win EP, et al. PLoS One. 2021 Jun 30;16(6):e0253755. doi: 10.1371/journal.pone.0253755. eCollection 2021. PLoS One. 2021. PMID: 34191848 Free PMC article.
- Greenhouse gas emission from rice fields: a review from Indian context. Gupta K, Kumar R, Baruah KK, Hazarika S, Karmakar S, Bordoloi N. Gupta K, et al. Environ Sci Pollut Res Int. 2021 Jun;28(24):30551-30572. doi: 10.1007/s11356-021-13935-1. Epub 2021 Apr 27. Environ Sci Pollut Res Int. 2021. PMID: 33905059 Review.
- Climate change and resilience, adaptation, and sustainability of agriculture in India: A bibliometric review. Baraj B, Mishra M, Sudarsan D, Silva RMD, Santos CAG. Baraj B, et al. Heliyon. 2024 Apr 15;10(8):e29586. doi: 10.1016/j.heliyon.2024.e29586. eCollection 2024 Apr 30. Heliyon. 2024. PMID: 38681622 Free PMC article. Review.
- Social media reporting on agricultural adaptation to climate change in Pakistan: Measures and implications for sustainability. Javed MN, Adnan HM, Hanan MA, Sarmiti NZ, Adeeb H, Khan A, Iftikhar A. Javed MN, et al. Heliyon. 2023 Nov 7;9(11):e21579. doi: 10.1016/j.heliyon.2023.e21579. eCollection 2023 Nov. Heliyon. 2023. PMID: 38027868 Free PMC article.
- Aggarwal, P. K. (2003). Impact of climate change on Indian agriculture. Journal of Plant Biology, 30(2), 189–198.
- Aggarwal, P. K., Roy, J., Pathak, H., Kumar, S. N., Venkateswarlu, B., Ghosh, A. & Ghosh, D. (2021) Managing climatic risks in agriculture. In Discussion Paper Indian Agriculture Towards 2030. Food and Agricultural Organization, Rome (in press).
- Anand, A., Singh M. P., & Nagarajan, S. (2012). Quantification of climate change impacts on crops: field experimentation. In Pathak, H., Aggarwal, P. K. and Singh, S. D. (Eds), Climate change impact, adaptation and mitigation in agriculture: methodology for assessment and applications (pp. xix + 302). Indian Agricultural Research Institute.
- Anant, B., Rai, N., & Rajesh, K. (2015). Grafting tomato on eggplant as a potential tool to improve waterlogging tolerance in hybrid tomato. Vegetable Science, 42(2), 82–87.
- Aryal, J. P., Sapkota, T. B., Stirling, C. M., Jat, M. L., Jat, H. S., Rai, M., Mittal, S., Sutaliya, J. M. (2016). Conservation agriculture-based wheat production better copes with extreme climate events than conventional tillage-based systems A case of untimely excess rainfall in Haryana, India. Agric. Ecosyst. Environ. 233, 325–335.
- Search in MeSH
Related information
- PubChem Compound (MeSH Keyword)
LinkOut - more resources
Full text sources, research materials.
- NCI CPTC Antibody Characterization Program
Miscellaneous
- NCI CPTAC Assay Portal
- Citation Manager
NCBI Literature Resources
MeSH PMC Bookshelf Disclaimer
The PubMed wordmark and PubMed logo are registered trademarks of the U.S. Department of Health and Human Services (HHS). Unauthorized use of these marks is strictly prohibited.
Impact of climate change on Indian agriculture: new evidence from the autoregressive distributed lag approach
- Ud Din, Mohammad Azhar
- Haseen, Shaukat
Climate change constitutes one of the most critical challenges of the contemporary period and can affect various sectors of economies across the globe, the agricultural sector is not an exception. This study aimed to assess the impact of climate change on India's agricultural sector from 1990 to 2020. The autoregressive distributed lag (ARDL) approach was utilized to determine the short-run and long-run relationships between variables such as carbon dioxide emissions, temperature, energy utilization, and fertilizer consumption. The ARDL method and the Johansen and Juselius cointegration test both supported the existence of a significant and long relationship among the selected variables. The estimated short- and long-run findings showed that carbon dioxide emissions (CO 2 ), temperature, and energy consumption affect agricultural yield positively and significantly. These findings have several implications for the Indian economy. With a large population dependent on agriculture, improved productivity can directly impact food security and rural income, consequently leading to the country's overall economic development. Enhanced agricultural output due to these factors may potentially lead to surplus production, allowing India to export more agricultural produce. This can positively impact the country's trade balance and generate revenue through exports.
- Agriculture;
- Climate change;
- CO<SUB>2</SUB>;
- ARDL technique
Login to your account
Change password, your password must have 8 characters or more and contain 3 of the following:.
- a lower case character,
- an upper case character,
- a special character
Password Changed Successfully
Your password has been changed
Create a new account
Can't sign in? Forgot your password?
Enter your email address below and we will send you the reset instructions
If the address matches an existing account you will receive an email with instructions to reset your password

Request Username
Can't sign in? Forgot your username?
Enter your email address below and we will send you your username
If the address matches an existing account you will receive an email with instructions to retrieve your username
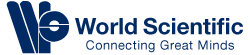
- This Journal
- Institutional Access
Cookies Notification
Our site uses javascript to enchance its usability. you can disable your ad blocker or whitelist our website www.worldscientific.com to view the full content., select your blocker:, adblock plus instructions.
- Click the AdBlock Plus icon in the extension bar
- Click the blue power button
- Click refresh
Adblock Instructions
- Click the AdBlock icon
- Click "Don't run on pages on this site"
uBlock Origin Instructions
- Click on the uBlock Origin icon in the extension bar
- Click on the big, blue power button
- Refresh the web page
uBlock Instructions
- Click on the uBlock icon in the extension bar
Adguard Instructions
- Click on the Adguard icon in the extension bar
- Click on the toggle next to the "Protection on this website" text
Brave Instructions
- Click on the orange lion icon to the right of the address bar
- Click the toggle on the top right, shifting from "Up" to "Down
Adremover Instructions
- Click on the AdRemover icon in the extension bar
- Click the "Don’t run on pages on this domain" button
- Click "Exclude"
Adblock Genesis Instructions
- Click on the Adblock Genesis icon in the extension bar
- Click on the button that says "Whitelist Website"
Super Adblocker Instructions
- Click on the Super Adblocker icon in the extension bar
- Click on the "Don’t run on pages on this domain" button
- Click the "Exclude" button on the pop-up
Ultrablock Instructions
- Click on the UltraBlock icon in the extension bar
- Click on the "Disable UltraBlock for ‘domain name here’" button
Ad Aware Instructions
- Click on the AdAware icon in the extension bar
- Click on the large orange power button
Ghostery Instructions
- Click on the Ghostery icon in the extension bar
- Click on the "Trust Site" button
Firefox Tracking Protection Instructions
- Click on the shield icon on the left side of the address bar
- Click on the toggle that says "Enhanced Tracking protection is ON for this site"
Duck Duck Go Instructions
- Click on the DuckDuckGo icon in the extension bar
- Click on the toggle next to the words "Site Privacy Protection"
Privacy Badger Instructions
- Click on the Privacy Badger icon in the extension bar
- Click on the button that says "Disable Privacy Badger for this site"
Disconnect Instructions
- Click on the Disconnect icon in the extension bar
- Click the button that says "Whitelist Site"
Opera Instructions
- Click on the blue shield icon on the right side of the address bar
- Click the toggle next to "Ads are blocked on this site"

System Upgrade on Tue, May 28th, 2024 at 2am (EDT)
How changes in climate affect crop yields in eastern india.
- SOURYABRATA MOHAPATRA ,
- BASIL SHARP , and
- DUKHABANDHU SAHOO
https://orcid.org/0000-0002-3627-8739
Department of Economics, The University of Auckland, 12 Grafton Road, Auckland 1010, New Zealand
E-mail Address: [email protected]
Corresponding author.
Search for more papers by this author
Department of Economics, The University of Auckland, New Zealand
School of Humanities, Social Sciences and Management, Indian Institute of Technology Bhubaneswar, India
Climate change wreaks havoc on the planet’s ecosystem, affects weather patterns and undermines food security. This paper investigates the effect of changing climate on Indian agriculture, and the climate sensitivity of food grain yield in the eastern region. Using a panel set of physical crop yields and analyzing district-level data from 1990 to 2017, the study estimates agricultural production functions for specific food grains with extrinsic seasonal weather variables. These include average temperature, rainfall, evapotranspiration and wind speed. Spatially disaggregated district-level data provides information from low administrative units, creating a broader picture to be examined. The research indicates that climate change significantly affects east Indian agriculture, and its outcomes are consistent with other state and district-level studies conducted in India. Changing weather patterns over the year considerably influence crop production and disrupt food availability and quality. Hence, the study further expands the implications of results for inter-region inequality and recommends corrective measures.
- Climate sensitivity
- East Indian agriculture
- Food grain yield
- Panel data analysis
- Ashalatha, KV, M Gopinath and ARS Bhat [ 2012 ] Impact of climate change on rainfed agriculture in India: a case study of Dharwad. International Journal of Environmental Science and Development , 3 (4), 368–371. Google Scholar
- ASP (2013). Bay of Bengal — a hotspot for climate insecurity. American Security Project. Google Scholar
- Banerjee, S and B Biswas [ 2020 ] Assessing climate change impact on future reference evapotranspiration pattern of West Bengal, India. Agricultural Sciences , 11 (9), 793–802. Crossref , Google Scholar
- Bhandari, H, S Pandey, R Sharan, D Naik, I Hirway, SK Taunk and ASRAS Sastri [ 2007 ] Economic costs of drought and rice farmers’ drought-coping mechanisms in eastern India . In Economic Costs of Drought and Rice Farmers’ Coping Mechanisms , Sushil PandeyH.S. BhandariB. Hardy (eds.). vol. 32 , International Rice Research Institute. Google Scholar
- Bhatt, B, MS Meena and K Singh [ 2013 ] Status and issues of agriculture in Eastern India . Souvenir Released During Agri-Summit: 2013- A Step Towards Second Green Revolution , 13–6 , 8–9. Google Scholar
- Blasiak, R, J Spijkers, K Tokunaga, J Pittman, N Yagi and H Österblom [ 2017 ] Climate change and marine fisheries: Least developed countries top global index of vulnerability . PLoS One , 12 (6), e0179632. Crossref , Google Scholar
- CDIAC (2011). CO2 emissions from India. Carbon Dioxide Information Analysis Center. Google Scholar
- Chatterjee, S and M Krishnamurthy [ 2021 ] Farm laws versus field realities: understanding India’s agricultural markets. Sikh Research Journal , 6 (1), 20. Google Scholar
- Cline, WR [ 2007 ] Global warming and agriculture: impact estimates by country . Peterson Institute. Google Scholar
- DaMatta, FM, A Grandis, BC Arenque and MS Buckeridge [ 2010 ] Impacts of climate changes on crop physiology and food quality. Food Research International , 43 (7), 1814–1823. Crossref , Google Scholar
- Das, MK and P Mishra [ 2017 ] Impacts of climate change on agricultural yield: evidence from Odisha, India . International Journal of African and Asian Studies , 38 , 58–65. Google Scholar
- Derner, J, J Linda and R Guerrero [ 2015 ] USDA Northern Plains Regional Climate Hub Assessment of Climate Change Vulnerability and Adaptation and Mitigation Strategies . USDA ARS, Natural Resources Research Center . Google Scholar
- Dong, W, C Li, Q Hu, F Pan, J Bhandari and Z Sun [ 2020 ] Potential evapotranspiration reduction and its influence on crop yield in the north China plain in 1961–2014 . Advances in Meteorology , 2020 , e3691421. Crossref , Google Scholar
- Ebi, KL, LO Mearns and B Nyenzi [ 2003 ] Weather and climate: changing human exposures . In Climate Change and Human Health: Risks and Responses , A.J. McMichaelD.H. Campbell-LendrumC.F. CorvalanK.L. EbiA. GithekoJ.D. ScheragaA. Woodward (eds.). World Health Organization. Google Scholar
- Eckstein, D, V Künzel and L Schäfer [ 2021 ] Global climate risk index 2021 . Germanwatch. Google Scholar
- Field, CB and VR Barros [ 2014 ] Climate Change 2014 – Impacts, Adaptation and Vulnerability: Regional Aspects . Cambridge University Press. Crossref , Google Scholar
- Geethalakshmi, V, A Lakshmanan, D Rajalakshmi, R Jagannathan, G Sridhar, AP Ramaraj, K Bhuvaneswari, L Gurusamy and R Anbhazhagan [ 2011 ] Climate change impact assessment and adaptation strategies to sustain rice production in Cauvery basin of Tamil Nadu. Current Science , 101 (3), 342–347. Google Scholar
- Goswami, BN, V Venugopal, D Sengupta, MS Madhusoodanan and PK Xavier [ 2006 ] Increasing trend of extreme rain events over India in a warming environment. Science , 314 (5804), 1442–1445. Crossref , Google Scholar
- Goyal, MK and RY Surampalli [ 2018 ] Impact of climate change on water resources in India. Journal of Environmental Engineering , 144 (7), 04018054. Crossref , Google Scholar
- Gupta, S, P Sen and S Srinivasan (2012). Impact of Climate Change on the Indian Economy-Evidence from Foodgrain Yields. Working Paper No. 218, Centre for Development Economics, Delhi School of Economics. Google Scholar
- Haris, AA, S Biswas and V Chhabra [ 2010 ] Climate change impacts on productivity of rice (Oryza Sativa) in Bihar. Indian Journal of Agronomy , 55 (4), 295–298. Google Scholar
- Hertel, TW and SD Rosch [ 2010 ] Climate change, agriculture and poverty. Applied Economic Perspectives and Policy , 32 (3), 355–385. Crossref , Google Scholar
- Hoechle, D [ 2007 ] Robust standard errors for panel regressions with cross-sectional dependence. Stata Journal , 7 (3), 281–312. Crossref , Google Scholar
- Hollaender, M [ 2010 ] Human right to adequate food: NGOs have to make the difference. CATALYST, Newsletter of Cyriac Elias Voluntary Association (CEVA) , 8 (1), 5–6. Google Scholar
- Howden, SM, J-F Soussana, FN Tubiello, N Chhetri, M Dunlop and H Meinke [ 2007 ] Adapting agriculture to climate change . Proceedings of the National Academy of Sciences , 104 (50), 19691–19696. Crossref , Google Scholar
- Hundal, SS and Prabhjyot-Kaur [ 2007 ] Climatic variability and its impact on cereal productivity in Indian Punjab. Current Science , 92 (4), 506–512. Google Scholar
- Kalra, N, D Chakraborty, A Sharma, HK Rai, M Jolly, S Chander, PR Kumar, S Bhadraray, D Barman, RB Mittal, M Lal and M Sehgal [ 2008 ] Effect of increasing temperature on yield of some winter crops in northwest India. Current Science , 94 (1), 82–88. Google Scholar
- Kapur, D, PB Mehta and R Khosla [ 2009 ] Climate change: India’s options . Economic and Political Weekly , 44 (31), 34–42. Google Scholar
- Kar, J and M Kar [ 2008 ] Environment and changing agricultural practices: evidence from Orissa, India. Indus Journal of Management & Social Sciences , 2 (2), 119–128. Google Scholar
- Karmacharya, J, A Shrestha and ML Shrestha (2007). Climate change scenarios for south Asia and central Himalayan region based on GCM ensemble. Department of Hydrology and Meteorology- Nepal. Google Scholar
- Kaul, S and G Ram [ 2009 ] Impact of global warming on production of jowar in India (special issue: sustainable agriculture in the context of climate change) . Agricultural Situation in India , 66 (5), 253–256. Google Scholar
- Kumar, A, P Sharma and S Joshi [ 2016 ] Assessing the impacts of climate change on land productivity in Indian crop agriculture: an evidence from panel data analysis. Journal of Agricultural Science and Technology , 18 (1), 1–13. Google Scholar
- Kumar, V, Y Sharma and S Chauhan [ 2011 ] Impact of climate change on the growth and production of Saccharum Officinarum and Magnifera Indica. International Journal of Science Technology and Management , 2 , 42–47. Google Scholar
- Kumar, KSK [ 2011 ] Climate sensitivity of Indian agriculture: do spatial effects matter?. Cambridge Journal of Regions, Economy and Society , 4 (2), 221–235. Crossref , Google Scholar
- Kumar, KSK and J Parikh [ 2001 ] Socio-economic impacts of climate change on Indian agriculture . International Review for Environmental Strategies , 2 (2), 277–293. Google Scholar
- Kurukulasuriya, P and R Mendelsohn [ 2008 ] Crop switching as a strategy for adapting to climate change . African Journal of Agricultural and Resource Economics , 2 (1), 105–126. Google Scholar
- Li, D, J Feng, A Dosio, J Qi, Z Xu and B Yin [ 2020 ] Historical evaluation and future projections of 100-m wind energy potentials over CORDEX-east Asia . Journal of Geophysical Research: Atmospheres , 125 (15), e2020JD032874. Crossref , Google Scholar
- Malhi, GS, M Kaur and P Kaushik [ 2021 ] Impact of climate change on agriculture and Its mitigation strategies: A review. Sustainability , 13 (3), 1318. Crossref , Google Scholar
- Masters, G, P Baker and J Flood (2010). Climate change and agricultural commodities. CABI Working Paper No. 2. Google Scholar
- Mishra, D, NC Sahu and D Sahoo [ 2016 ] Impact of climate change on agricultural production of Odisha (India): A Ricardian analysis. Regional Environmental Change , 16 (2), 575–584. Crossref , Google Scholar
- MoEFCC (2021). India: Third biennial update report to the United Nations Framework Convention on Climate Change. Ministry of Environment, Forest and Climate Change, Government of India. Google Scholar
- Nandhini, US, T Alagumani and S Shibi [ 2006 ] Economic analysis of agriculture in southern parts of coastal India. Agricultura Tropica et Subtropica , 39 (4), 254–259. Google Scholar
- Naresh Kumar, S, PK Aggarwal, DN Swaroopa Rani, S Jain, R Saxena and N Chauhan [ 2011 ] Impact of climate change on crop productivity in western Ghats, coastal and northeastern regions of India. Current Science , 101 (3), 332–341. Google Scholar
- Ninan, KN and S Bedamatta (2012). Climate change, agriculture, poverty and livelihoods: a status report. ISEC Working Paper No. 277. Google Scholar
- Padakandla, SR [ 2016 ] Climate sensitivity of crop yields in the former state of Andhra Pradesh, India. Ecological Indicators , 70 , 431–438. Crossref , Google Scholar
- Parry, ML, C Rosenzweig, A Iglesias, M Livermore and G Fischer [ 2004 ] Effects of climate change on global food production under SRES emissions and socio-economic scenarios. Global Environmental Change , 14 (1), 53–67. Crossref , Google Scholar
- Ranuzzi, A and R Srivastava (2012). Impact of climate change on agriculture and food security. ICRIER policy series No. 16. Google Scholar
- Samui, RP, PS Kulkarni, MV Kamble and NG Vaidya [ 2014 ] A critical evaluation of sugarcane yield variation as influenced by climatic parameters in Uttar Pradesh and Maharashtra states of India . Time Journals of Agriculture and Veterinary Sciences , 2 , 63–69, https://scholar.google.com/scholar_lookup? . Google Scholar
- Saseendran, SA, KK Singh, LS Rathore, SV Singh and SK Sinha [ 2000 ] Effects of climate change on rice production in the tropical humid climate of Kerala, India. Climatic Change , 44 (4), 495–514. Crossref , Google Scholar
- Sayari, N, M Bannayan, A Farid, A Alizadeh and MRH Kermani [ 2011 ] Crop water consumption and crop yield prediction under climate change conditions at northeast of Iran . In IPCBEE . Vol. 19 , IACSIT Press. Google Scholar
- Singh, MP [ 2009 ] Rice productivity in India under variable climates . Google Scholar
- Srivastava, A, S Naresh Kumar and PK Aggarwal [ 2010 ] Assessment on vulnerability of sorghum to climate change in India. Agriculture, Ecosystems & Environment , 138 (3), 160–169. Crossref , Google Scholar
- Stern, N [ 2007 ] The Economics of Climate Change: The Stern Review . Cambridge University Press. Crossref , Google Scholar
- Tanaka, K and BC O’Neill [ 2018 ] The Paris agreement zero-emissions goal is not always consistent with the 1 . 5 ° C and 2 ° C temperature targets. Nature Climate Change , 8 (4), 319–324. Crossref , Google Scholar
- Vaidyanathan, A [ 1987 ] Agricultural development in eastern India. Economic and Political Weekly , 22 (52), 2259–2263. Google Scholar
- Verma, S, S Gupta and P Sen (2020). Does climate change make foodgrain yields more unpredictable? Evidence from India. CESifo Working Paper Series No. 8161. Google Scholar
- Wang, Y-M, S Traore and T Kerh [ 2008 ] Computing and modeling for crop yields in Burkina Faso based on climatic data information. WSEAS Transactions on Information Science and Applications , 5 (5), 832–842. Google Scholar
- WMO [ 2017 ] WMO Guidelines on the Calculation of Climate Normals . World Meteorological Organization. Google Scholar
- WMO [ 2021 ] 2020 was One of Three Warmest Years on Record . World Meteorological Organization. Google Scholar
- Wolfe, D, J Beem Miller, L Chambliss, A Chatrchyan and Menninger [ 2014 ] Farming success in an uncertain climate . Cornell University College of Agriculture and Life Sciences. Google Scholar
- Zhai, F, T Lin and E Byambadorj [ 2009 ] A general equilibrium analysis of the impact of climate change on agriculture in the people’s republic of China . Asian Development Review , 26–1 (1), 206–225. Link , Google Scholar
Remember to check out the |
---|
Be inspired by these today. |
- How climate-included variations in crop yields affect migration in India Souryabrata Mohapatra, Amarendra Das, Dukhabandhu Sahoo, Basil Sharp and Auro Kumar Sahoo 29 May 2023 | International Journal of Social Economics, Vol. 50, No. 11
- Heterogeneous climate effect on crop yield and associated risks to water security in India Souryabrata Mohapatra, Dukhabandhu Sahoo, Auro Kumar Sahoo, Basil Sharp and Le Wen 6 September 2023 | International Journal of Water Resources Development, Vol. 10
Recommended
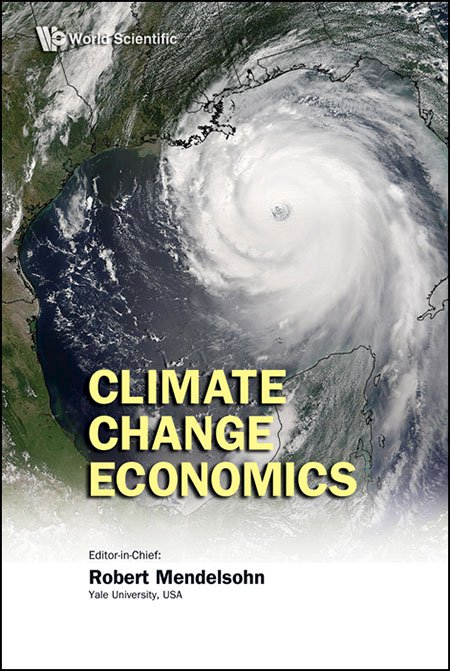
Accepted 10 January 2022 Published: 4 March 2022
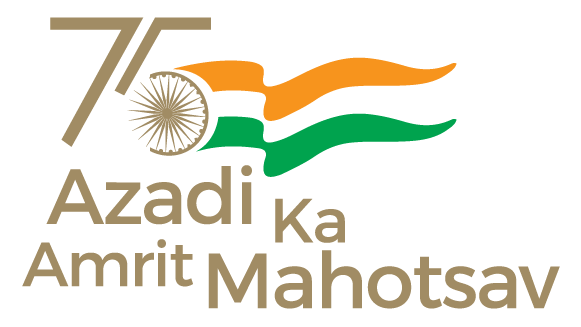
Impact of Climate Change on Agriculture
Government of India is aware about the impact of climate change on agriculture and farmers’ lives. Extensive field and simulation studies were carried out in agriculture by the network centres located in different parts of the country. The climate change impact assessment was carried out using the crop simulation models by incorporating the projected climates of 2050 & 2080. In absence of adoption of adaptation measures, rainfed rice yields in India are projected to reduce by 20% in 2050 and 47% in 2080 scenarios while, irrigated rice yields are projected to reduce by 3.5% in 2050 and 5% in 2080 scenarios. Climate change is projected to reduce wheat yield by 19.3% in 2050 and 40% in 2080 scenarios towards the end of the century with significant spatial and temporal variations. Climate change is projected to reduce the kharif maize yields by 18 and 23% in 2050 and 2080 scenarios, respectively. Climate change reduces crop yields and lower nutrition quality of produce. Extreme events like droughts affect the food and nutrient consumption, and its impact on farmers.
Government of India has formulated schemes/plans to make agriculture more resilient to climate change. The National Mission for Sustainable Agriculture (NMSA) is one of the Missions within the National Action Plan on Climate Change (NAPCC). The mission aims at evolving and implementing strategies to make Indian agriculture more resilient to the changing climate.
To meet the challenges of sustaining domestic food production in the face of changing climate, the Indian Council of Agricultural Research (ICAR), Ministry of Agriculture and Farmers Welfare, Government of India launched a flagship network research project ‘National Innovations in Climate Resilient Agriculture’ (NICRA) in 2011. The project aims to develop and promote climate resilient technologies in agriculture, which addresses vulnerable areas of the country and the outputs of the project help the districts and regions prone to extreme weather conditions like droughts, floods, frost, heat waves, etc. to cope with such extreme events. Short term and long-term research programs with a national perspective have been taken up involving adaptation and mitigation covering crops, horticulture, livestock, fisheries and poultry. The main thrust areas covered are;
(i) identifying most vulnerable districts/regions,
(ii) evolving crop varieties and management practices for adaptation and mitigation,
(iii) assessing climate change impacts on livestock, fisheries and poultry and identifying adaptation strategies. Since 2014, 1888 climate resilient varieties have been developed besides 68 location specific climate resilient technologies have been developed and demonstrated for wider adoption among farming communities.
This information was given by the Union Minister of Agriculture and Farmers Welfare, Shri Narendra Singh Tomar in a written reply in Lok Sabha today.
SNC/PK/3339
Ministry of Agriculture & Farmers Welfare maize yields by 18 and 23% in 2050 and 2080 scenarios, respectively. Climate change reduces crop yields and lower nutrition quality of produce. Extreme events like droughts affect the food and nutrient consumption, and its impact on farmers. Government of India has formulated schemes/plans to make agriculture more resilient to climate change. The National Mission for Sustainable Agriculture (NMSA) is one of the Missions within the National Action Plan on Climate Change (NAPCC). The mission aims at evolving and implementing strategies to make Indian agriculture more resilient to the changing climate. To meet the challenges of sustaining domestic food production in the face of changing climate, the Indian Council of Agricultural Research (ICAR), Ministry of Agriculture and Farmers Welfare, Government of India launched a flagship network research project ‘National Innovations in Climate Resilient Agriculture’ (NICRA) in 2011. The project aims to develop and promote climate resilient technologies in agriculture, which addresses vulnerable areas of the country and the outputs of the project help the districts and regions prone to extreme weather conditions like droughts, floods, frost, heat waves, etc. to cope with such extreme events. Short term and long-term research programs with a national perspective have been taken up involving adaptation and mitigation covering crops, horticulture, livestock, fisheries and poultry. The main thrust areas covered are; (i) identifying most vulnerable districts/regions, (ii) evolving crop varieties and management practices for adaptation and mitigation, (iii) assessing climate change impacts on livestock, fisheries and poultry and identifying adaptation strategies. Since 2014, 1888 climate resilient varieties have been developed besides 68 location specific climate resilient technologies have been developed and demonstrated for wider adoption among farming communities. This information was given by the Union Minister of Agriculture and Farmers Welfare, Shri Narendra Singh Tomar in a written reply in Lok Sabha today.
|
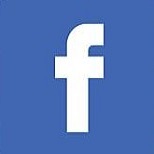
Agricultural Reviews
Chief Editor Pradeep K. Sharma
Print ISSN 0253-1496
Online ISSN 0976-0741
NAAS Rating 4.84
Issue Home |
Online First
Reviewer Article
Study of climate change impact on crops and soil health in india: a review.
- Email [email protected]
Submitted 22-03-2021 |
Accepted 13-06-2022 |
First Online 20-07-2022 |
doi 10.18805/ag.R-2200
- Climate change
- Horticulture crop
- Physical properties
INTRODUCTION

Fig 1: Change in temperature relative to 1850-2018.
Fig 2: Impact of climate change and global warming.
Fig 3: Schematic diagram showing climate change impact on Soil organic carbon loss.
Table 1: Climate change Projection for India (Prasantakumar et al., 2016).
Table 2: Yield reduction by drought in different growth stages in field crops.
Table 3: Abiotic stress susceptible horticultural crops.
CONFLICT OF INTEREST
- Atteya, A.M. (2003). Alteration of water relations and yield of corn genotypes in response to drought stress. Journal of Plant Physiology. 29(1-2): 63-76.
- Basnayake, J., Fukai, S. and Ouk, M. (2006). Contribution of potential yield, drought tolerance and escape to adaptation of 15 rice varieties in rainfed lowlands in Cambodia. In Proceedings of the Australian Agronomy Conference, Australian Society of Agronomy, Birsbane, Australia.1-2.
- Bhardwaj, M.L. (2012). Challenges and opportunities of vegetable cultivation under changing climate scenario. A training manual on vegetable production under changing climate scenario: 13-18.
- Boopen, S. and Vinesh, S. (2011). On the relationship between CO 2 emissions and economic growth: The Mauritian experience. In University of Mauritius, Mauritius Environment Outlook Report, http://www.csae.ox.ac.uk/conferences/ 2011/EDiA/papers/776/Seetanah.pdf (14): 1-25.
- Buol, S.W., Sanchez, P.A., Weed, S.B. and Kimble, J.M. (1990). Predicted Impact of Climatic Warming on Soil Properties and Use 1. Impact of carbon dioxide, trace gases, and climate change on global agriculture. (impactofcarbond). 71-82.
- Chadha, K.L., (2015). Global Climate Change and Indian Horticulture. In Climate Dynamics in Horticultural Science. Impact Adaptation and Mitigation, Eds.; Press, USA. 2: 1-26.
- Davis, J.H.S. (1997). Phaseolous beans. In Wien, H, C. (Ed.), The Physiology of Vegetable Crops. CAB International, Wallingford. UK: 409-428.
- Dawes, M.A., Hagedorn, F., Handa, I.T., Streit, K., Ekblad, A., Rixen, C., et al . (2013). An alpine treeline in a carbon dioxide- rich world: synthesis of a nine-year free-air carbon dioxide enrichment study. Oecologia 171(3): 623-637.
- Deuter, P. (2008). Defining the impact of climate change on horticulture in Australia.Garnaut Climate Change Review, Department of Primary Industries and Fisheries. Queensland.1-23.
- Erickson, A.N. and Markhart, A.H. (2002). Flower developmental stage and organ sensitivity of bell pepper ( Capsicum annuum L.) to elevated temperature. Plant, Cell and Environment, 25(1): pp. 123-130.
- FAO, (2001). Climate variability and change: A challenge for sustainable agricultural production. Committee on Agriculture, Sixteenth Session Report. 26-30 March, 2001. Rome, Italy.
- FAO, (2009). Global agriculture towards 2050 Issues Brief. High level expert forum. Rome: 12-13.
- Geethalakshmi, V., Lakshmanan, A., Rajalakshmi, D., Jagannathan, R., Sridhar, G., Ramara, A. P., Anbhazhagan, R. (2011). Climate change impact assessment and adaptation strategies to sustain rice production in Cauvery basin of Tamil Nadu. Current Science. 101(03): 342-347.
- Guo, Z., Zhang, L., Yang, W., Hau, L., Cai, L., (2019). Aggregate stability under long-term fertilization practices: the case of eroded ultisols of South-Central China. Sustainability 11 (4):1-17.
- Hundal, S.S. and Kaur P. (2007). Climatic variability and its impact on cereal productivity in Indian Punjab. Current Science. 92(4): 506-512
- IPCC (Intergovernmental Panel on Climate Change) (2021). The Physical Science Basis. Contribution of Working Group I to the Sixth Assessment Report of the Intergovernmental Panel on Climate Change [Masson-Delmotte, V., P. Zhai, A. Pirani, S.L. Connors, C. Péan, S. Berger, N. Caud, Y. Chen, L. Goldfarb, M.I. Gomis, M. Huang, K. Leitzell, E. Lonnoy, J.B.R. Matthews, T.K. Maycock, T. Waterfield, O. Yelekçi, R. Yu, and B. Zhou (eds.)]. Cambridge University Press.
- Kamara, A.Y., Menkir, A., Badu-Apraku, B. and Ibikunle, O., (2003). The influence of drought stress on growth, yield and yield components of selected maize genotypes. The Journal of Agricultural Science. 141(1): 43-50.
- Kapur, D., Khosla, R., and Mehta, P. B. (2009). Climate change: India’s options. Economic and Political Weekly. 44(31): 34-42.
- Kar, J. and Kar, M. (2008). Environment and changing agricultural practices: Evidence from Orissa, India. Indus Journal of Management and Social Sciences. 2(2): 119-128.
- Kaul, S. and Ram, G. (2009). Impact of global warming on production of Jowar in India (special issue: sustainable agriculture in the context of climate change). Agricultural Situation in India, 66(5): 253-256.
- Kirschbaum, M.U., (1995). The temperature dependence of soil organic matter decomposition and the effect of global warming on soil organic C storage. Soil Biology and Biochemistry. 27(6): 753-760.
- Kumar, K.S.K. and Parikh, J. (2001b). Indian agriculture and climate sensitivity. Global Environmental Change. 11: 147-154.
- Kumar, K.M., Sridhara, C.J., Hanumanthappa, M. and Marimuthu, S., (2019). A Review of Impacts and Mitigation Strategies of Climate Change on Dryland Agriculture. Current Journal of Applied Science and Technology. pp.1-12.
- Kumar, R. and Kumar, K.K., (2007). Managing physiological disorders in litchi. Indian Horticulture. 52(1): 22-24.
- Kumar, R., Solankey, S.S. and Singh, M. (2012). Breeding for drought tolerance in vegetables. Journal of Vegetation Science. 39(1): 1-15.
- Kumar, S.N., Aggarwal, P.K., Rani, S., Jain, S., Saxena, R. and Chauhan, N., (2011). Impact of climate change on crop productivity in Western Ghats, coastal and northeastern regions of India. Current Science. 101(3): 332-341.
- Lafitte, H.R., Yongsheng, G., Yan, S. and Li, Z.K., (2007). Whole plant responses, key processes, and adaptation to drought stress: the case of rice. Journal of experimental botany. 58(2): 169-175.
- Liverman, D.M. and K. O’Brien. (1991). The impacts of global warming in Mexico. Global Environmental Management. Forthcoming December 1991.
- Malhotra, S.K., (2017). Horticultural crops and climate change: A review. Indian Journal of Agricultural Sciences. 87(1): 12-22.
- Mazahery-Laghab, H., Nouri, F. and ZareAbianeh, H., (2003). Effects of the reduction of drought stress using supplementary irrigation for sunflower ( Helianthus annuus ) in dry farming conditions. Horticulture. 16(4): 81-86.
- Mitran, T., Mishra, U., Lal, R., Ravisankar, T. and Sreenivas, K., (2018). Spatial distribution of soil carbon stocks in a semi- arid region of India. Geoderma Regional. 15: 1-9.
- Monneveux, P., Zaidi, P.H. and Sanchez, C., (2005). Population density and low nitrogen affects yield associated traits in tropical maize. Crop Science. 45(2): 535-545.
- Muthukumar, P. and Selvakumar, R. (2013). Glaustas Horticulture. New Vishal Publications, New Delhi, India. 544-547.
- Nam, N.H., Chauhan, Y.S. and Johansen, C., (2001). Effect of timing of drought stress on growth and grain yield of extra-short- duration pigeonpea lines. Journal of Agricultural Science. 136(2): 179-189.
- Nayyar, H., Kaur, S., Singh, S. and Upadhyaya, H.D., (2006). Differential sensitivity of Desi (small seeded) and Kabuli (large seeded) chickpea genotypes to water stress during seed filling: effects on accumulation of seed reserves and yield. Journal of the Science of Food and Agriculture. 86(13): 2076-2082.
- NOAA Climate.govt, (2020). Reporting on the State of the Climate in 2020. https://www.climate.gov/news-features/understanding-climate/reporting-state-climate-2020.
- Ogbonnaya, C.I., Sarr, B., Brou, C., Diouf, O., Diop, N.N. and Roy Macauley, H., (2003). Selection of cowpea genotypes in hydroponics, pots, and field for drought tolerance. Crop Science. 43(3): 1114-1120.
- Parry, M.L., Carter, T.R. and Konijn, N.T., (1988b). The Impact of Climatic Variations on Agriculture.Volume 1.Assessments in Cool Temperate and Cold Regions. International Institute for Applied Systems Analysis, United Nations Environment Program, Kluwer Academic Publishers, Dordrecht, The Netherlands.
- Parry, M.L., T.R. Carter, and N.T. Konijn. (1988a). The Impact of Climatic Variations on Agriculture.Volume I: Assessments in Cool Temperature and Cold Regions. Kluwer Academic.
- Pathak, H., Aggarwal, P.K. and Singh, S.D. (2012). Climate change impact, adaptation and mitigation in agriculture: methodology for assessment and applications. Indian Agricultural Research Institute. New Delhi:1-302.
- Prasanta Kumar, Andimuthun, B., Ramachandran, Palanivelu, K., Thirumurugan, P., Geetha, R., Bhaskaran, B. (2016). Climate change projections over India by a downscaling approach using PRECIS. Asia-Pac. Journal of Atmospheric Science. 52(4): 353-369.
- Rai, N. and Yadav, D.S. (2005). Advances in Vegetable Production. Researcher Book Center, New Delhi, India.
- Ramulu, M. (1996). Supply response of sugarcane in Andhra Pradesh. Finance India. 10: 116-122.
- Ranuzzi, A., and Srivastava R., (2012). Impact of Climate Change on Agriculture and Food Security. ICRIER policy series 16, New Delhi.
- Ross, D.J., Newton, P.C.D., Tate, K.R. and Luo, D., (2013). Impact of a low level of CO2 enrichment on soil carbon and nitrogen pools and mineralization rates over ten years in a seasonally dry, grazed pasture. Soil Biology and Biochemistry. 58: 265-274.
- Samarah, N.H., (2005). Effects of drought stress on growth and yield of barley. Agronomy for sustainable development. 25(1): 145-149.
- Saseendran, S.A., Singh, K.K., Rathore, L.S., Singh, S.V., and Sinha, S.K. (2000). Effects of climate change on rice production in the tropical humid climate of Kerala, India. Climatic Change. 44(4): 495-514.
- Shetty, P.K., Ayyappan, S. and Swaminathan, M.S. (2013). Climate change and sustainable food security (NIAS Books and Special Publications No.SP4-2013). NIAS; ICAR: 1-340.
- Singh, A. (2012). Impact of sustainable agriculture on food production and challenges for food security in India. Indian Streams Research Journal. 1(5): 1-4.
- Singh, H.P. (2010). Ongoing research in abiotic stress due to climate change in horticulture. Curtain Raiser Meet on Research Needs Arising due to Global Climate Scenario, Baramati, Maharashtra, October. 29-30: 1-23.
- Singh, H.P., Singh, J.P. and Lal, S.S. (2010). Challnges on Climate Change-Indian Horticulture, Westville Publishing House, New Delhi. India: 224.
- Srivastava, A., Kumar, S.N., andAggarwal, P.K. (2010).Assessment on vulnerability of sorghum to climate change in India. Agriculture, Ecosystemsand Environment. 138(3 4): 160-169.
- Stevens, M.A. (1978). Genetic potential for overcoming physiological limitations on adaptability, yield, and quality in the tomato. Horticultural Science. 13: 673-678.
- Veni, V.G., Srinivasarao, C., Reddy, K.S., Sharma, K.L. and Rai, A. (2020). Soil health and climate change. In Climate Change and Soil Interactions: 751-767.
- Yang, D., Kanae, S., Oki, T., Koike, T., and Musiake, K. (2003). Global potential soil erosion with reference to land use and climate changes. Hydrological Process. 17(14): 2913-2928.
- Zhou, X., Xu, X., Zhou, G. and Luo, Y., (2018). Temperature sensitivity of soil organic carbon decomposition increased with mean carbon residence time: Field incubation and data assimilation. Global Change Biology. 24(2): 810-822.
All claims expressed in this article are solely those of the authors and do not necessarily represent those of their affiliated organizations, or those of the publisher, the editors and the reviewers. Any product that may be evaluated in this article or claim that may be made by its manufacturer is not guaranteed or endorsed by the publisher.
This is an open access article distributed under the Creative Commons Attribution License, which permits unrestricted use, distribution, and reproduction in any medium, provided the original work is properly cited.
Reviewed By

In this Article
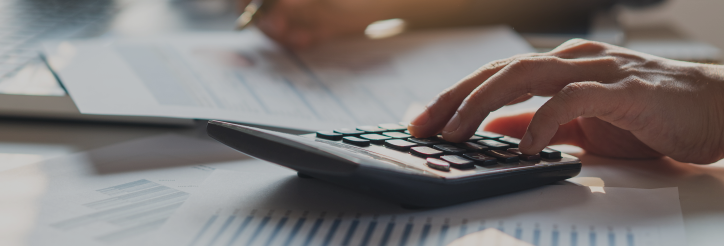
Publish With US
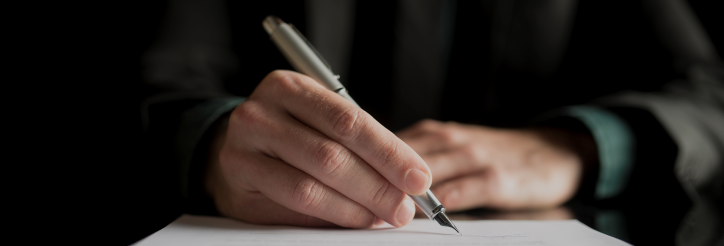
Become a Reviewer/Member
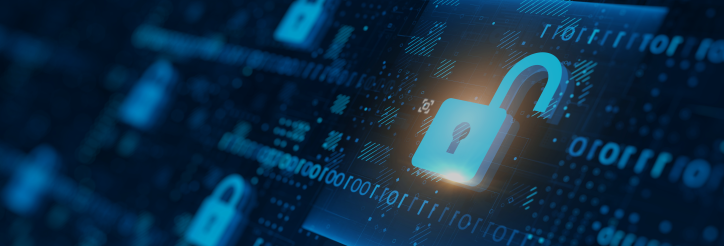
Open Access
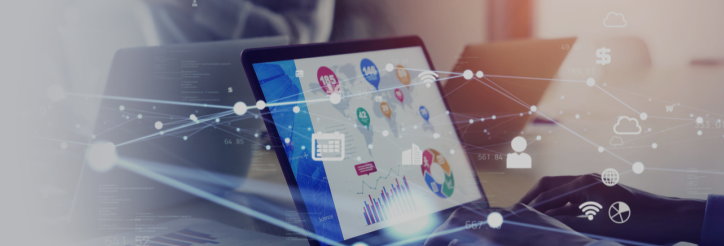
Products and Services
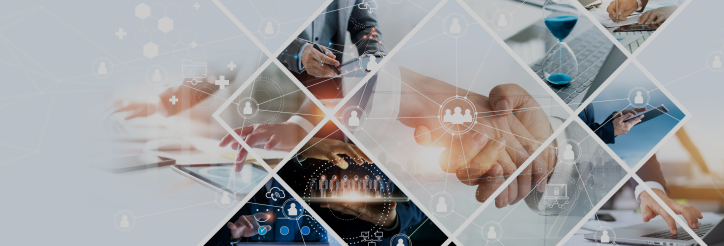
Support and Policies
Editorial board.
- Election 2024
- Entertainment
- Newsletters
- Photography
- Personal Finance
- AP Investigations
- AP Buyline Personal Finance
- AP Buyline Shopping
- Press Releases
- Israel-Hamas War
- Russia-Ukraine War
- Global elections
- Asia Pacific
- Latin America
- Middle East
- Election Results
- Delegate Tracker
- AP & Elections
- Auto Racing
- 2024 Paris Olympic Games
- Movie reviews
- Book reviews
- Personal finance
- Financial Markets
- Business Highlights
- Financial wellness
- Artificial Intelligence
- Social Media
Climate change makes India’s monsoons erratic. Can farmers still find a way to prosper?
A farmer walks as he works in a paddy field on the outskirts of Gauhati, India, July 30, 2021. (AP Photo/Anupam Nath, File)
FILE - A farmer walks as he works in a paddy field on the outskirts of Gauhati, India, July 30, 2021. Human-caused climate change is making rainfall more unpredictable and erratic, which makes it difficult for farmers to plant, grow and harvest crops on their rain-fed fields. (AP Photo/Anupam Nath, File)
- Copy Link copied
Lahuane Rao, an elected head of a village, gazes into the dry well near damaged sugarcane fields due to drought in Beed district, India, Saturday, May 4, 2024. Experts suggest growing crops that need less water. (AP Photo/Rafiq Maqbool)
Damaged cotton plants are visible due to drought in Beed district, India, Saturday, May 4, 2024. Experts suggest growing crops that need less water. (AP Photo/Rafiq Maqbool)
Indian agricultural workers plant paddy saplings at a field after monsoon rains on the outskirts of Lucknow, India, June 30, 2021. Human-caused climate change is making rainfall more unpredictable and erratic, which makes it difficult for farmers to plant, grow and harvest crops on their rain-fed fields. (AP Photo/Rajesh Kumar Singh)
FILE - An Indian farmer wearing a raincoat walks past a paddy field during monsoon rains in Dharmsala, India, July 19, 2021. Human-caused climate change is making rainfall more unpredictable and erratic, which makes it difficult for farmers to plant, grow and harvest crops on their rain-fed fields. (AP Photo/Ashwini Bhatia, File)
FILE - A farmer carries a sack of paddy on his bicycle during monsoon rains as clouds hover over the sky on the outskirts of Guwahati, India, June 20, 2024. Human-caused climate change is making rainfall more unpredictable and erratic, which makes it difficult for farmers to plant, grow and harvest crops on their rain-fed fields. (AP Photo/Anupam Nath, File)

BENGALURU, India (AP) — Each year from June to September, a heavy band of rain makes its way from India’s southwest coast to its northeastern borders, quenching farmers’ thirsty fields.
India’s monsoon season is arguably the single most important weather phenomenon for the country, and a good monsoon can noticeably boost the nation’s economy and the livelihoods of its 120 million farmers. But human-caused climate change is making the rainfall more erratic, making it difficult for farmers to plant, grow and harvest crops on their rain-fed fields.
“Either it rains too much within a short time or it doesn’t rain at all,” said Vijay Jawandhia, a 77-year-old farmer in western Maharashtra state. Jawandhia grows cotton, soya bean and various other crops that require a relatively cool climate and constant irrigation for the first few weeks after sowing. “We planted our cotton seeds after a good monsoon was predicted but it rained just two days and stopped after, so now we’re worried our crops will fail again.”
The Indian Meteorological Department had predicted good rainfall from the monsoon clouds earlier this year, but extreme heat in northern India stalled the rain’s progress. The agency revised its predictions in June, saying the rainfall this year will be less than previously expected.
Many are looking for ways to adapt to this new, unpredictable reality. Experts suggest growing crops that need less water, better and more localized forecasting methods and protection against unexpected weather. But changing centuries-old ways of tending to the land won’t be an easy task.
How is climate change affecting monsoons?
India typically has two monsoons: one from June to September moving southwest to northeast, and another from October to December going the opposite direction.
But with more planet-warming gases in the air, the rain now only loosely follows this pattern. This is because the warmer air can hold more moisture from the Indian Ocean, and that rain then gets dumped all at once. It means the monsoon is punctuated with intense flooding and dry spells, rather than sustained rain throughout.
“When it rains now, it rains heavily,” said Madhavan Rajeevan, a retired senior official at India’s Ministry of Earth Sciences. Rajeevan has tracked the monsoons for decades, and has noticed “the number of rainy days is decreasing even though the total amount of seasonal rainfall has remained the same for the last century or so.”
Landslides and flooding are increasing, he said, alongside high temperatures and longer periods of drought that are adding to farmer’s woes.
The flooding can also result in death and economic losses , such as the hundreds killed and the over $1.42 billion in damages in Himachal Pradesh in 2023 because of heavy monsoon rains.
Rajeevan added that hydropower resources that generate large amounts of electricity are also built with sustained rains in mind, and extreme rain and floods can lead to health issues such as increased cases of typhoid, cholera and malaria.
An Indian farmer wearing a raincoat walks past a paddy field during monsoon rains in Dharmsala, India, July 19, 2021.(AP Photo/Ashwini Bhatia, File)
What does this mean for farmers?
The erratic rain is a significant blow to their livelihood.
Maharashtra has witnessed thousands of farmers dying by suicide that many say is a result of agriculture-related debt. “Our region has become infamous for this,” said Jawandhia, the farmer.
Farmers in traditionally resource-rich regions, such as Punjab and Haryana in northern India, also say they are adversely affected by both fewer rainy days and too much rain when it pours.
Tezveer Singh, a farmer in Ambala city in Haryana remembers how “entire towns and fields were flooded, hundreds of cattle died due to drowning and three people lost their lives” there in last year’s flooding.
Singh grows rice, potatoes and sugarcane on his 20-acre farm and said urgent policy changes are needed to stop flood damage.
He suggested that officials can “give compensation for our losses when needed, provide climate-resilient seeds, make supply chains for farm produce more efficient and increase minimum prices for crops.”
“The climate has become tough and we need to adapt,” he said.
Indian agricultural workers plant paddy saplings at a field after monsoon rains on the outskirts of Lucknow, India, June 30, 2021. (AP Photo/Rajesh Kumar Singh)
How can they adapt?
India’s weather agency makes state-level monsoon rain predictions for the entire country, but climate experts say forecasts need to be more localized in order to be useful to farmers.
Vishwas Chitale, who leads the climate resilience team at the New Delhi-based Council on Energy, Environment and Water, said making localized weather predictions and changing the times of year farmers plant their crops accordingly can help.
In many places across India, “maximum rainfall occurs in October now and not really June and July as it used to,” said Chitale, who also co-authored a 2024 report looking at India’s changing monsoon patterns. “This results in a lot of crops that are waiting to be harvested getting damaged.”
He added that it’s important that better forecasting is available for everyone across the country who needs it.
Some farmers are already adapting to a warmer world. In southern Kerala state, an organic farming collective has begun altering when they sow and harvest plants according to shifting rain patterns. The farming collective has also drawn up an agriculture calendar that factors in climate change that they share with other local farmers.
“The preparedness helps farmers,” said Rajesh Krishnan, a paddy farmer who’s part of the collective that worked with local weather officials on the forecasts. Krishnan said their daily and weekly forecasts have an accuracy of at least 70%. “This is helping cut losses and also get a better crop. The forecasts are also helping us decide when to harvest our crops,” he said.
A farmer carries a sack of paddy on his bicycle during monsoon rains as clouds hover over the sky on the outskirts of Guwahati, India, June 20, 2024. (AP Photo/Anupam Nath)
Climate experts like Rajeevan said the collective’s model needs to be replicated across the country to allow farmers to work with the changing monsoons.
After all, he said, “monsoons are a part of our culture. We cannot think of India without monsoons.”
Follow Sibi Arasu on X at @sibi123
The Associated Press’ climate and environmental coverage receives financial support from multiple private foundations. AP is solely responsible for all content. Find AP’s standards for working with philanthropies, a list of supporters and funded coverage areas at AP.org .
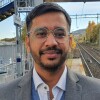

An official website of the United States government
Here’s how you know
Official websites use .gov A .gov website belongs to an official government organization in the United States.
Secure .gov websites use HTTPS A lock ( Lock A locked padlock ) or https:// means you’ve safely connected to the .gov website. Share sensitive information only on official, secure websites.
India: Climate Change - Agriculture and Policy in India
Link to report:.
India’s agricultural sector is highly vulnerable to the effects of climate change and extreme weather events. With a rapidly growing population and limited natural resource base, India’s grain and livestock sectors contribute to significant global greenhouse gas emissions, yet the country’s diverse agricultural systems offer significant ecological benefits that can improve resiliency to climate change impacts. India’s climate policies have been consistent over the years and continue to emphasize climate change adaptation and mitigation efforts through domestic growth and economic development. As the growth center for energy demand the next two decades, India maintains its goal to install 450 gigawatts of renewable energy by 2030, while prioritizing climate adaption policies and supporting rural, agricultural livelihoods.
Database Source:

Global Agricultural Information Network (GAIN)
Related reports, philippines: confectionery and ice cream market brief, japan: japan proposes 29 additional gi protections for uk food items, mexico: exporter guide annual, get reports, news, and more delivered straight to your inbox.
- Important Notices
- GBI Secure Login

- Program and Exams
- Fees and Payments
- Our FRM Certified Professionals
- Study Materials
- Exam Logistics
- Exam Policies
- Risk Career Blog
- Register for FRM Exam
- Path to Certificate
- Climate Resource Center
- Register for SCR Exam
- Risk&AI
- Foundations of Financial Risk (FFR)
- Financial Risk and Regulation (FRR)
- About Membership
- Exclusive Offers
- Risk Intelligence
- Board of Trustees
- GARP Benchmarking Initiative (GBI)
- GARP Risk Institute
- Buy Side Risk Managers Forum
- Academic Partners
- Careers at GARP
- Culture & Governance
- Sustainability & Climate
- Operational
- Comment Letters
- White Papers
- Islamic Finance Book
Physical Risk - Risk Management - Transition Risk
India: A Case Study in Climate Mitigation and Adaptation
This article explores the difficult trade-offs that need to be made between the competing claims of climate mitigation, adaptation, and economic development..
Thursday, September 14, 2023
By Maxine Nelson
This article has been extensively updated, incorporating new COP 27 commitments, Reserve Bank of India (RBI) statements and current green bond issuance. It was originally published Oct. 18, 2021.
After decades of population growth and economic development, India is now the third largest emitter of greenhouse gases in the world. In addition, India is among the countries most vulnerable to climate change due to its geography and dependence on agriculture.
It has been estimated that if emissions are not significantly reduced, India could suffer economic losses of USD 35 trillion . Indeed, much of India has been experiencing annual heatwaves followed by intense flooding, and in 2021 alone it experienced even more extreme weather events — including cyclones and a glacier collapse. Thus, India makes a thought-provoking case study for policymakers and risk professionals given the difficult trade-offs that need to be made between the competing claims of climate mitigation, adaptation and economic development.
Climate Change’s Effect on India
The banking regulator, Reserve Bank of India (RBI) , explains that “India has witnessed changes in climatic patterns in line with the rest of the world… the rainfall pattern, particularly with respect to the [south west monsoon] SWM, which provides around 75 percent of the annual rainfall, has undergone significant changes. Moreover, the occurrence of extreme weather events like floods/unseasonal rainfall, heat waves and cyclones has increased during the past two decades, and data reveal that some of the key agricultural states in India have been the most affected by such events.”
A more recent, detailed RBI study points out that “it is the increased frequency of extreme weather occurrences that is breaking the back of our capability to cope with natural disasters.” As shown by India’s nationally determined contributions (NDCs) — the actions it has committed to take to reduce its emissions and adapt to the impacts of climate change — it is among the most vulnerable countries in the world to the impact of accelerated sea level rise from global warming. This is due to its long coastline, large number of islands and population of 170 million living in coastal regions.
The RBI also notes that precipitation and temperature — the two key climate indicators — “play a crucial role in the overall health of the Indian economy.” As well as affecting food production, the extreme weather in agricultural states impacts employment and GDP, with approximately 44% of the working population employed in agriculture and allied sectors which contribute about 20% of GDP, according to M.K. Jain, the deputy governor of the Reserve Bank . Several challenges confronting Indian agriculture, including diminishing and degrading natural resources and unprecedented climate change, need to be tackled for the long-term sustainability and viability of Indian agriculture.
However, there is uncertainty over how large the impacts might be. The Swiss Re Institute , for example, estimates a 35% reduction in the level of India’s GDP by 2050 if greenhouse gas emissions are not reduced globally, and approximately a 6% GDP reduction even if the Paris Agreement goals are met. An Oxford Economics report “Estimating the Economic Impact of Global Warming” has framed the impact differently, estimating that India’s GDP could be 90% lower in 2100 than it would be if there was no climate change, suggesting that climate change has the potential to absorb all of India’s future prospective growth in income per capita. And Deloitte has estimated USD 35 trillion of economic losses by 2070. While these different approaches produce diverse estimates, they all show that the impact will be big and require additional investments in both mitigation and adaptation.
India’s Effect on Climate Change
Not only will the changing climate have a significant impact on India, but India is also expected to have a significant impact on the climate. Although historically it has not had high emissions, India rose to the number three spot in the national emissions rankings 15 years ago, behind China and the U.S. The RBI noted that “With the increase in population, the cumulative level of greenhouse gas (GHG) emissions has increased, resulting in a rise of average temperature. According to a study by the International Energy Agency (IEA), India emitted 2,299 million tonnes of carbon dioxide (CO 2 ) in 2018, a rise of 4.8% over the previous year.”
Unfortunately, India’s future potential emissions are not yet aligned with the Paris Agreement goals. India’s NDCs currently correspond to temperature increases above 3°C, according to Climate Action Tracker . (You can find out more about NDCs and their place in the Paris Agreement in this short article . ) India increased its commitment to reduce greenhouse gas emissions at COP 26, the 2021 annual meeting of the signatories of the Paris Agreement, where it pledged to cut its emissions to net zero by 2070. While this was a large increase in commitment, it isn’t yet aligned with the worldwide goal of cutting emissions to net zero by 2050 needed to limit global warming to 1.5°C.
Maxine Nelson
In advance of COP 27, India has again increased its commitment and pledged to a 45% reduction in GDP emissions intensity by 2030 — marking an 10% increase from the previous pledge. Any emissions reduction is helpful to mitigate climate change. However, as the pledge is based on emissions intensity and not absolute emissions, emissions can continue increasing as the economy expands. This pledge, therefore, doesn’t meet the net-zero goal of reducing emissions by 45% by 2030. Still, the effort required to overcome the challenge of rapidly expanding an economy while decreasing emissions intensity needs to be appreciated.
To further mitigate climate change, India may need to agree to reduce its emissions even more — a big task for a developing economy with average annual energy consumption of a third the global average, and per capita emissions already 10 times lower than that of the U.S., four times lower than China, and three times lower than Europe. With IPCC reports highlighting the urgency of tackling climate change quickly to reduce the loss and damage for humans and ecosystems, it is even more important that emissions reductions are ambitious.
Financing Mitigation and Adaptation
A 2021 RBI Financial Stability Report noted that climate change and the associated mitigating policy commitments are “set to reshape the macroeconomic and financial landscape”. Extensive funding is needed both to reduce future emissions and to finance the adaptation needed to manage the impacts of climate change. In their 2016 NDC, India estimated that at least USD 2.5 trillion (at 2014-15 prices) would be required for meeting its climate change actions between 2016 and 2030. And the International Energy Agency estimates that nearly 60% of India’s CO 2 emissions in the late 2030s will be coming from infrastructure and machines that do not exist today. If this investment is to be sustainable, USD 1.4 trillion extra funding (above that required for current policies) is needed over the next 20 years.
Like most of the world, green bond issuance in India — which could provide some of this funding — is currently a small proportion of all bond issuance. The rate of issuance is increasing, however, with USD 21.6 billion of green, sustainable or social bonds issued in 2022. And in 2023, the Government of India entered the green finance market issuing USD 2 billion of green bonds to finance their spending on a range of projects including solar power, green hydrogen and afforestation. As they obtained a greenium (lower financing costs than other equivalent bonds), we should expect to see more of these issued in the future.
There are also substantial opportunities in other financial markets, such as the development of a derivatives market to aid adaptation via products such as:
- agricultural commodity derivatives, which can help reduce risks by enabling continuous price discovery and providing hedging
- weather derivatives, which can hedge the risks of high-probability, low-risk events
Of course, meeting the needs of climate change financing carries the usual financial risk implications of any lending. An RBI analysis shows that banks’ direct exposure to fossil fuels (through electricity, chemicals and cars) is 10% of total outstanding non-retail bank credit, so it should have a limited impact on the banking system. However, it notes that many other industries indirectly use fossil fuels and their impacts also need to be closely monitored.
Regulatory Response
The RBI has noted that policy measures such as a deepening of the corporate bond market, standardization of green investment terminology, consistent corporate reporting and removing information asymmetry between investors and recipients can make a significant contribution in addressing some of the shortcomings of the green finance market.
Like in most of the rest of the world, there is an increasing regulatory focus on climate risk. The RBI Governor has stated that guidelines will be issued about disclosure of climate-related risks, and also scenario analysis and stress testing. This followed last year’s RBI consultation which asked for inputs on a comprehensive range of topics from climate risk governance to strategy, and risk monitoring, management and mitigation at regulated entities. This consultation, in turn, built on the results of an RBI survey of banks that was also published last year. The survey found that “although banks have begun taking steps in the area of climate risk and sustainable finance, there remains a need for concerted effort and further action in this regard.” It also found that board-level engagement is inadequate, and few banks had a strategy for incorporating climate risk into their risk management framework. To see what leading climate risk firms are doing globally look at GARP’s whitepaper: “ Climate Risk Leadership: Lessons From 4 Annual Surveys .”
Given the widespread impact of climate change, it isn’t just the banking regulator that is looking at how climate risk will affect firms in its jurisdiction. In 2021, the Securities and Exchange Board of India (SEBI) mandated that the largest 1,000 listed firms complete a Business Responsibility and Sustainability Report . The report asks for information like material ESG risks and opportunities and their financial implications; sustainability related targets and performance; and their greenhouse gas emissions. Companies’ value chains also need to be assessed. This requirement is being progressively rolled out from 2023 to 2027, with the largest companies also required to get assurance of their disclosures.
In addition, SEBI has altered the rules for mutual funds , allowing them to have multiple ESG schemes with different strategies; in the past, a mutual fund could only have one ESG fund. This increase in scope follows one for green debt securities , which was expanded to include bonds such as blue bonds (sustainable water management and marine sector), yellow bonds (solar energy generation and transmission), transition bonds and adaptation bonds. Both of these expansions in scope should increase financing for sustainability related initiatives.
Reflecting the fact that addressing climate change is a global problem, needing both local and global solutions, the RBI joined the Network for Greening the Financial System (NGFS) in April 2021. The NGFS’s purpose is to strengthen the global response required to meet the goals of the Paris Agreement and to enhance the role of the financial system to manage risks and to mobilize capital for green and low-carbon investments. These goals align very well with the work India needs to undertake to make not just its financial system resilient to the risks from climate change, but to balance mitigation, adaptation, and economic development across the country.
Maxine Nelson , Ph.D, Senior Vice President, GARP Risk Institute, currently focusses on sustainability and climate risk management. She has extensive experience in risk, capital and regulation gained from a wide variety of roles across firms including Head of Wholesale Credit Analytics at HSBC. She also worked at the U.K. Financial Services Authority, where she was responsible for counterparty credit risk during the last financial crisis.
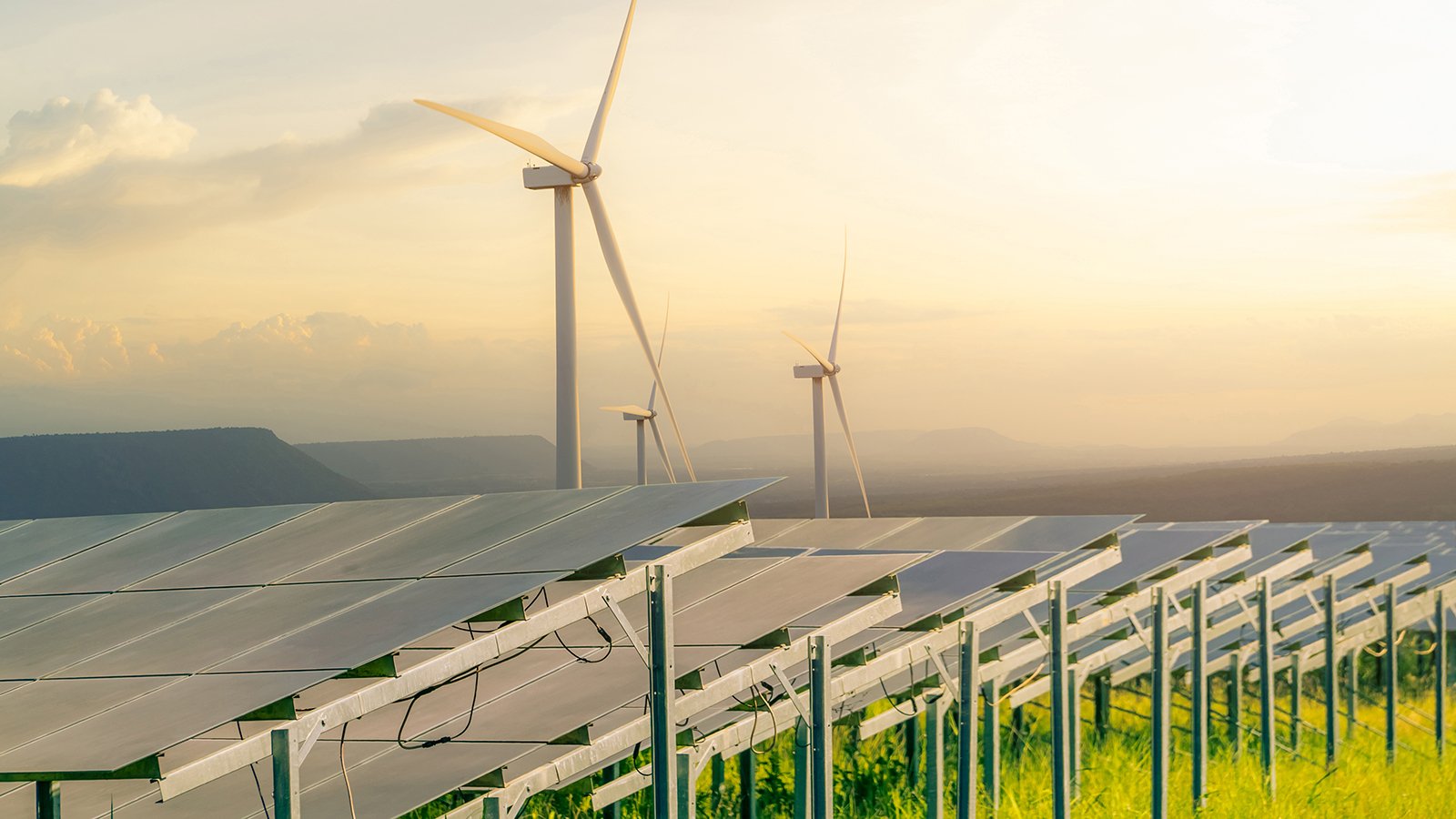
ESG Explained: What It Is and Why It Matters Jun 30, 2023
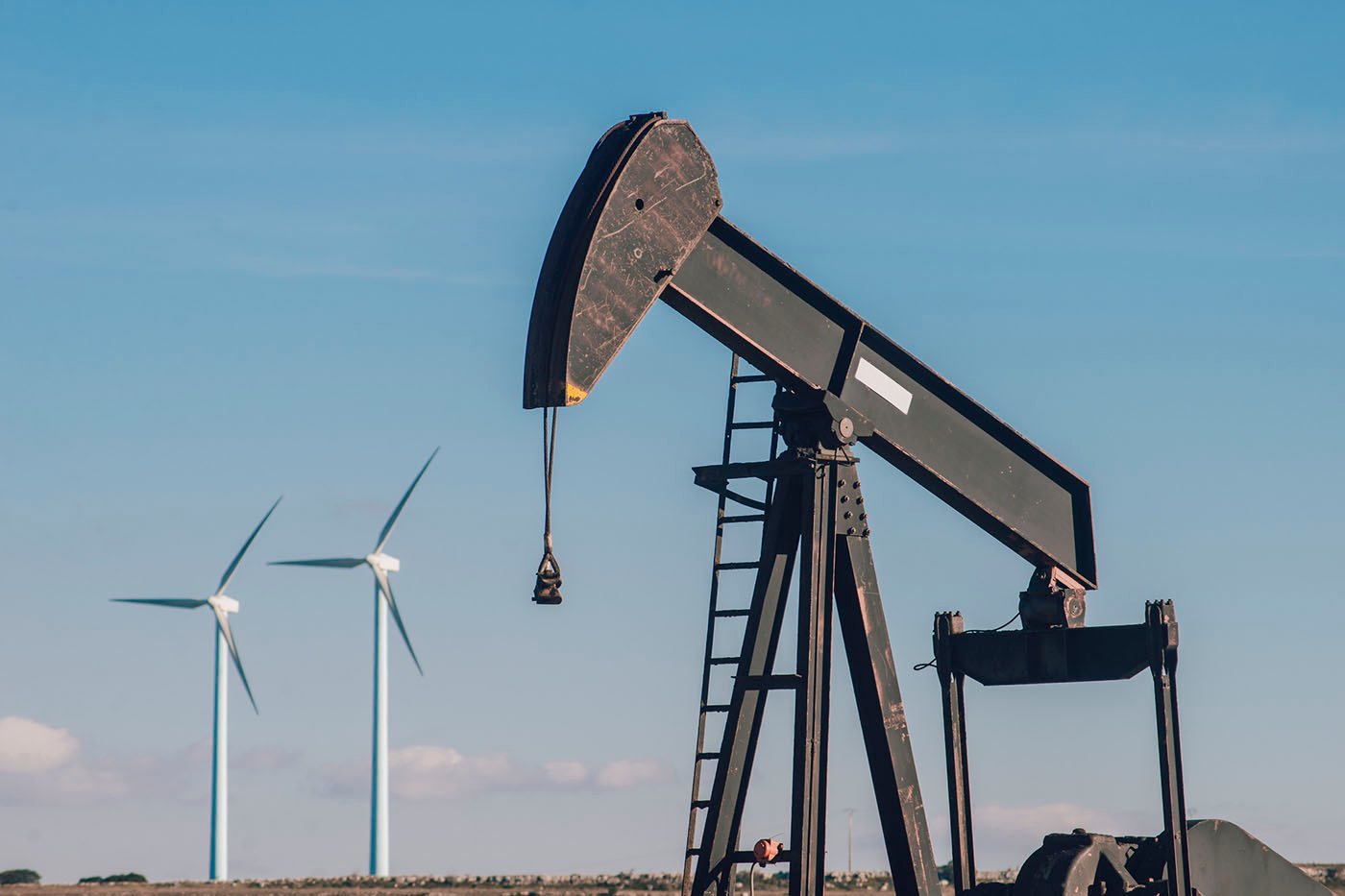
The 4 Main Drivers of Transition Risk, and Why the Risks Are Increasing Apr 12, 2022
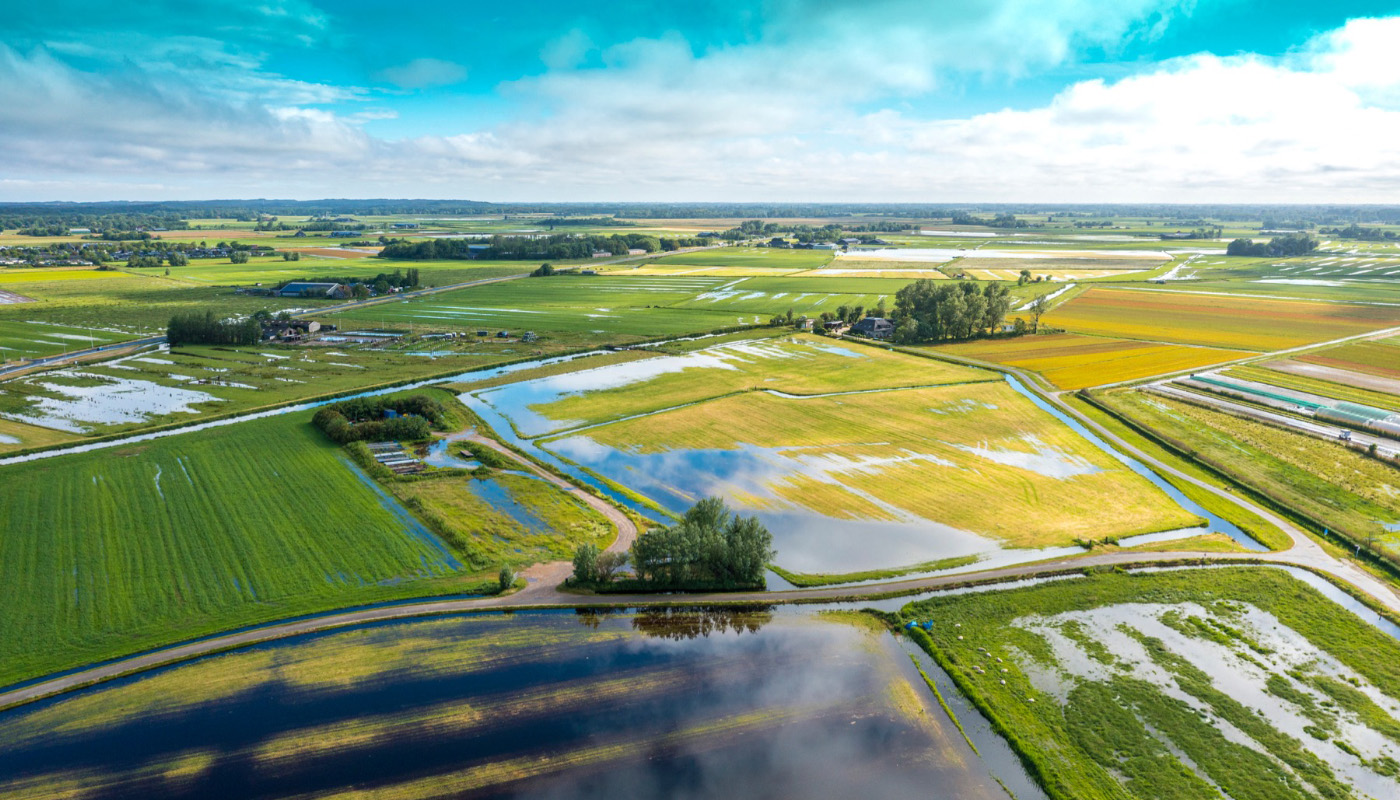
Understanding the Physical Risks Associated with Climate Change Apr 27, 2022
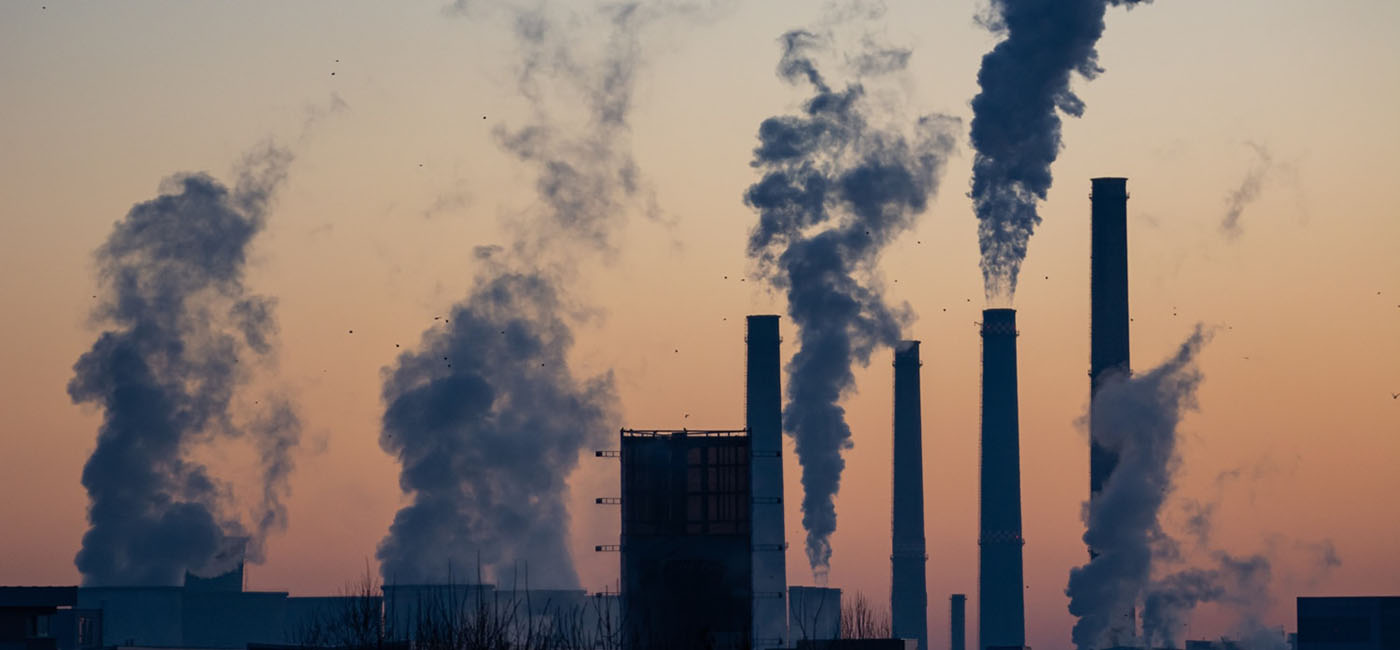
India: A Case Study in Climate Mitigation and Adaptation Sep 14, 2023
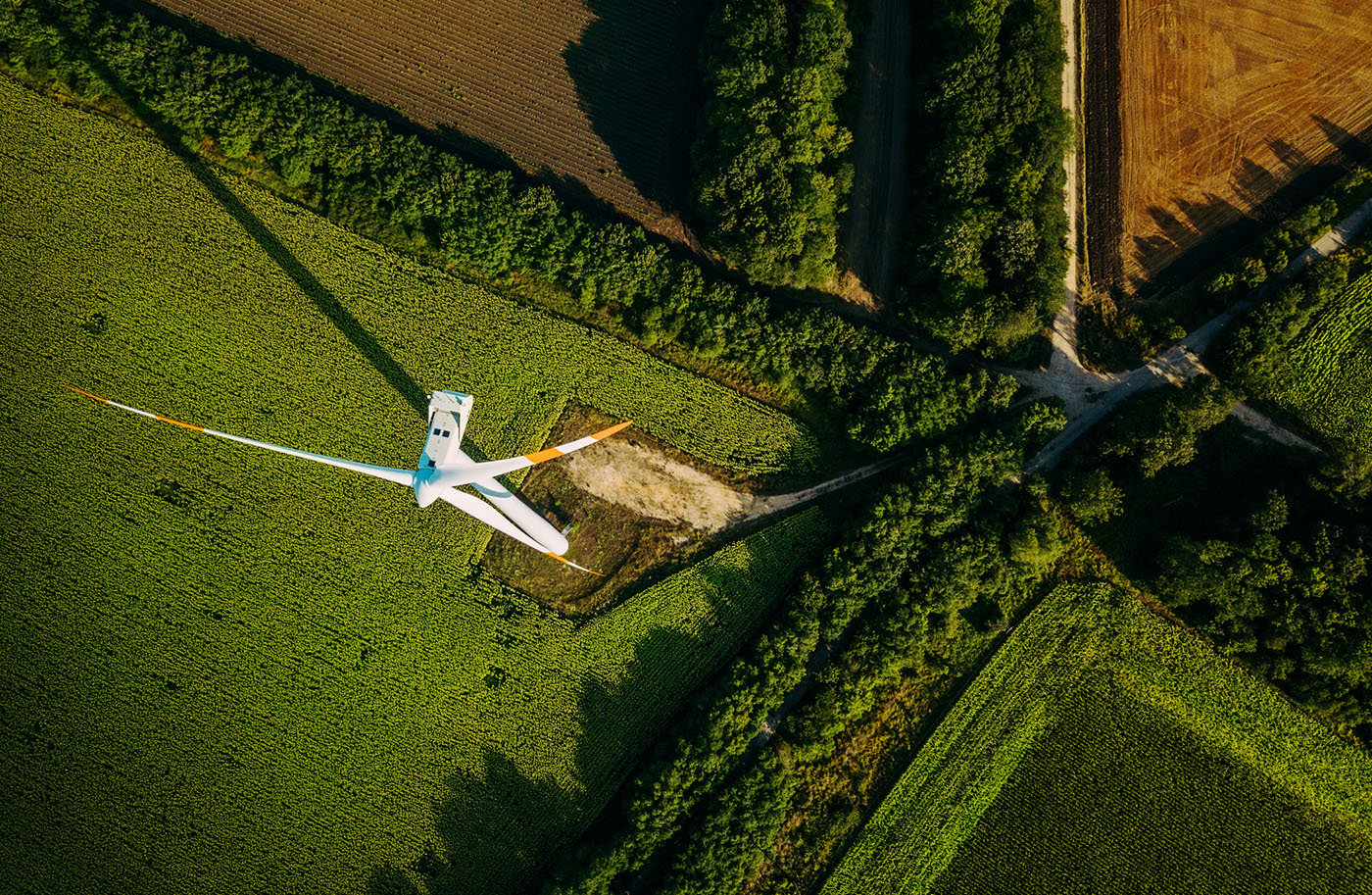
4 Key Areas to Know About Climate Change May 18, 2023
- Financial Risk Manager
- Sustainability and Climate Risk
We are a not-for-profit organization and the leading globally recognized membership association for risk managers.
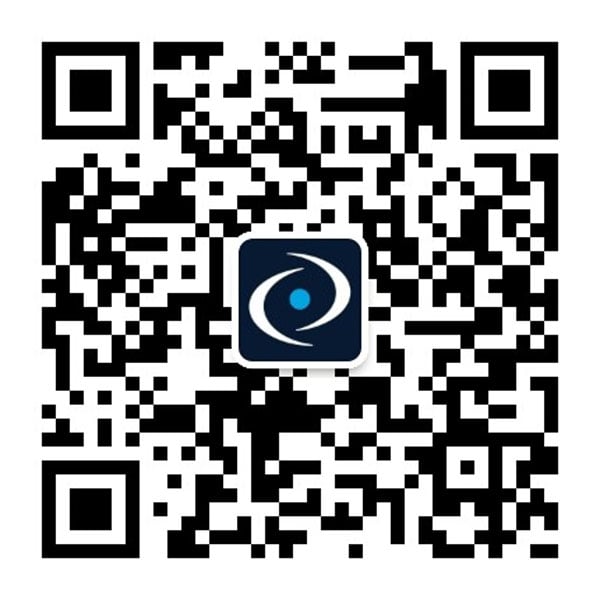
• Bylaws • Code of Conduct • Privacy Notice • Terms of Use © 2024 Global Association of Risk Professionals
Create an account
Create a free IEA account to download our reports or subcribe to a paid service.
Overview and key findings
Tracking cop28 progress.
- United States
- Latin America and the Caribbean
- European Union
- Middle East
- Japan and Korea
- Southeast Asia
Cite report
IEA (2024), World Energy Investment 2024 , IEA, Paris https://www.iea.org/reports/world-energy-investment-2024, Licence: CC BY 4.0
Share this report
- Share on Twitter Twitter
- Share on Facebook Facebook
- Share on LinkedIn LinkedIn
- Share on Email Email
- Share on Print Print
Report options
The world now invests almost twice as much in clean energy as it does in fossil fuels…, global investment in clean energy and fossil fuels, 2015-2024, …but there are major imbalances in investment, and emerging market and developing economies (emde) outside china account for only around 15% of global clean energy spending, annual investment in clean energy by selected country and region, 2019 and 2024, investment in solar pv now surpasses all other generation technologies combined, global annual investment in solar pv and other generation technologies, 2021-2024, the integration of renewables and upgrades to existing infrastructure have sparked a recovery in spending on grids and storage, investment in power grids and storage by region 2017-2024, rising investments in clean energy push overall energy investment above usd 3 trillion for the first time.
Global energy investment is set to exceed USD 3 trillion for the first time in 2024, with USD 2 trillion going to clean energy technologies and infrastructure. Investment in clean energy has accelerated since 2020, and spending on renewable power, grids and storage is now higher than total spending on oil, gas, and coal.
As the era of cheap borrowing comes to an end, certain kinds of investment are being held back by higher financing costs. However, the impact on project economics has been partially offset by easing supply chain pressures and falling prices. Solar panel costs have decreased by 30% over the last two years, and prices for minerals and metals crucial for energy transitions have also sharply dropped, especially the metals required for batteries.
The annual World Energy Investment report has consistently warned of energy investment flow imbalances, particularly insufficient clean energy investments in EMDE outside China. There are tentative signs of a pick-up in these investments: in our assessment, clean energy investments are set to approach USD 320 billion in 2024, up by more 50% since 2020. This is similar to the growth seen in advanced economies (+50%), although trailing China (+75%). The gains primarily come from higher investments in renewable power, now representing half of all power sector investments in these economies. Progress in India, Brazil, parts of Southeast Asia and Africa reflects new policy initiatives, well-managed public tenders, and improved grid infrastructure. Africa’s clean energy investments in 2024, at over USD 40 billion, are nearly double those in 2020.
Yet much more needs to be done. In most cases, this growth comes from a very low base and many of the least-developed economies are being left behind (several face acute problems servicing high levels of debt). In 2024, the share of global clean energy investment in EMDE outside China is expected to remain around 15% of the total. Both in terms of volume and share, this is far below the amounts that are required to ensure full access to modern energy and to meet rising energy demand in a sustainable way.
Power sector investment in solar photovoltaic (PV) technology is projected to exceed USD 500 billion in 2024, surpassing all other generation sources combined. Though growth may moderate slightly in 2024 due to falling PV module prices, solar remains central to the power sector’s transformation. In 2023, each dollar invested in wind and solar PV yielded 2.5 times more energy output than a dollar spent on the same technologies a decade prior.
In 2015, the ratio of clean power to unabated fossil fuel power investments was roughly 2:1. In 2024, this ratio is set to reach 10:1. The rise in solar and wind deployment has driven wholesale prices down in some countries, occasionally below zero, particularly during peak periods of wind and solar generation. This lowers the potential for spot market earnings for producers and highlights the need for complementary investments in flexibility and storage capacity.
Investments in nuclear power are expected to pick up in 2024, with its share (9%) in clean power investments rising after two consecutive years of decline. Total investment in nuclear is projected to reach USD 80 billion in 2024, nearly double the 2018 level, which was the lowest point in a decade.
Grids have become a bottleneck for energy transitions, but investment is rising. After stagnating around USD 300 billion per year since 2015, spending is expected to hit USD 400 billion in 2024, driven by new policies and funding in Europe, the United States, China, and parts of Latin America. Advanced economies and China account for 80% of global grid spending. Investment in Latin America has almost doubled since 2021, notably in Colombia, Chile, and Brazil, where spending doubled in 2023 alone. However, investment remains worryingly low elsewhere.
Investments in battery storage are ramping up and are set to exceed USD 50 billion in 2024. But spending is highly concentrated. In 2023, for every dollar invested in battery storage in advanced economies and China, only one cent was invested in other EMDE.
Investment in energy efficiency and electrification in buildings and industry has been quite resilient, despite the economic headwinds. But most of the dynamism in the end-use sectors is coming from transport, where investment is set to reach new highs in 2024 (+8% compared to 2023), driven by strong electric vehicle (EV) sales.
The rise in clean energy spending is underpinned by emissions reduction goals, technological gains, energy security imperatives (particularly in the European Union), and an additional strategic element: major economies are deploying new industrial strategies to spur clean energy manufacturing and establish stronger market positions. Such policies can bring local benefits, although gaining a cost-competitive foothold in sectors with ample global capacity like solar PV can be challenging. Policy makers need to balance the costs and benefits of these programmes so that they increase the resilience of clean energy supply chains while maintaining gains from trade.
In the United States, investment in clean energy increases to an estimated more than USD 300 billion in 2024, 1.6 times the 2020 level and well ahead of the amount invested in fossil fuels. The European Union spends USD 370 billion on clean energy today, while China is set to spend almost USD 680 billion in 2024, supported by its large domestic market and rapid growth in the so-called “new three” industries: solar cells, lithium battery production and EV manufacturing.
Overall upstream oil and gas investment in 2024 is set to return to 2017 levels, but companies in the Middle East and Asia now account for a much larger share of the total
Change in upstream oil and gas investment by company type, 2017-2024, newly approved lng projects, led by the united states and qatar, bring a new wave of investment that could boost global lng export capacity by 50%, investment and cumulative capacity in lng liquefaction, 2015-2028, investment in fuel supply remains largely dominated by fossil fuels, although interest in low-emissions fuels is growing fast from a low base.
Upstream oil and gas investment is expected to increase by 7% in 2024 to reach USD 570 billion, following a 9% rise in 2023. This is being led by Middle East and Asian NOCs, which have increased their investments in oil and gas by over 50% since 2017, and which account for almost the entire rise in spending for 2023-2024.
Lower cost inflation means that the headline rise in spending results in an even larger rise in activity, by approximately 25% compared with 2022. Existing fields account for around 40% total oil and gas upstream investment, while another 33% goes to new fields and exploration. The remainder goes to tight oil and shale gas.
Most of the huge influx of cashflows to the oil and gas industry in 2022-2023 was either returned to shareholders, used to buy back shares or to pay down debt; these uses exceeded capital expenditure again in 2023. A surge in profits has also spurred a wave of mergers and acquisitions (M&A), especially among US shale companies, which represented 75% of M&A activity in 2023. Clean energy spending by oil and gas companies grew to around USD 30 billion in 2023 (of which just USD 1.5 billion was by NOCs), but this represents less than 4% of global capital investment on clean energy.
A significant wave of new investment is expected in LNG in the coming years as new liquefaction plants are built, primarily in the United States and Qatar. The concentration of projects looking to start operation in the second half of this decade could increase competition and raise costs for the limited number of specialised contractors in this area. For the moment, the prospect of ample gas supplies has not triggered a major reaction further down the value chain. The amount of new gas-fired power capacity being approved and coming online remains stable at around 50-60 GW per year.
Investment in coal has been rising steadily in recent years, and more than 50 GW of unabated coal-fired power generation was approved in 2023, the most since 2015, and almost all of this was in China.
Investment in low-emissions fuels is only 1.4% of the amount spent on fossil fuels (compared to about 0.5% a decade ago). There are some fast-growing areas. Investments in hydrogen electrolysers have risen to around USD 3 billion per year, although they remain constrained by uncertainty about demand and a lack of reliable offtakers. Investments in sustainable aviation fuels have reached USD 1 billion, while USD 800 million is going to direct air capture projects (a 140% increase from 2023). Some 20 commercial-scale carbon capture utilisation and storage (CCUS) projects in seven countries reached final investment decision (FID) in 2023; according to company announcements, another 110 capture facilities, transport and storage projects could do the same in 2024.
Energy investment decisions are primarily driven and financed by the private sector, but governments have essential direct and indirect roles in shaping capital flows
Sources of investment in the energy sector, average 2018-2023, sources of finance in the energy sector, average 2018-2023, households are emerging as important actors for consumer-facing clean energy investments, highlighting the importance of affordability and access to capital, change in energy investment volume by region and fuel category, 2016 versus 2023, market sentiment around sustainable finance is down from the high point in 2021, with lower levels of sustainable debt issuances and inflows into sustainable funds, sustainable debt issuances, 2020-2023, sustainable fund launches, 2020-2023, energy transitions are reshaping how energy investment decisions are made, and by whom.
This year’s World Energy Investment report contains new analysis on sources of investments and sources of finance, making a clear distinction between those making investment decisions (governments, often via state-owned enterprises (SOEs), private firms and households) and the institutions providing the capital (the public sector, commercial lenders, and development finance institutions) to finance these investments.
Overall, most investments in the energy sector are made by corporates, with firms accounting for the largest share of investments in both the fossil fuel and clean energy sectors. However, there are significant country-by-country variations: half of all energy investments in EMDE are made by governments or SOEs, compared with just 15% in advanced economies. Investments by state-owned enterprises come mainly from national oil companies, notably in the Middle East and Asia where they have risen substantially in recent years, and among some state-owned utilities. The financial sustainability, investment strategies and the ability for SOEs to attract private capital therefore become a central issue for secure and affordable transitions.
The share of total energy investments made or decided by private households (if not necessarily financed by them directly) has doubled from 9% in 2015 to 18% today, thanks to the combined growth in rooftop solar installations, investments in buildings efficiency and electric vehicle purchases. For the moment, these investments are mainly made by wealthier households – and well-designed policies are essential to making clean energy technologies more accessible to all . A comparison shows that households have contributed to more than 40% of the increase in investment in clean energy spending since 2016 – by far the largest share. It was particularly pronounced in advanced economies, where, because of strong policy support, households accounted for nearly 60% of the growth in energy investments.
Three quarters of global energy investments today are funded from private and commercial sources, and around 25% from public finance, and just 1% from national and international development finance institutions (DFIs).
Other financing options for energy transition have faced challenges and are focused on advanced economies. In 2023, sustainable debt issuances exceeded USD 1 trillion for the third consecutive year, but were still 25% below their 2021 peak, as rising coupon rates dampened issuers’ borrowing appetite. Market sentiment for sustainable finance is wavering, with flows to ESG funds decreasing in 2023, due to potential higher returns elsewhere and credibility concerns. Transition finance is emerging to mobilise capital for high-emitting sectors, but greater harmonisation and credible standards are required for these instruments to reach scale.
A secure and affordable transitioning away from fossil fuels requires a major rebalancing of investments
Investment change in 2023-2024, and additional average annual change in investment in the net zero scenario, 2023-2030, a doubling of investments to triple renewables capacity and a tripling of spending to double efficiency: a steep hill needs climbing to keep 1.5°c within reach, investments in renewables, grids and battery storage in the net zero emissions by 2050 scenario, historical versus 2030, investments in end-use sectors in the net zero emissions by 2050 scenario, historical versus 2030, meeting cop28 goals requires a doubling of clean energy investment by 2030 worldwide, and a quadrupling in emde outside china, investments in renewables, grids, batteries and end use in the net zero emissions by 2050 scenario, 2024 and 2030, mobilising additional, affordable financing is the key to a safer and more sustainable future, breakdown of dfi financing by instrument, currency, technology and region, average 2019-2022, much greater efforts are needed to get on track to meet energy & climate goals, including those agreed at cop28.
Today’s investment trends are not aligned with the levels necessary for the world to have a chance of limiting global warming to 1.5°C above pre-industrial levels and to achieve the interim goals agreed at COP28. The current momentum behind renewable power is impressive, and if the current spending trend continues, it would cover approximately two-thirds of the total investment needed to triple renewable capacity by 2030. But an extra USD 500 billion per year is required in the IEA’s Net Zero Emissions by 2050 Scenario (NZE Scenario) to fill the gap completely (including spending for grids and battery storage). This equates to a doubling of current annual spending on renewable power generation, grids, and storage in 2030, in order to triple renewable capacity.
The goal of doubling the pace of energy efficiency improvement requires an even greater additional effort. While investment in the electrification of transport is relatively strong and brings important efficiency gains, investment in other efficiency measures – notably building retrofits – is well below where it needs to be: efficiency investments in buildings fell in 2023 and are expected to decline further in 2024. A tripling in the current annual rate of spending on efficiency and electrification – to about USD 1.9 trillion in 2030 – is needed to double the rate of energy efficiency improvements.
Anticipated oil and gas investment in 2024 is broadly in line with the level of investment required in 2030 in the Stated Policies Scenario, a scenario which sees oil and natural gas demand levelling off before 2030. However, global spare oil production capacity is already close to 6 million barrels per day (excluding Iran and Russia) and there is a shift expected in the coming years towards a buyers’ market for LNG. Against this backdrop, the risk of over-investment would be strong if the world moves swiftly to meet the net zero pledges and climate goals in the Announced Pledges Scenario (APS) and the NZE Scenario.
The NZE Scenario sees a major rebalancing of investments in fuel supply, away from fossil fuels and towards low-emissions fuels, such as bioenergy and low-emissions hydrogen, as well as CCUS. Achieving net zero emissions globally by 2050 would mean annual investment in oil, gas, and coal falls by more than half, from just over USD 1 trillion in 2024 to below USD 450 billion per year in 2030, while spending on low-emissions fuels increases tenfold, to about USD 200 billion in 2030 from just under USD 20 billion today.
The required increase in clean energy investments in the NZE Scenario is particularly steep in many emerging and developing economies. The cost of capital remains one of the largest barriers to investment in clean energy projects and infrastructure in many EMDE, with financing costs at least twice as high as in advanced economies as well as China. Macroeconomic and country-specific factors are the major contributors to the high cost of capital for clean energy projects, but so, too, are risks specific to the energy sector. Alongside actions by national policy makers, enhanced support from DFIs can play a major role in lowering financing costs and bringing in much larger volumes of private capital.
Targeted concessional support is particularly important for the least-developed countries that will otherwise struggle to access adequate capital. Our analysis shows cumulative financing for energy projects by DFIs was USD 470 billion between 2013 and 2021, with China-based DFIs accounting for slightly over half of the total. There was a significant reduction in financing for fossil fuel projects over this period, largely because of reduced Chinese support. However, this was not accompanied by a surge in support for clean energy projects. DFI support was provided almost exclusively (more than 90%) as debt (not all concessional) with only about 3% reported as equity financing and about 6% as grants. This debt was provided in hard currency or in the currency of donors, with almost no local-currency financing being reported.
The lack of local-currency lending pushes up borrowing costs and in many cases is the primary reason behind the much higher cost of capital in EMDE compared to advanced economies. High hedging costs often make this financing unaffordable to many of the least-developed countries and raises questions of debt sustainability. More attention is needed from DFIs to focus interventions on project de-risking that can mobilise much higher multiples of private capital.
Subscription successful
Thank you for subscribing. You can unsubscribe at any time by clicking the link at the bottom of any IEA newsletter.

Search the United Nations
- What Is Climate Change
- Myth Busters
- Renewable Energy
- Finance & Justice
- Initiatives
- Sustainable Development Goals
- Paris Agreement
- Climate Ambition Summit 2023
- Climate Conferences
- Press Material
- Communications Tips
Causes and Effects of Climate Change
Fossil fuels – coal, oil and gas – are by far the largest contributor to global climate change, accounting for over 75 per cent of global greenhouse gas emissions and nearly 90 per cent of all carbon dioxide emissions.
As greenhouse gas emissions blanket the Earth, they trap the sun’s heat. This leads to global warming and climate change. The world is now warming faster than at any point in recorded history. Warmer temperatures over time are changing weather patterns and disrupting the usual balance of nature. This poses many risks to human beings and all other forms of life on Earth.
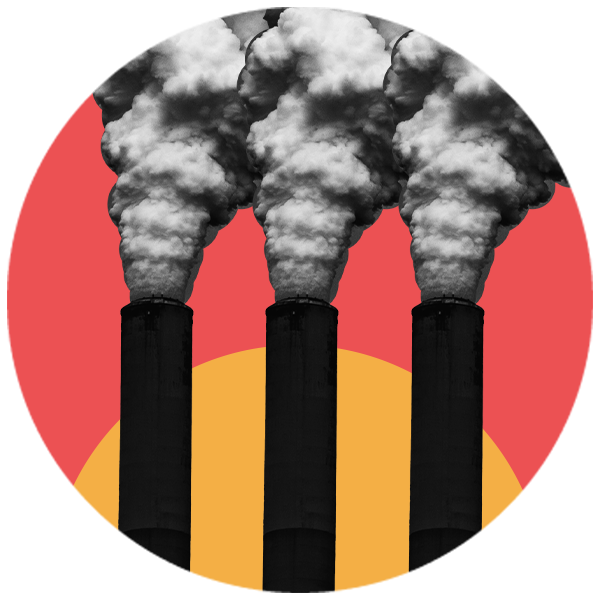
Causes of Climate Change
Generating power
Generating electricity and heat by burning fossil fuels causes a large chunk of global emissions. Most electricity is still generated by burning coal, oil, or gas, which produces carbon dioxide and nitrous oxide – powerful greenhouse gases that blanket the Earth and trap the sun’s heat. Globally, a bit more than a quarter of electricity comes from wind, solar and other renewable sources which, as opposed to fossil fuels, emit little to no greenhouse gases or pollutants into the air.
Manufacturing goods
Manufacturing and industry produce emissions, mostly from burning fossil fuels to produce energy for making things like cement, iron, steel, electronics, plastics, clothes, and other goods. Mining and other industrial processes also release gases, as does the construction industry. Machines used in the manufacturing process often run on coal, oil, or gas; and some materials, like plastics, are made from chemicals sourced from fossil fuels. The manufacturing industry is one of the largest contributors to greenhouse gas emissions worldwide.
Cutting down forests
Cutting down forests to create farms or pastures, or for other reasons, causes emissions, since trees, when they are cut, release the carbon they have been storing. Each year approximately 12 million hectares of forest are destroyed. Since forests absorb carbon dioxide, destroying them also limits nature’s ability to keep emissions out of the atmosphere. Deforestation, together with agriculture and other land use changes, is responsible for roughly a quarter of global greenhouse gas emissions.
Using transportation
Most cars, trucks, ships, and planes run on fossil fuels. That makes transportation a major contributor of greenhouse gases, especially carbon-dioxide emissions. Road vehicles account for the largest part, due to the combustion of petroleum-based products, like gasoline, in internal combustion engines. But emissions from ships and planes continue to grow. Transport accounts for nearly one quarter of global energy-related carbon-dioxide emissions. And trends point to a significant increase in energy use for transport over the coming years.
Producing food
Producing food causes emissions of carbon dioxide, methane, and other greenhouse gases in various ways, including through deforestation and clearing of land for agriculture and grazing, digestion by cows and sheep, the production and use of fertilizers and manure for growing crops, and the use of energy to run farm equipment or fishing boats, usually with fossil fuels. All this makes food production a major contributor to climate change. And greenhouse gas emissions also come from packaging and distributing food.
Powering buildings
Globally, residential and commercial buildings consume over half of all electricity. As they continue to draw on coal, oil, and natural gas for heating and cooling, they emit significant quantities of greenhouse gas emissions. Growing energy demand for heating and cooling, with rising air-conditioner ownership, as well as increased electricity consumption for lighting, appliances, and connected devices, has contributed to a rise in energy-related carbon-dioxide emissions from buildings in recent years.
Consuming too much
Your home and use of power, how you move around, what you eat and how much you throw away all contribute to greenhouse gas emissions. So does the consumption of goods such as clothing, electronics, and plastics. A large chunk of global greenhouse gas emissions are linked to private households. Our lifestyles have a profound impact on our planet. The wealthiest bear the greatest responsibility: the richest 1 per cent of the global population combined account for more greenhouse gas emissions than the poorest 50 per cent.
Based on various UN sources
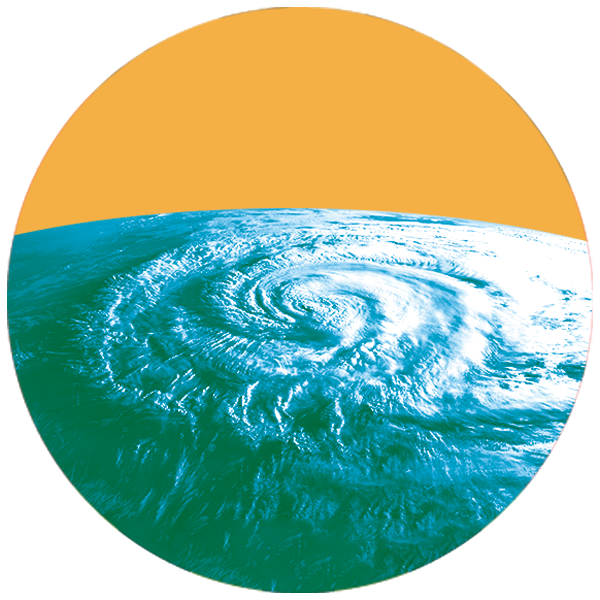
Effects of Climate Change
Hotter temperatures
As greenhouse gas concentrations rise, so does the global surface temperature. The last decade, 2011-2020, is the warmest on record. Since the 1980s, each decade has been warmer than the previous one. Nearly all land areas are seeing more hot days and heat waves. Higher temperatures increase heat-related illnesses and make working outdoors more difficult. Wildfires start more easily and spread more rapidly when conditions are hotter. Temperatures in the Arctic have warmed at least twice as fast as the global average.
More severe storms
Destructive storms have become more intense and more frequent in many regions. As temperatures rise, more moisture evaporates, which exacerbates extreme rainfall and flooding, causing more destructive storms. The frequency and extent of tropical storms is also affected by the warming ocean. Cyclones, hurricanes, and typhoons feed on warm waters at the ocean surface. Such storms often destroy homes and communities, causing deaths and huge economic losses.
Increased drought
Climate change is changing water availability, making it scarcer in more regions. Global warming exacerbates water shortages in already water-stressed regions and is leading to an increased risk of agricultural droughts affecting crops, and ecological droughts increasing the vulnerability of ecosystems. Droughts can also stir destructive sand and dust storms that can move billions of tons of sand across continents. Deserts are expanding, reducing land for growing food. Many people now face the threat of not having enough water on a regular basis.
A warming, rising ocean
The ocean soaks up most of the heat from global warming. The rate at which the ocean is warming strongly increased over the past two decades, across all depths of the ocean. As the ocean warms, its volume increases since water expands as it gets warmer. Melting ice sheets also cause sea levels to rise, threatening coastal and island communities. In addition, the ocean absorbs carbon dioxide, keeping it from the atmosphere. But more carbon dioxide makes the ocean more acidic, which endangers marine life and coral reefs.
Loss of species
Climate change poses risks to the survival of species on land and in the ocean. These risks increase as temperatures climb. Exacerbated by climate change, the world is losing species at a rate 1,000 times greater than at any other time in recorded human history. One million species are at risk of becoming extinct within the next few decades. Forest fires, extreme weather, and invasive pests and diseases are among many threats related to climate change. Some species will be able to relocate and survive, but others will not.
Not enough food
Changes in the climate and increases in extreme weather events are among the reasons behind a global rise in hunger and poor nutrition. Fisheries, crops, and livestock may be destroyed or become less productive. With the ocean becoming more acidic, marine resources that feed billions of people are at risk. Changes in snow and ice cover in many Arctic regions have disrupted food supplies from herding, hunting, and fishing. Heat stress can diminish water and grasslands for grazing, causing declining crop yields and affecting livestock.
More health risks
Climate change is the single biggest health threat facing humanity. Climate impacts are already harming health, through air pollution, disease, extreme weather events, forced displacement, pressures on mental health, and increased hunger and poor nutrition in places where people cannot grow or find sufficient food. Every year, environmental factors take the lives of around 13 million people. Changing weather patterns are expanding diseases, and extreme weather events increase deaths and make it difficult for health care systems to keep up.
Poverty and displacement
Climate change increases the factors that put and keep people in poverty. Floods may sweep away urban slums, destroying homes and livelihoods. Heat can make it difficult to work in outdoor jobs. Water scarcity may affect crops. Over the past decade (2010–2019), weather-related events displaced an estimated 23.1 million people on average each year, leaving many more vulnerable to poverty. Most refugees come from countries that are most vulnerable and least ready to adapt to the impacts of climate change.
Learn more about...
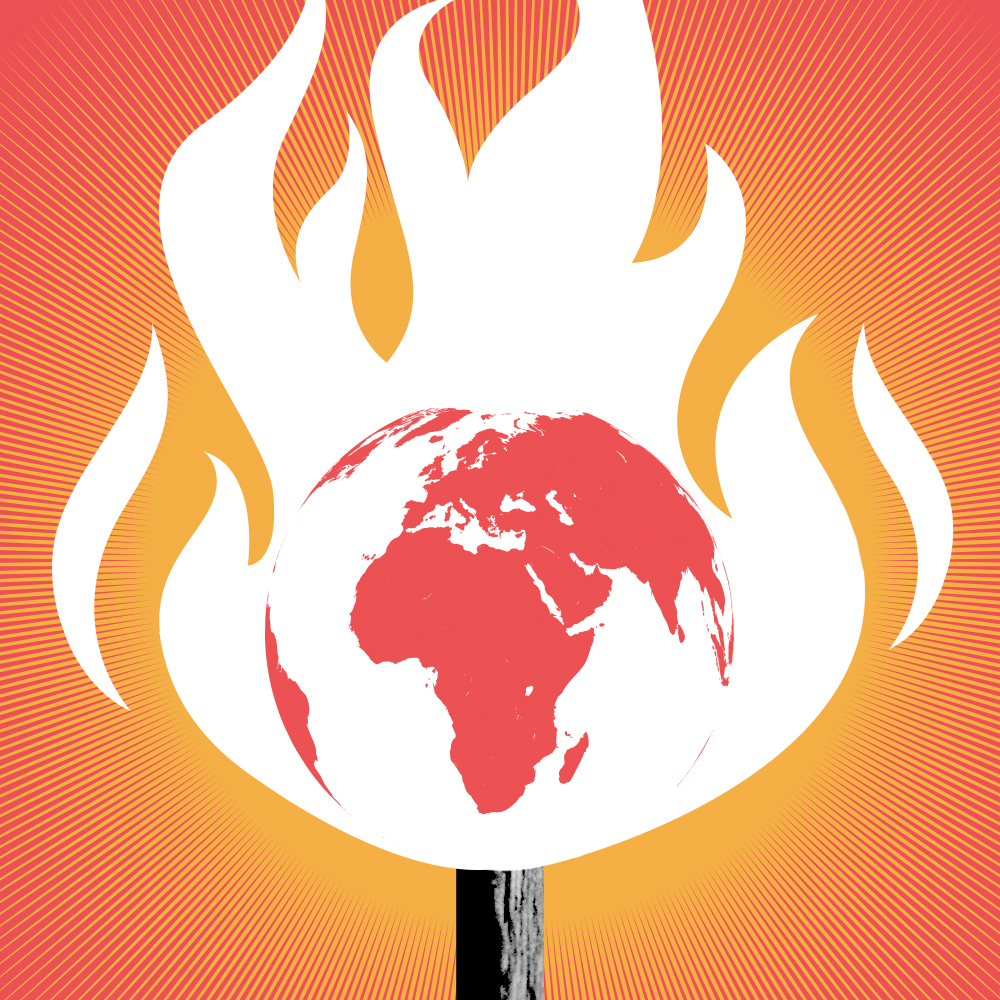
- What is climate change?
Our climate 101 offers a quick take on the how and why of climate change.
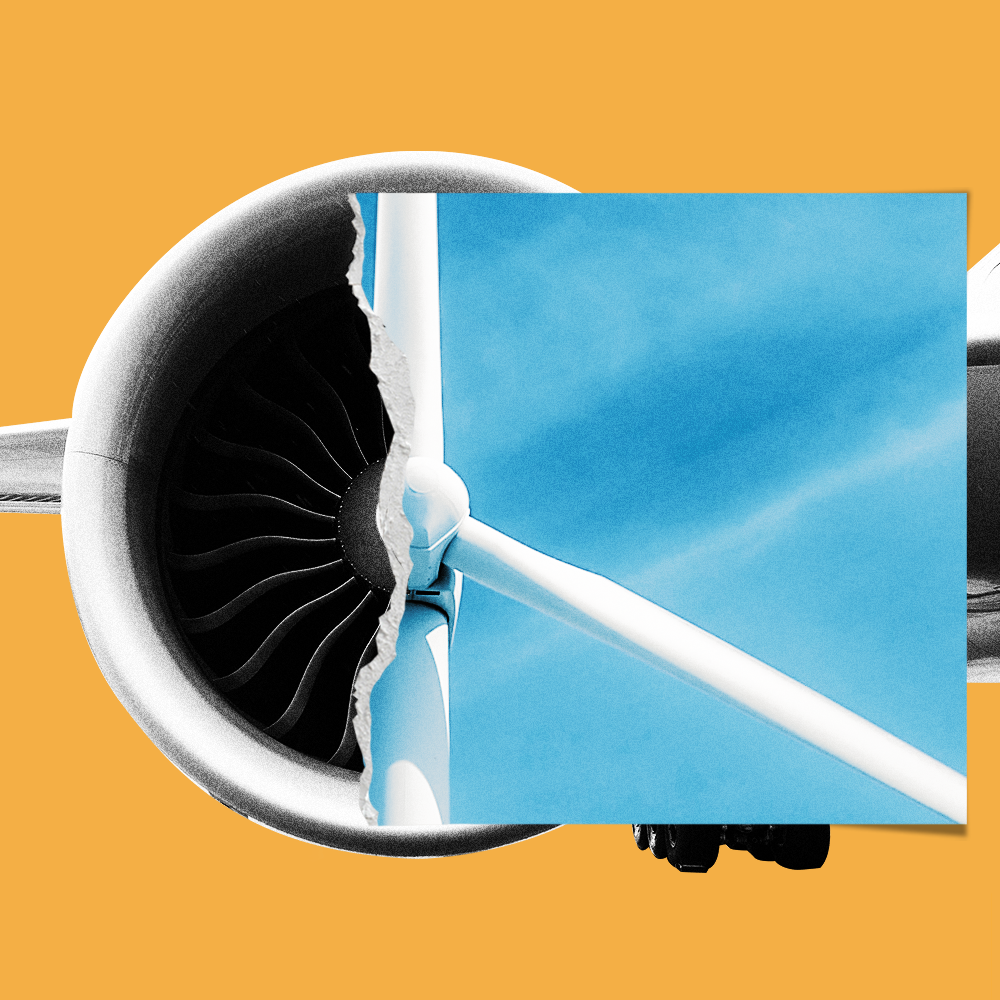
What is “net zero”, why is it important, and is the world on track to reach it?
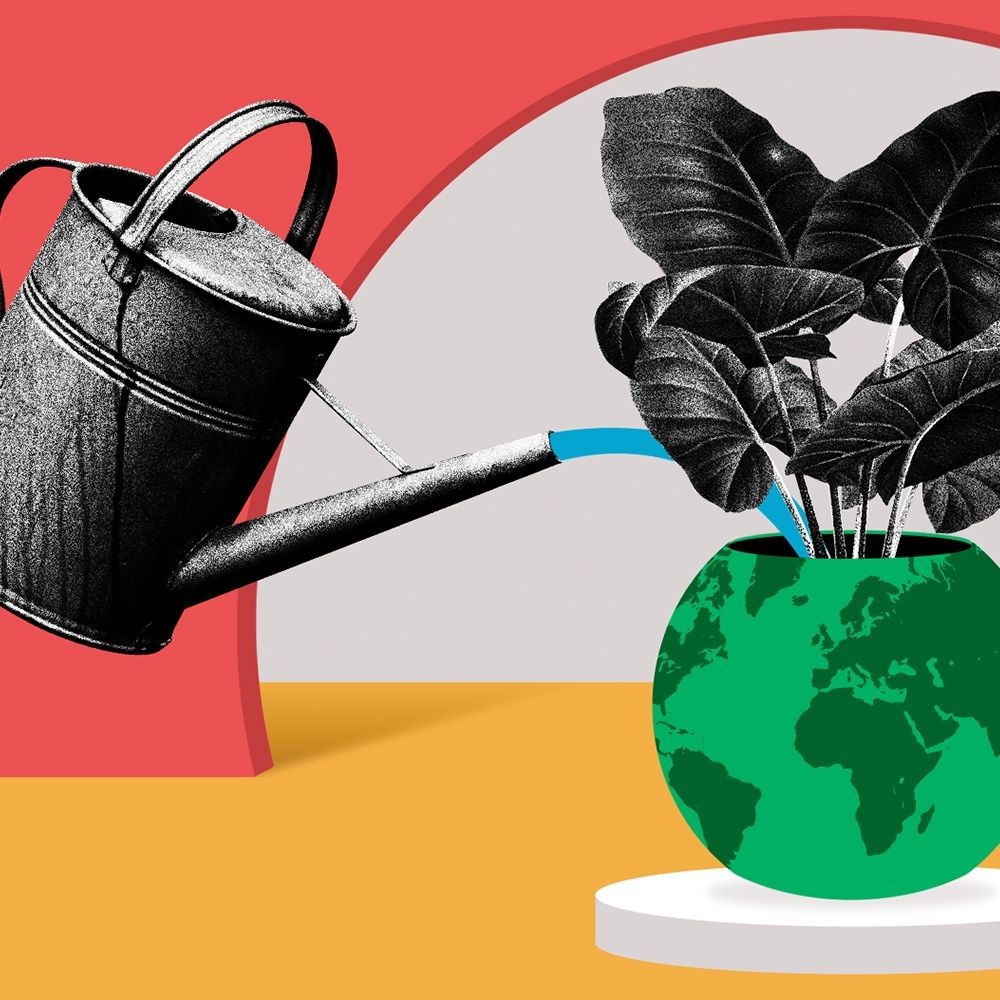
Initiatives for action
Read about global initiatives aimed at speeding up the pace of climate action.
Facts and figures
- Causes and effects
- Myth busters
Cutting emissions
- Explaining net zero
- High-level expert group on net zero
- Checklists for credibility of net-zero pledges
- Greenwashing
- What you can do
Clean energy
- Renewable energy – key to a safer future
- What is renewable energy
- Five ways to speed up the energy transition
- Why invest in renewable energy
- Clean energy stories
- A just transition
Adapting to climate change
- Climate adaptation
- Early warnings for all
- Youth voices
Financing climate action
- Finance and justice
- Loss and damage
- $100 billion commitment
- Why finance climate action
- Biodiversity
- Human Security
International cooperation
- What are Nationally Determined Contributions
- Acceleration Agenda
- Climate Ambition Summit
- Climate conferences (COPs)
- Youth Advisory Group
- Action initiatives
- Secretary-General’s speeches
- Press material
- Fact sheets
- Communications tips
Unilever Global Change location
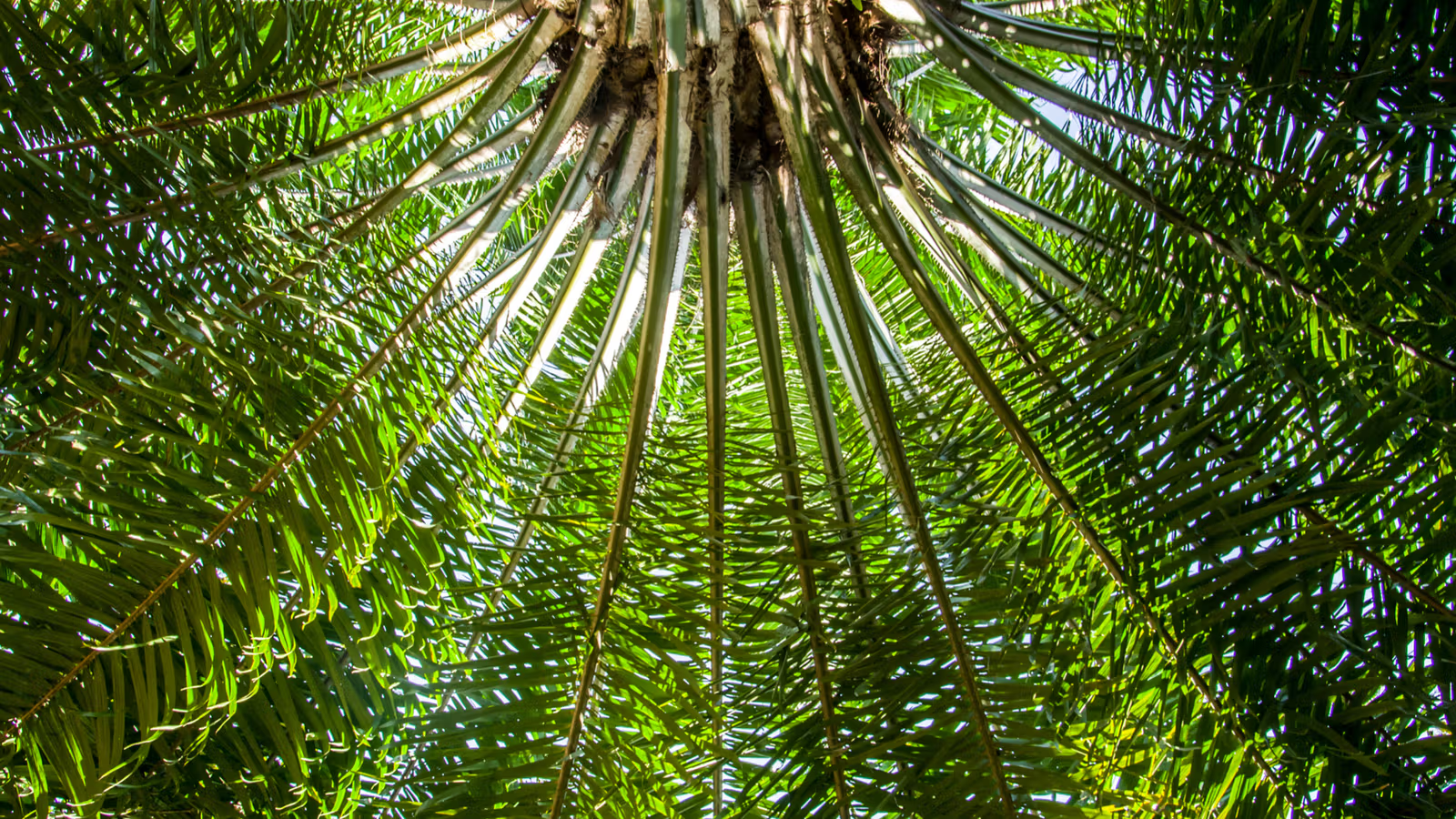
Leading in the next era of corporate sustainability
Fewer things, done better, with greater impact.
Our Growth Action Plan addresses these challenges head-on. In the coming years, our focus will be on four sustainability priorities .
A montage of Unilever products being used together with agricultural footage.
Detailed description
A jar of Hellmann’s mayonnaise on a kitchen counter, a sandwich in the background with someone holding a knife. The sandwich is then pressed down ready to cut.
Tomatoes growing on a vine. Someone in the foreground with a handful of soil.
A woman rubs Vaseline on her lower legs.
Rain falling into a pool of water in a rice field. A rice field with water droplets rising up.
A man tosses a Persil capsule into a washing machine. A Persil 3-in-1 Bio packshot with the words ‘Dirt is good’.
Our ambition is to deliver net zero emissions across our value chain.
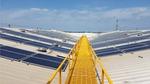
Our ambition is to deliver resilient and regenerative natural and agricultural ecosystems.
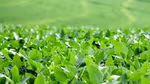
Our ambition is an end to plastic pollution through reduction, circulation and collaboration.
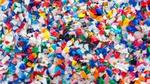
Livelihoods
Our ambition is to ensure a decent livelihood for people in our global value chain, including by earning a living wage.
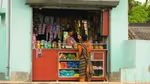
The next era of corporate sustainability
The first era was about ringing the alarm. The second was about setting long-term ambitions. The third is about delivering impact faster , by making sustainability progress integral to business performance.
We intend to lead in this new era by being:
More focused in allocating our resources
using capital allocation to make progress across our sustainability priorities.
More urgent in our actions
with roadmaps, clear accountability and reward.
More systemic in our advocacy
through deeper collaboration and more assertive policy advocacy.
"Our updated commitments are very stretching, but they are also intentionally and, unashamedly, realistic. We are determined that Unilever will deliver against them." Hein Schumacher, CEO Read our CEO Hein Schumacher’s views
Our sustainability news
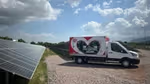
How sunshine drives sales and powers our ice cream business
10 June 2024
The sun doesn’t just drive our ice cream sales; it also helps power our business in a sustainable way. Find out how solar solutions integrated into our warehouses, fleets and sales kiosks are making energy savings, reducing emissions and contributing to our climate goals.
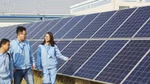
Why we’ve updated our Climate Transition Action Plan
16 May 2024
Our updated Climate Transition Action Plan (CTAP) sets out Unilever’s ambitious new climate targets. Discover how we’re focusing our efforts so we can deepen our impact by 2030, and why we believe taking urgent climate action now is good for our business in the long term.
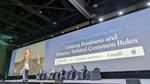
UN plastics treaty: reasons to be optimistic
With the fourth round of negotiations for a UN treaty to end plastic pollution now concluded, Unilever CEO Hein Schumacher reflects on the progress made and the road ahead.
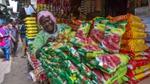
How we’re improving livelihoods across our value chain
Head of Social Sustainability, Anouk Heilen, explains how we’re ensuring a living wage for everyone we work with and why better living standards can build a stronger business.
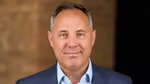
How we’re aiming for greater impact with updated plastic goals
30 April 2024
We recently announced an evolved sustainability agenda to make more tangible progress on the big complex challenges we face. Pablo Costa, Global Head of Packaging, explains what that means for our approach to plastic, and how our updated goals are aimed at making greater impact.
Looking for more on sustainability?
Find out about our approach to sustainability and our ongoing commitment to responsible business.
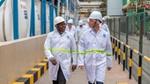
Our commitment to responsible business
Find out more about our progress and reporting on issues such as positive nutrition, business integrity, and safety.
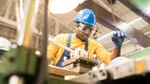
Human rights
Human rights continue to underpin our sustainability agenda. Find out more about our progress.
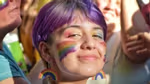
Equity, diversity and inclusion
Find out more about how we are working towards a society where everyone is treated equally.
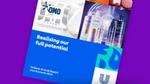
Unilever Annual Report
Read more about our Growth Action Plan and how we are stepping up our execution to deliver improved performance.
Legal disclaimer
Disclaimer covering our sustainability pages.
Read the disclaimer covering our sustainability pages (PDF 92.63 KB) .

IMAGES
VIDEO
COMMENTS
Farmers' perception of climate change, impact and adaptation strategies: a case study of four villages in the semi-arid regions of India Nat Hazards , 75 ( 3 ) ( 2015 ) , pp. 2829 - 2845 , 10.1007/s11069-014-1466-z
There are a number of studies which assessed the impact of climate change on Indian Agriculture and vulnerability of the small and medium rainfed farmers in different aspects. But the perceptions on the climate change and the factors which drive the farmers to adopt or to follow coping mechanisms have not been studied in detail.
Climate Change Impact on Agriculture Globally and in India: A Case Study. March 2021. DOI: 10.22214/ijraset.2021.33419. Authors: Prof. Pratik Priyadarshi. To read the full-text of this research ...
The study examines the impact of climatic changes on the production of the major crops grown in India. The study estimates the panel data from 1970 to 2020 by using the Panel Autoregressive Distrib...
Climate change poses serious risks to Indian agriculture as half of the agricultural land of the country is rainfed. Climate change affects crop yield, soil processes, water availability, and pest dynamics. Several adaptation strategies such as heat- and water stress-tolerant crop varieties, stress-tolerant new crops, improved agronomic management practices, improved water use efficiency ...
Climate change constitutes one of the most critical challenges of the contemporary period and can affect various sectors of economies across the globe, the agricultural sector is not an exception. This study aimed to assess the impact of climate change on India's agricultural sector from 1990 to 2020. The autoregressive distributed lag (ARDL) approach was utilized to determine the short-run ...
Chander, S. (2012) Impact of climate change on insects. In: Pathak, H. and P.K. Aggarwal, (eds) Climate Change Impact ... Dalgaard, T.et al. (2018) Impacts of climate change adaptation options on soil functions: A review of European case-studies ... N.G. (2021) Paving the way for digital green economy in Indian agriculture. India Matters. ...
Climate variations have significant impact on agriculture production and farm livelihoods especially in India, with diverse agro-climatic settings. Several studies in the past have attempted to analyse the variability/trend in meteorological variables and the consequent impact of climate change on crop yields in the country.
In the context of climate change, the Indian agricultural sector treads in a certain duality between promoting food security in response to the increasing population, but at the same time in ensuring environmental sustainability, and sustained economic growth, especially in developing countries like India. The concept of Climate Smart Agriculture (CSA) emerged from the recognition of this ...
directly affected by the climate change. Agriculture and climate change are inextricably linked and has a direct biophysical effect on the agricultural production in general and specically deteriorating the soil health. Unfortunately, current farming practices and climate changes result in soil degradation. Soil is linked to the atmospheric system
The lived experiences of people across the most climate-vulnerable regions in India, as documented in the Faces of Climate Resilience project, reflect a determination to respond with action. From Kerala to Rajasthan, individuals and communities are making efforts to ward off the worst effects of global warming and adapt through solutions.
Climate change is likely to have significant impact on agriculture production and farm livelihoods in India, with diverse agro-climatic settings. Several studies in the past have analysed the variability/trend in meteorological variables and the consequent impact of climate change on crop yields in the country. A
Daily Updates of the Latest Projects & Documents. This report provides information on a series of associated analyses done on how climate change impacts on the agricultural system in India. The analysis features the .
Studies have shown that these climate trends have had a negative impact on Indian agriculture, reducing relative yields by several percent ( 3, 4 ). However, although temperature and precipitation changes have and will continue to ( 5) impact future yields, these two variables alone do not tell the entire story of India's changing crop yields ...
In India, 78% of farmers are small and marginal, cultivating only 33% of the arable land but producing 50% of the food grain; their vulnerability to climate change poses a significant threat to the country's food security. To enhance agricultural resilience, it is crucial to understand how these farmers perceive and integrate climate-smart technologies into their farming practices. A random ...
India has taken several proactive steps for addressing the issues of climate change in agriculture. Recently, it has also committed for reducing GHG emission intensity by 45% by 2030 and achieving net zero emission by 2070. The paper discusses the major impacts of climate change, potential adaptation, and mitigation options and the initiatives ...
Climate change constitutes one of the most critical challenges of the contemporary period and can affect various sectors of economies across the globe, the agricultural sector is not an exception. This study aimed to assess the impact of climate change on India's agricultural sector from 1990 to 2020. The autoregressive distributed lag (ARDL) approach was utilized to determine the short-run ...
Ashalatha, KV, M Gopinath and ARS Bhat [2012] Impact of climate change on rainfed agriculture in India: a case study of Dharwad. International Journal of Environmental Science and Development, 3(4), 368-371. Google Scholar; ASP (2013). Bay of Bengal — a hotspot for climate insecurity. American Security Project. Google Scholar
Government of India is aware about the impact of climate change on agriculture and farmers' lives. Extensive field and simulation studies were carried out in agriculture by the network centres located in different parts of the country. The climate change impact assessment was carried out using the crop simulation models by incorporating the ...
From 1850-1900 to 2006-2015 mean land surface air temperature has increased by 1.53°C (very likely range from 1.38°C to 1.68°C) while GMST increased by 0.87°C (likely range from 0.75°C to 0.99°C). Fig 1: Change in temperature relative to 1850-2018. Fig 2: Impact of climate change and global warming. Climate change impact on soil.
The impact of climate change is studied in various ways in different locations in the country and it is concluded that there is high impact on agriculture compared to any other sector in the country. The study results revealed that the climatic variation such as occurrence of drought has high level of impact on the yield of Rainfed crops.
Indian agricultural workers plant paddy saplings at a field after monsoon rains on the outskirts of Lucknow, India, June 30, 2021. Human-caused climate change is making rainfall more unpredictable and erratic, which makes it difficult for farmers to plant, grow and harvest crops on their rain-fed fields. (AP Photo/Rajesh Kumar Singh)
Office of Agricultural Affairs, New Delhi | (011-91-11) 2419-8769. Link to report: Climate Change - Agriculture and Policy in India. India's agricultural sector is highly vulnerable to the effects of climate change and extreme weather events. With a rapidly growing population and limited natural resource base, India's grain and livestock ...
India's Effect on Climate Change. Not only will the changing climate have a significant impact on India, but India is also expected to have a significant impact on the climate. Although historically it has not had high emissions, India rose to the number three spot in the national emissions rankings 15 years ago, behind China and the U.S.
Much greater efforts are needed to get on track to meet energy & climate goals, including those agreed at COP28 Today's investment trends are not aligned with the levels necessary for the world to have a chance of limiting global warming to 1.5°C above pre-industrial levels and to achieve the interim goals agreed at COP28.
Climate Change and Indian Agriculture: Impacts, Adaptation and Mitigation Strategies ... India Oby 3 C or more by 2050. The study also ... central parts while it would rise by 2OC in southern parts by 2050. In the case of rainfall, a marginal increase of 7 to 10 percent in annual rainfall is projected over the subcontinent by the year 2080. ...
Climate impacts are already harming health, through air pollution, disease, extreme weather events, forced displacement, pressures on mental health, and increased hunger and poor nutrition in ...
A montage of Unilever products being used together with agricultural footage. Detailed description. A jar of Hellmann's mayonnaise on a kitchen counter, a sandwich in the background with someone holding a knife. The sandwich is then pressed down ready to cut. Tomatoes growing on a vine. Someone in the foreground with a handful of soil.
News at 2pm || 11th June, 2024 #gbcnews #news