- Skip to Nav
- Skip to Main
- Skip to Footer


Four computational thinking strategies for building problem-solving skills across the curriculum
Please try again
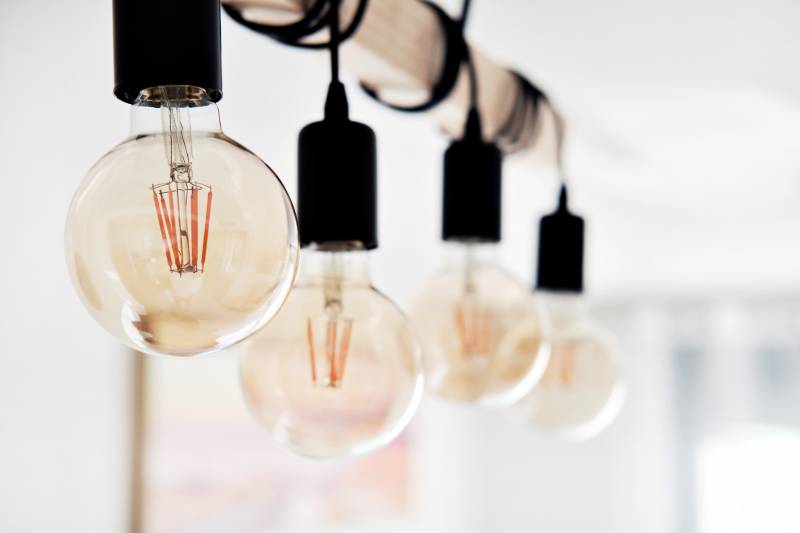
Two decades into the 21st century, educators are still tackling the question of how to help young people prepare for a rapidly evolving work landscape . Industry leaders have long called for more emphasis on skills such as critical thinking , communication and problem-solving , though the definitions and methods for teaching all of these can vary widely. At the International Society for Technology in Education conference in July, a number of education leaders and teachers discussed a framework that can help build students’ problem-solving skills in any subject: computational thinking.
Much of the research and discussion on computational thinking in the last twenty years has focused on computer science contexts . Harvard’s Karen Brennan , for example, has led studies and developed resources on computational thinking with Scratch . But several advocates argued that these skills are not just applicable to coding and should be integrated across the curriculum. They outlined four strategies that make up the computational thinking process:
Decomposition - breaking a complex problem into smaller parts or questions
Pattern recognition - identifying trends, differences or similarities in data
Abstraction - removing unnecessary elements or data to focus on what’s useful in solving a problem
Algorithmic design - making steps and rules to solve problems
Most problems will require students to employ multiple strategies. Julie Evans , CEO of the education nonprofit Project Tomorrow, illustrated that point by asking attendees at one session to draw a cat in less than 30 seconds. No drawing looked exactly the same, but the participating educators had to quickly break their mental image of a cat into important parts, such as a tail and whiskers (decomposition). They discarded unnecessary data; for instance, a cat can be conveyed by drawing its head and body or just its face (abstraction). And they envisioned and executed steps to get from a blank page to a completed drawing (algorithmic design).
Bryan Cox, who works in the Georgia Department of Education to broaden computer science education, offered practical and pedagogical reasons for integration. Not all schools offer computer science and even at schools that do, not all students take those classes . For elementary school teachers, stand-alone computer science lessons can feel like one more thing to add to an already packed curriculum. “Integration is less disruptive,” Cox said. He also said integration mirrors how computational thinking occurs in the real world in fields like medicine, automotives, law and sports.
Over the past two years, Project Tomorrow trained 120 teachers in New York City elementary schools to integrate computational thinking into their classrooms. In one example from a second and third grade writing unit, students wrote a realistic fiction story and created a movie to bring the story to life. That may sound like a pretty typical language arts project, but the difference was in the approach, according to Project Tomorrow instructional coach David Gomez. Rather than being told how to write a realistic fiction story, students developed an algorithm for the process, with steps such as making up a pretend character, giving the character a name, imagining the setting and so on. In this example and others, Gomez said that algorithms help students acknowledge the steps they are following during a task and increase their awareness of their work processes.
Gomez works with teachers to help students recognize when they’re using other computational thinking strategies, too. One second grade teacher, for example, used a poster with sticky notes for students to reflect on which strategies they’d used in different subjects throughout the day.
Evans said she loves hearing kids identify the strategies in discussions with each other. She’s heard questions like “Did you try abstraction?” and “Why didn’t you do pattern recognition ?” from students chatting with classmates. “Those little tykes in second grade are already developing their problem-solving muscles, and they’ve got the vocabulary to have that be a sustainable skill for the future,” she said.
Crafting computational problems
Not every question or problem is a computational one. Carolyn Sykora, senior director of the ISTE Standards programs, shared three characteristics that teachers can use to identify a computational problem:
- It’s open-ended with multiple potential solutions. “How can we design a car to get from point A to point B?” is an example that meets this criteria, whereas “How does a self-driving car work?” is a knowledge-based question.
- It requires using or collecting data. Data doesn’t just mean numbers. It could, for example, be the lines in a poem or the notes in a musical composition.
- It includes an opportunity to create a procedure or algorithm. In some cases, such as an engineering challenge, it’s easy to identify where this opportunity will arise. But often that’s not so clear. “Sometimes you don’t understand where the algorithm design comes into play until you do your problem decomposition,” Sykora said.
Using these characteristics can help teachers rethink curriculum, rather than trying to add something new. “We have our tried and true lessons and the things that we want our kids to learn,” Sykora said. The next step is to look at those lessons and ask, “How can we take something that’s knowledge-based and turn it into a computational problem?”

Curricular Components
Problem solving skills can easily be taught within the learning environment. if you are looking for a non-competitive problem solving resource, consider these options:.

Action-Based Problem Solving (AbPS)
AbPS is designed for integration into the classroom curriculum and can be used with the primary level (K-3) and up through grade 9. AbPS teaches a simplified version of the problem solving process, providing guidance in the writing of ideas. The materials may be used with a few students or with an entire class; either the teacher or the students may record the ideas that are generated; the work may be completed with complete teacher guidance or independently in small groups.
Some Affiliates offer AbPS as a non-competitive component. In these Affiliates, registration provides two additional problem solving scenes for students to address, and the registration entitles the teacher to submit the students’ work for evaluation twice during the year.

The Problem Solving Experience Curriculum
The Problem Solving Experience: Classroom Curriculum Designed to Promote Problem Solving in the 21st Century is a complete curriculum targeted at grades 5-8, and portions can be adjusted for use with younger or older students.The curriculum can be implemented as a full semester course, or spread across 1-4 school years. Complete lesson plans and resource materials are provided.
Problem Solving Across the Curriculum (PSAC)
PSAC provides hundreds of Future Scenes that have been used in the Global Issues Problem Solving component. Teachers may select Future Scenes to fit their curriculum and may modify the scenes to meet their students’ educational needs. These Future Scenes can be used to practice and apply the creative problem solving process.
Skip to content
Curriculum design: Problem-centred curriculum
- Research Summary
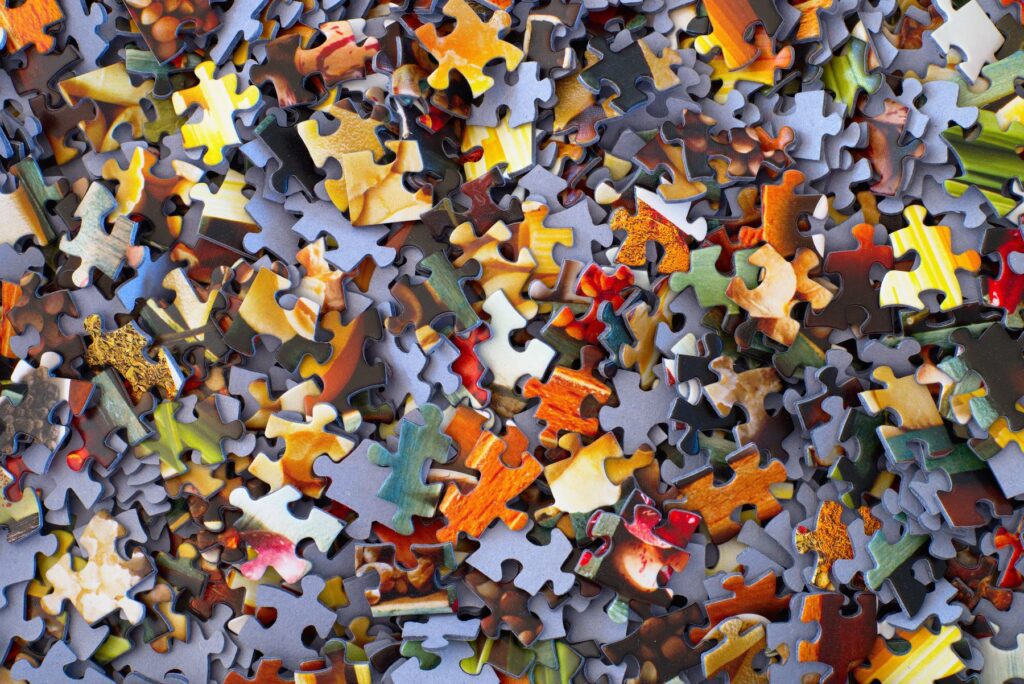
- Collaboration |
- Cross-Curricular |
- Curriculum |
- Developing effective learners |
- Effective instruction |
- Enrichment |
- Expert teacher |
- Innovation |
- Leadership |
- Lifelong learning |
- Professional learning |
- Project-based learning |
- Research Engagement |
- Self-directed learning |
- Self-regulation
What’s the idea?
A problem-centred curriculum is a cross-curricular, inquiry-based, student-centred approach that is built around real-world problems. Within a problem-centred curriculum, learning transcends subject disciplines and brings together elements of the curriculum that would otherwise be separate. It is designed to develop skilful, knowledgeable, collaborative, self-regulated and self-determined lifelong learners (Casey and Tucker, 1994; Hmelo-Silver, 2004; Savery, 2006).
What does it mean?
A problem-centred curriculum has its philosophical roots in constructivism, whereby knowledge is built through the students’ active participation in the learning process. A problem-centred curriculum places learning in the context of real-world problems that make students want to solve them (Hmelo-Silver, 2004). Problem-centred curriculum models take a ‘learner-centered approach that empowers learners to conduct research, integrate theory and practice, and apply knowledge and skills to develop a viable solution to a defined problem.’ (Savery, 2006, p. 12).
Casey and Tucker (1994) suggest the purpose of a problem-centred curriculum is to develop effective learners, which they define as students who are able to solve problems and harness their creativity through their planning and organisational skills. The purpose, therefore, of a problem-centred curriculum is to develop creative problem solvers who:
- are constantly curious and questioning
- enjoy figuring things out
- seek out challenges
- are persistent
- are resourceful and flexible
- are independent learners
- feel confident about themselves as learners
- are risk-takers (Casey and Tucker, 1994).
As problem-centred learning is student-centred, the role of the teacher is to act as more of a facilitator – posing problems and guiding the learner. Other characteristics that define a problem-centred curriculum are students being responsible for their own learning through self-regulation and self-determination, student collaboration, free-inquiry, and interdisciplinary learning (Hmelo-Silver, 2004; Savery, 2006).
This curriculum model involves integrating both the content (what is being learned) and the process (how it is being learned). While knowledge acquisition is important, the main focus of a problem-centred curriculum is on developing competencies such as team work, problem-solving skills, higher-order thinking skills, and self-directed learning skills which are transferable across disciplines (Savery, 2006). Students should be given opportunities to reflect on what they have learnt and the effectiveness of the strategies they employed (Hmelo-Silver, 2004; Savery, 2006).
What are the implications for teachers?
- How can schools design problems and challenges that are real, purposeful and impactful in the wider world, rather than falsify or ‘create’ these so that they pretend to be ‘real’?
- How can schools ensure that problems are loosely structured and allow for free enquiry so that there is a number of ways they can be solved?
- How will students’ work be managed when working on multidisciplinary problem-based projects?
- How will assessment operate within a problem-based curriculum? For example, will students submit portfolios rather than sit examinations?
Want to know more?
- Casey MB and Tucker EC (1994) Problem-centered classrooms: Creating lifelong learners. The Phi Delta Kappan 76(2): 139–143.
- Hmelo-Silver CE (2004) Problem-based learning: What and how do students learn? Educational Psychology Review 16(3): 235–266.
- Savery JR (2006) Overview of problem-based learning: Definitions and distinctions. Interdisciplinary Journal of Problem-Based Learning 1(1). Available at: https://doi.org/10.7771/1541-5015.1002 (accessed 22 May 2019).
Other content you may be interested in
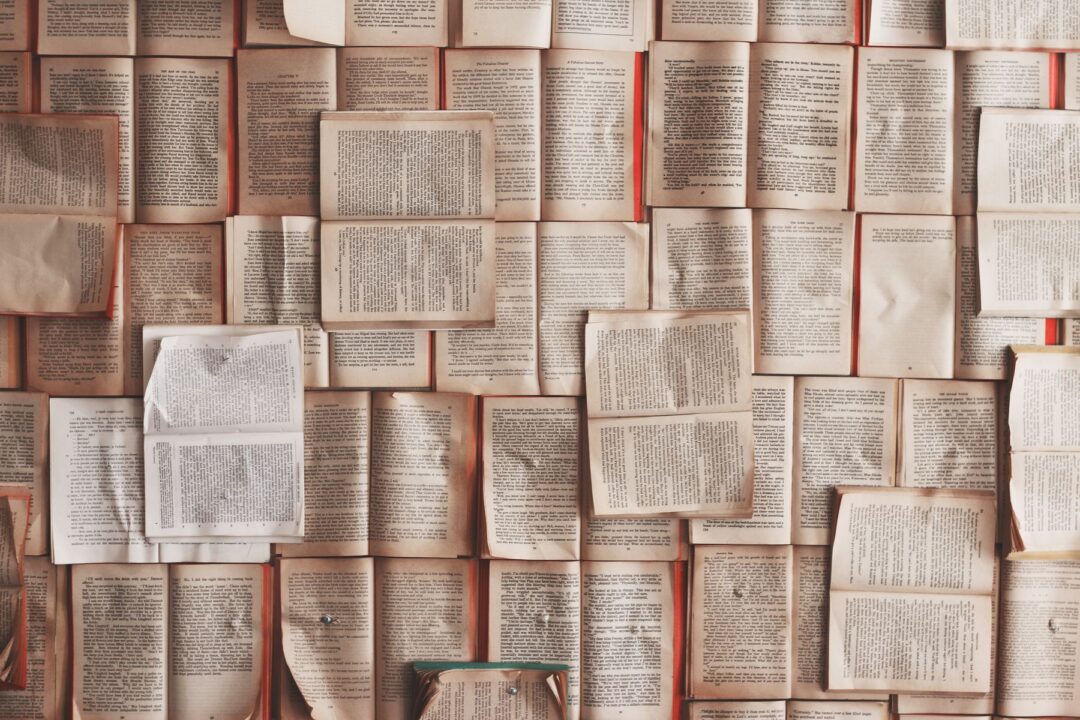
Great teaching techniques: Whole-class reading
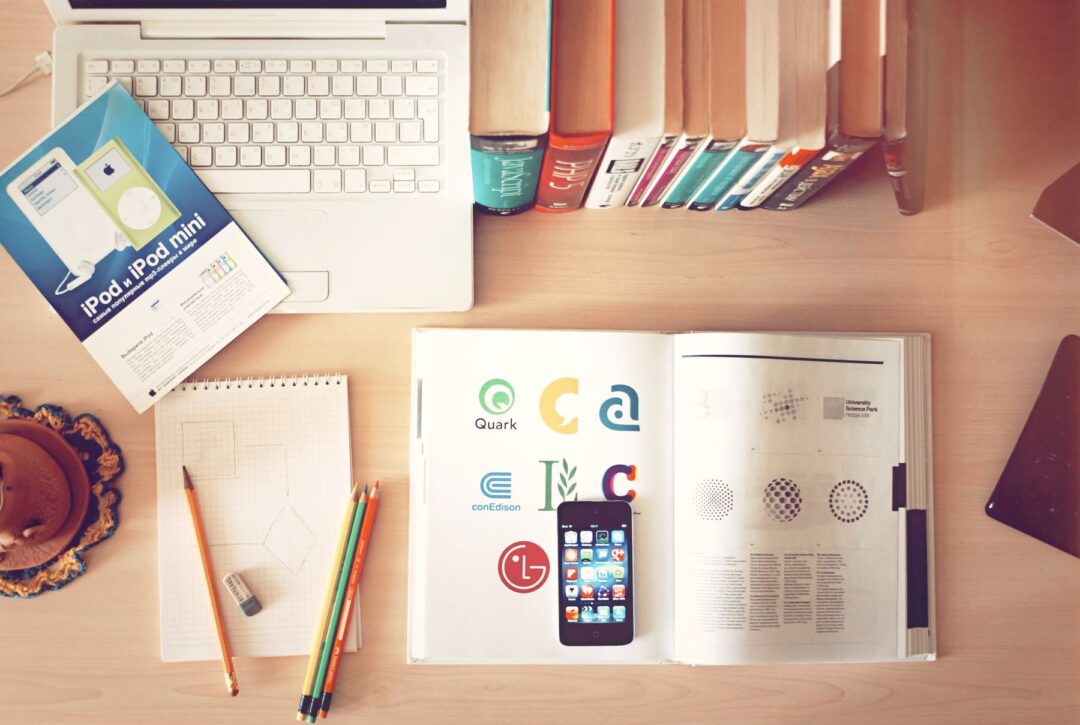
Checking pupil understanding using online quizzes
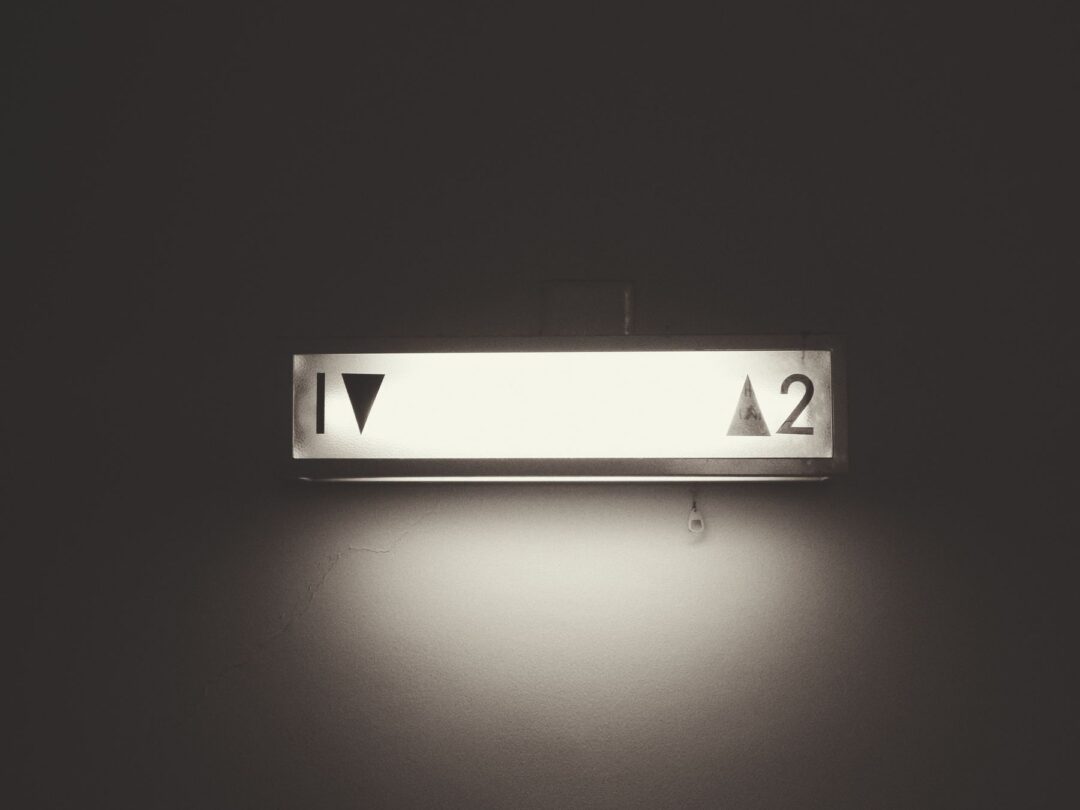
Q: How can we improve teacher retention? A: professional development
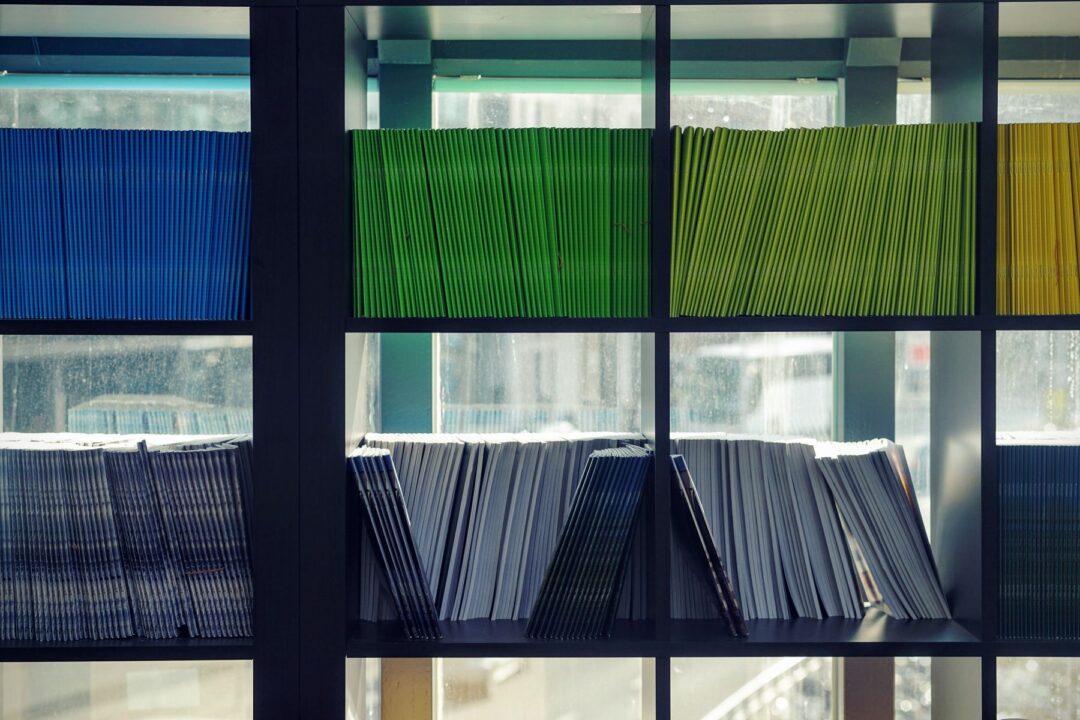
Teachers’ engagement with research
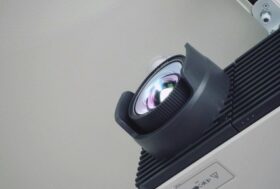
Improving PowerPoint presentations
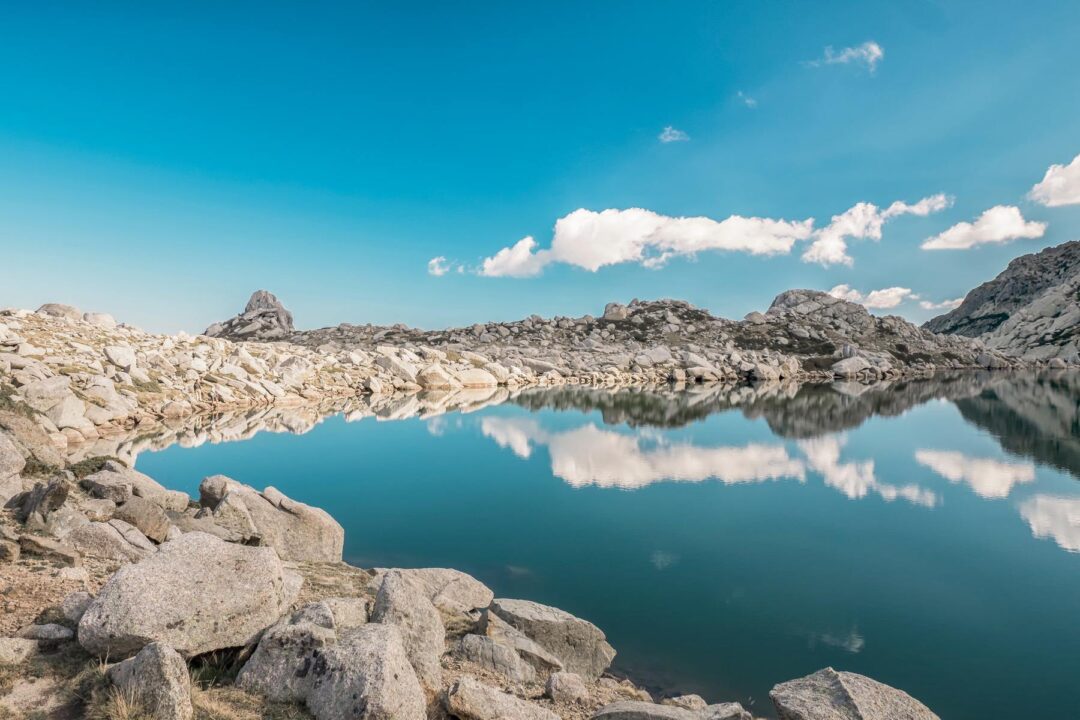
Assessment and feedback in an online context: Self assessment
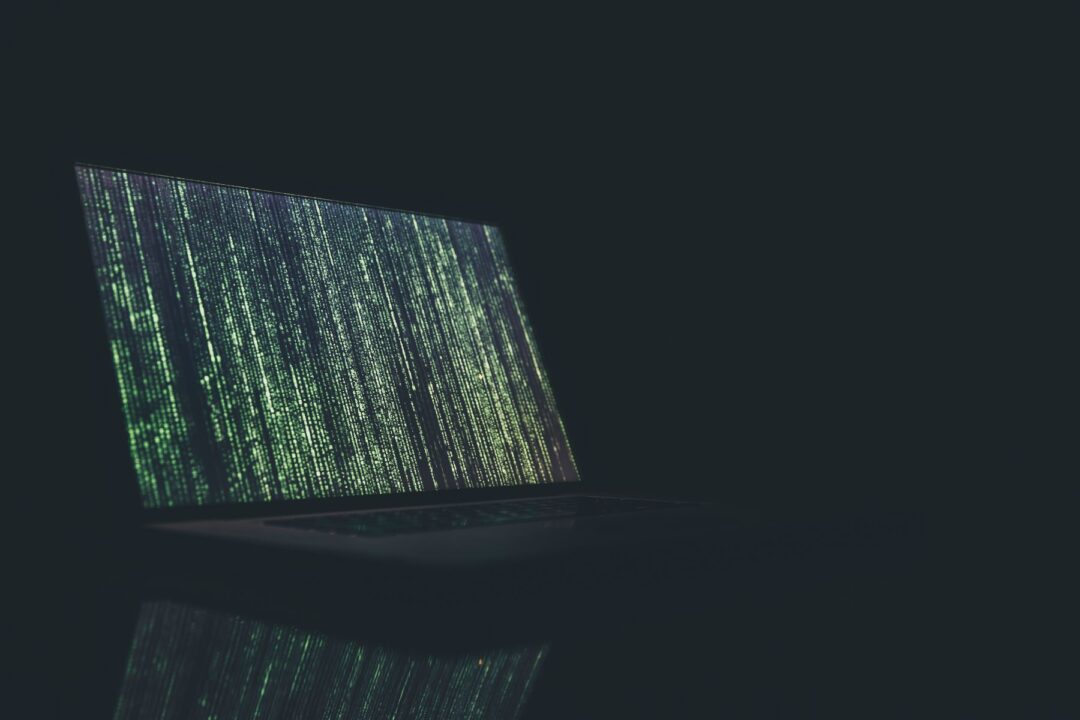
Hosting material online
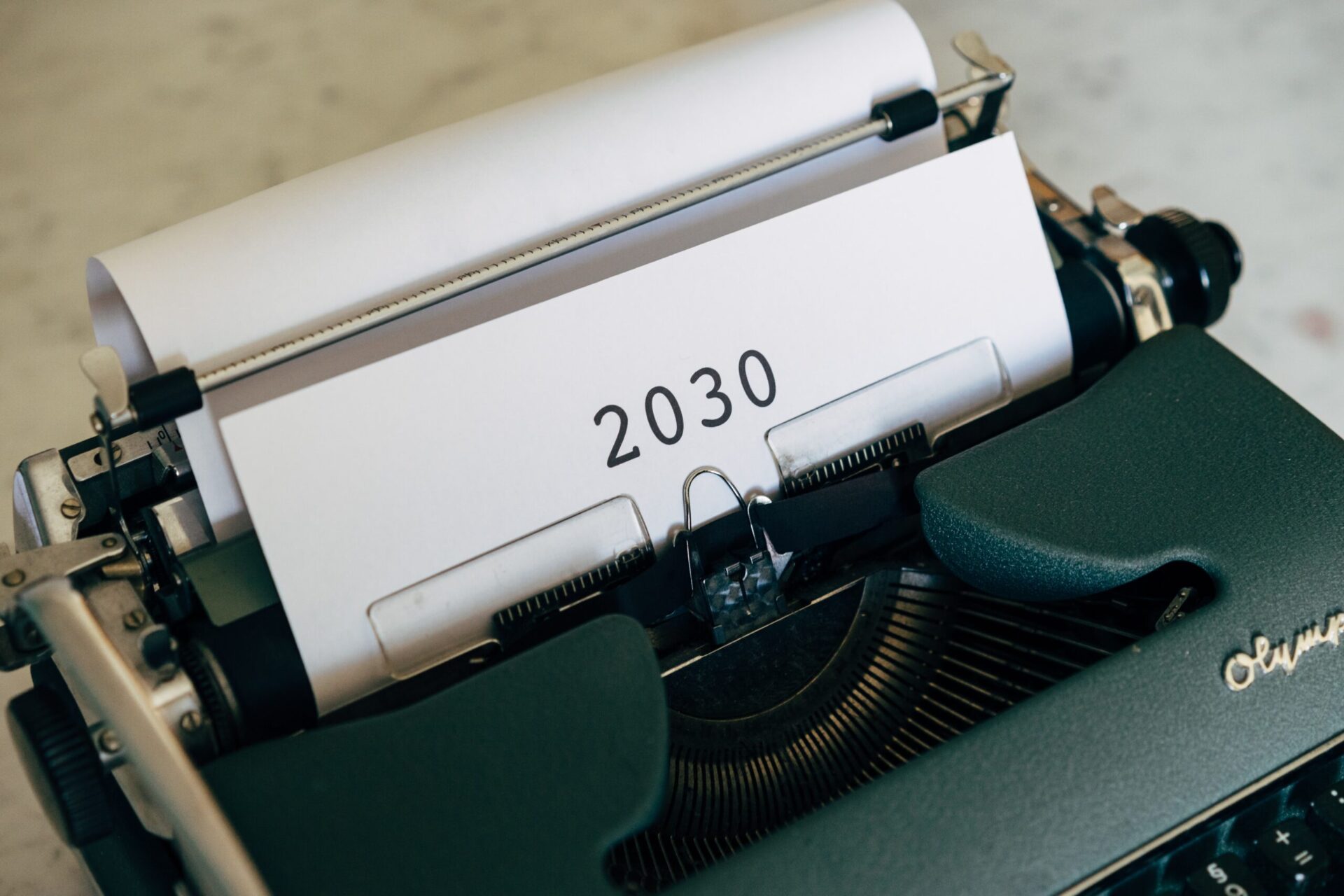
Implementing the Sustainable Development Goals through social action in primary schools
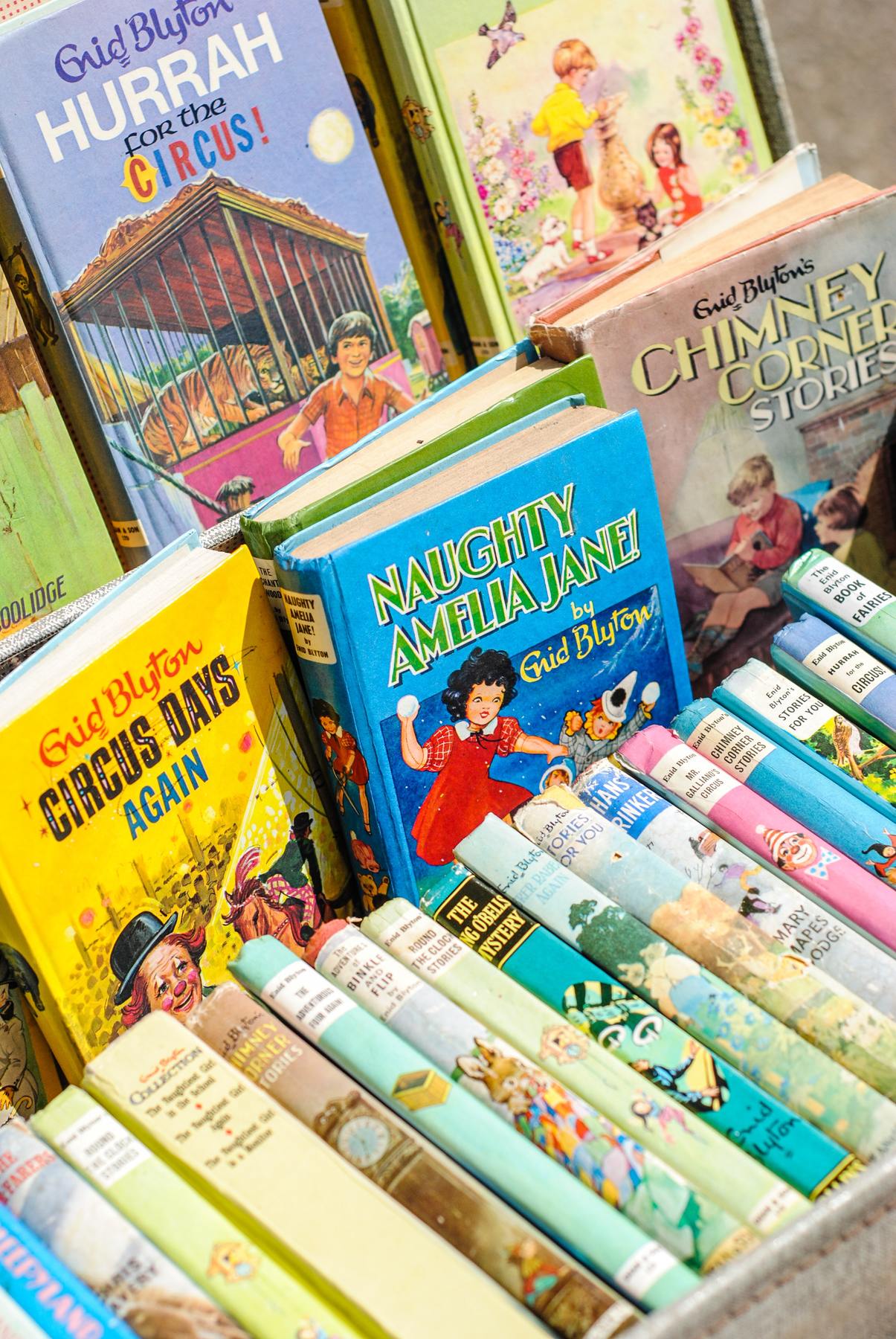
Could picture books improve your students’ language and literacy skills?
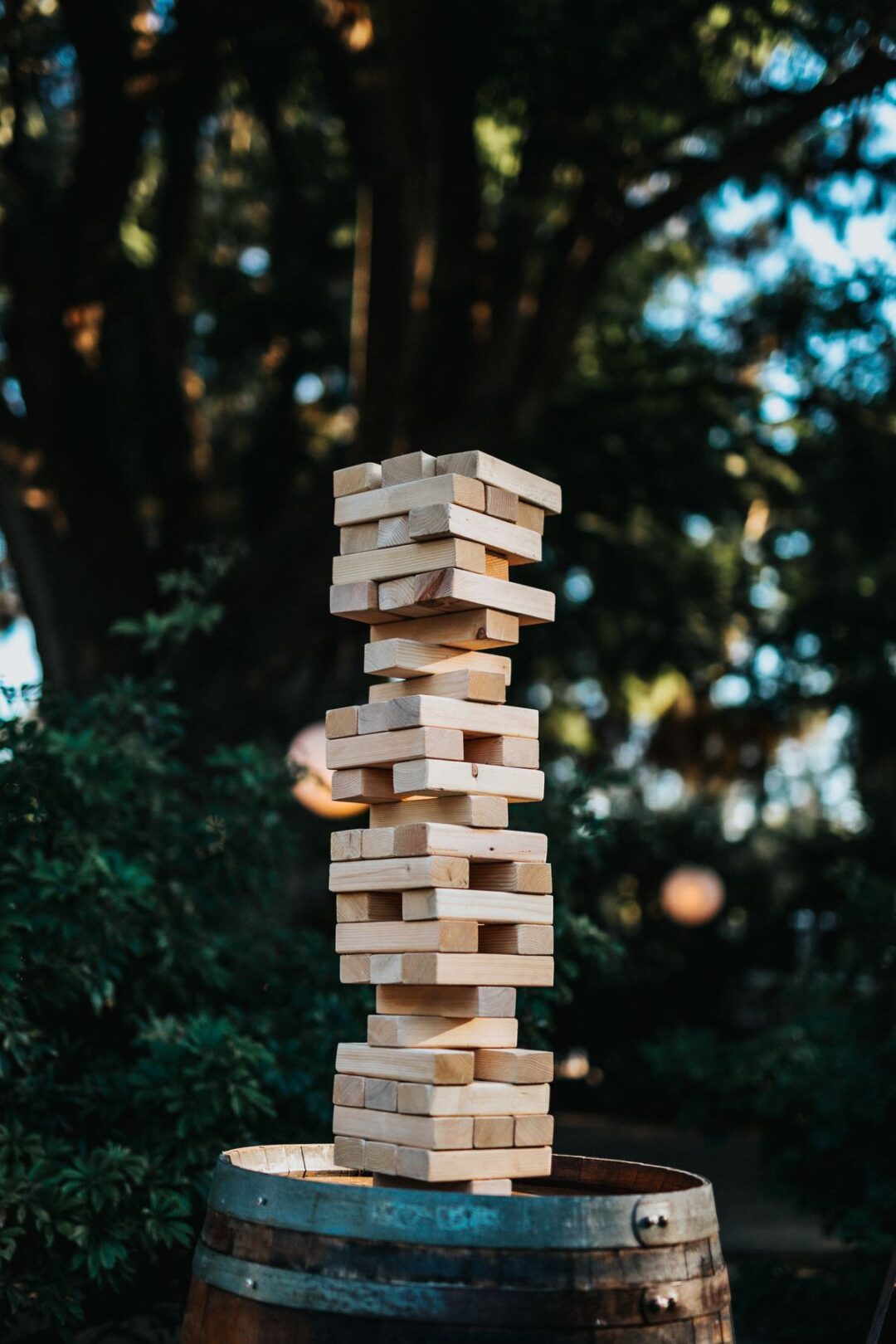
Curriculum design: The curriculum design process
Pears Pavillion Corum Campus 41 Brunswick Square London WC1N 1AZ
[email protected] 020 3433 7624
How to Incorporate Problem Solving into Your Curriculum
Posted August 25, 2022 by Miranda Marshall

With schools across the United States getting started this month, now is the time many teachers are getting to know their classes, subjects, and maybe even grade levels. During this critical planning period, there are educational standards that need to be met at the national, state, and district levels. It can be stressful thinking about how to make sure students aren’t just building the hard skills they’ll need for testing, but also the soft skills that they’ll carry with them into higher education and/or their future careers – such as problem solving.
At every age, problem solving skills help students think critically and strategically about the world around them. Not only is problem-solving necessary in the classroom and during exams like SATs and ACTs, it can also encourage creativity and inspire students to pursue new knowledge and career pathways.
Problem solving is essential in every classroom, no matter the subject area, but it can be tricky finding organic ways to incorporate it into your curriculum. One of the best ways to help students hone their problem solving skills is to have your students participate in an academic competition! Competitions like Future Problem Solving Program International (FPSPI) cater to students in multiple age ranges, and work with educators to curate the best experience for your students and their individual needs.
In this blog post, we will detail how you can integrate problem-solving into your curriculum for all grade levels – from kindergarten to high school – through FPSPI.

1. Check out their affordable, non-competitive resources for teachers.
FPSPI offers three different resources for educators who want to teach problem solving without guidance or the challenge environment:
- — Action-Based Problem Solving (ABPS) – available for students K-9 to provide guidance in the problem solving process and writing of ideas.
- — The Problem Solving Experience Curriculum – targeted at grades 5-8 to be implemented either as a full course, or spread out over 1-4 years, with complete lesson plans included.
- — Problem Solving Across the Curriculum (PSAC) – provides hundred of Future Scenes that can be modified for any classroom or grade level to give students the opportunity to apply the problem solving process.

2. Tackle global issues in the classroom.
Global Issues Problem Solving (GIPS) is available to teams or individual students who want to make a positive impact on the world. It can be used as a curriculum, integrated into existing content plans, or completed outside of school hours.
Participating students research global topics and resolve a Future Scene (a hypothetical scenario) by creating a detailed action plan.
3. Enact local change.
Community Problem Solving (CmPS) is also available to teams or individual students who want to explore the world around them, particularly issues that are close to home. CPS participants may also choose to address global concerns with their projects, but work to resolve the problems they choose by developing and carrying out their projects in the real world.

4. Get creative with performance or writing.
Scenario Writing (SW) and Scenario Performance (ScP) competitions call upon individual students to
respond to one of the five annual topics through either a 1500-word comprehensive essay or through an oral presentation, respectively. In both instances, students are asked to create stories that logically detail the outcomes of events taking place in the future.
Feedback from coaches:
[FPSPI] teaches important life skills.
[FPSPI helped our students with] developing advanced thinking skills.
FPS is challenging and involves hard work.
Want to learn more about how you can get your students involved in FPSPI? Check out the benefits of the program and get started this school year or join the 30-minute Intro to FPS Webinar on September 14th !
Signup Newsletter
Sign me up for the newsletter!

The Institute of Competition Sciences (ICS) was founded in 2012 to help transform learning into an exciting challenge for all students. We exist to support students in realizing the full potential of their future.
Quick Links
- Competitions
- Privacy Policy
- Terms and Conditions
Connect with us on social media

Copyright © 2024 Institute of Competition Sciences. All rights reserved.

New Designs for School 5 Steps to Teaching Students a Problem-Solving Routine
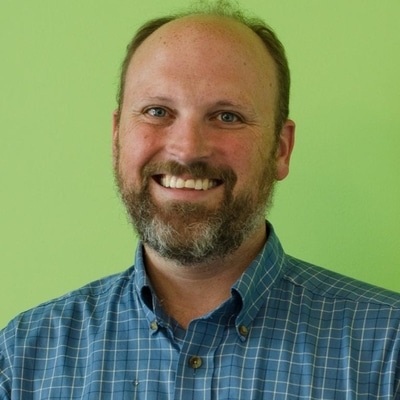
Jeff Heyck-Williams (He, His, Him) Director of the Two Rivers Learning Institute in Washington, DC
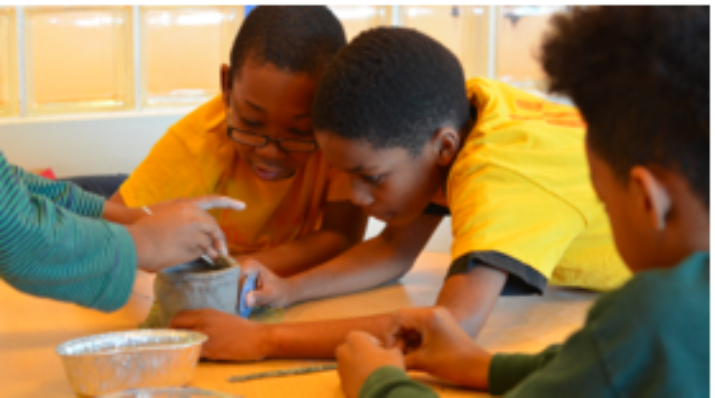
We’ve all had the experience of truly purposeful, authentic learning and know how valuable it is. Educators are taking the best of what we know about learning, student support, effective instruction, and interpersonal skill-building to completely reimagine schools so that students experience that kind of purposeful learning all day, every day.
Students can use the 5 steps in this simple routine to solve problems across the curriculum and throughout their lives.
When I visited a fifth-grade class recently, the students were tackling the following problem:
If there are nine people in a room and every person shakes hands exactly once with each of the other people, how many handshakes will there be? How can you prove your answer is correct using a model or numerical explanation?
There were students on the rug modeling people with Unifix cubes. There were kids at one table vigorously shaking each other’s hand. There were kids at another table writing out a diagram with numbers. At yet another table, students were working on creating a numeric expression. What was common across this class was that all of the students were productively grappling around the problem.
On a different day, I was out at recess with a group of kindergarteners who got into an argument over a vigorous game of tag. Several kids were arguing about who should be “it.” Many of them insisted that they hadn’t been tagged. They all agreed that they had a problem. With the assistance of the teacher they walked through a process of identifying what they knew about the problem and how best to solve it. They grappled with this very real problem to come to a solution that all could agree upon.
Then just last week, I had the pleasure of watching a culminating showcase of learning for our 8th graders. They presented to their families about their project exploring the role that genetics plays in our society. Tackling the problem of how we should or should not regulate gene research and editing in the human population, students explored both the history and scientific concerns about genetics and the ethics of gene editing. Each student developed arguments about how we as a country should proceed in the burgeoning field of human genetics which they took to Capitol Hill to share with legislators. Through the process students read complex text to build their knowledge, identified the underlying issues and questions, and developed unique solutions to this very real problem.
Problem-solving is at the heart of each of these scenarios, and an essential set of skills our students need to develop. They need the abilities to think critically and solve challenging problems without a roadmap to solutions. At Two Rivers Public Charter School in Washington, D.C., we have found that one of the most powerful ways to build these skills in students is through the use of a common set of steps for problem-solving. These steps, when used regularly, become a flexible cognitive routine for students to apply to problems across the curriculum and their lives.
The Problem-Solving Routine
At Two Rivers, we use a fairly simple routine for problem solving that has five basic steps. The power of this structure is that it becomes a routine that students are able to use regularly across multiple contexts. The first three steps are implemented before problem-solving. Students use one step during problem-solving. Finally, they finish with a reflective step after problem-solving.
Problem Solving from Two Rivers Public Charter School
Before Problem-Solving: The KWI
The three steps before problem solving: we call them the K-W-I.
The “K” stands for “know” and requires students to identify what they already know about a problem. The goal in this step of the routine is two-fold. First, the student needs to analyze the problem and identify what is happening within the context of the problem. For example, in the math problem above students identify that they know there are nine people and each person must shake hands with each other person. Second, the student needs to activate their background knowledge about that context or other similar problems. In the case of the handshake problem, students may recognize that this seems like a situation in which they will need to add or multiply.
The “W” stands for “what” a student needs to find out to solve the problem. At this point in the routine the student always must identify the core question that is being asked in a problem or task. However, it may also include other questions that help a student access and understand a problem more deeply. For example, in addition to identifying that they need to determine how many handshakes in the math problem, students may also identify that they need to determine how many handshakes each individual person has or how to organize their work to make sure that they count the handshakes correctly.
The “I” stands for “ideas” and refers to ideas that a student brings to the table to solve a problem effectively. In this portion of the routine, students list the strategies that they will use to solve a problem. In the example from the math class, this step involved all of the different ways that students tackled the problem from Unifix cubes to creating mathematical expressions.
This KWI routine before problem solving sets students up to actively engage in solving problems by ensuring they understand the problem and have some ideas about where to start in solving the problem. Two remaining steps are equally important during and after problem solving.
The power of teaching students to use this routine is that they develop a habit of mind to analyze and tackle problems wherever they find them.
During Problem-Solving: The Metacognitive Moment
The step that occurs during problem solving is a metacognitive moment. We ask students to deliberately pause in their problem-solving and answer the following questions: “Is the path I’m on to solve the problem working?” and “What might I do to either stay on a productive path or readjust my approach to get on a productive path?” At this point in the process, students may hear from other students that have had a breakthrough or they may go back to their KWI to determine if they need to reconsider what they know about the problem. By naming explicitly to students that part of problem-solving is monitoring our thinking and process, we help them become more thoughtful problem solvers.
After Problem-Solving: Evaluating Solutions
As a final step, after students solve the problem, they evaluate both their solutions and the process that they used to arrive at those solutions. They look back to determine if their solution accurately solved the problem, and when time permits they also consider if their path to a solution was efficient and how it compares to other students’ solutions.
The power of teaching students to use this routine is that they develop a habit of mind to analyze and tackle problems wherever they find them. This empowers students to be the problem solvers that we know they can become.
Jeff Heyck-Williams (He, His, Him)
Director of the two rivers learning institute.
Jeff Heyck-Williams is the director of the Two Rivers Learning Institute and a founder of Two Rivers Public Charter School. He has led work around creating school-wide cultures of mathematics, developing assessments of critical thinking and problem-solving, and supporting project-based learning.
Read More About New Designs for School
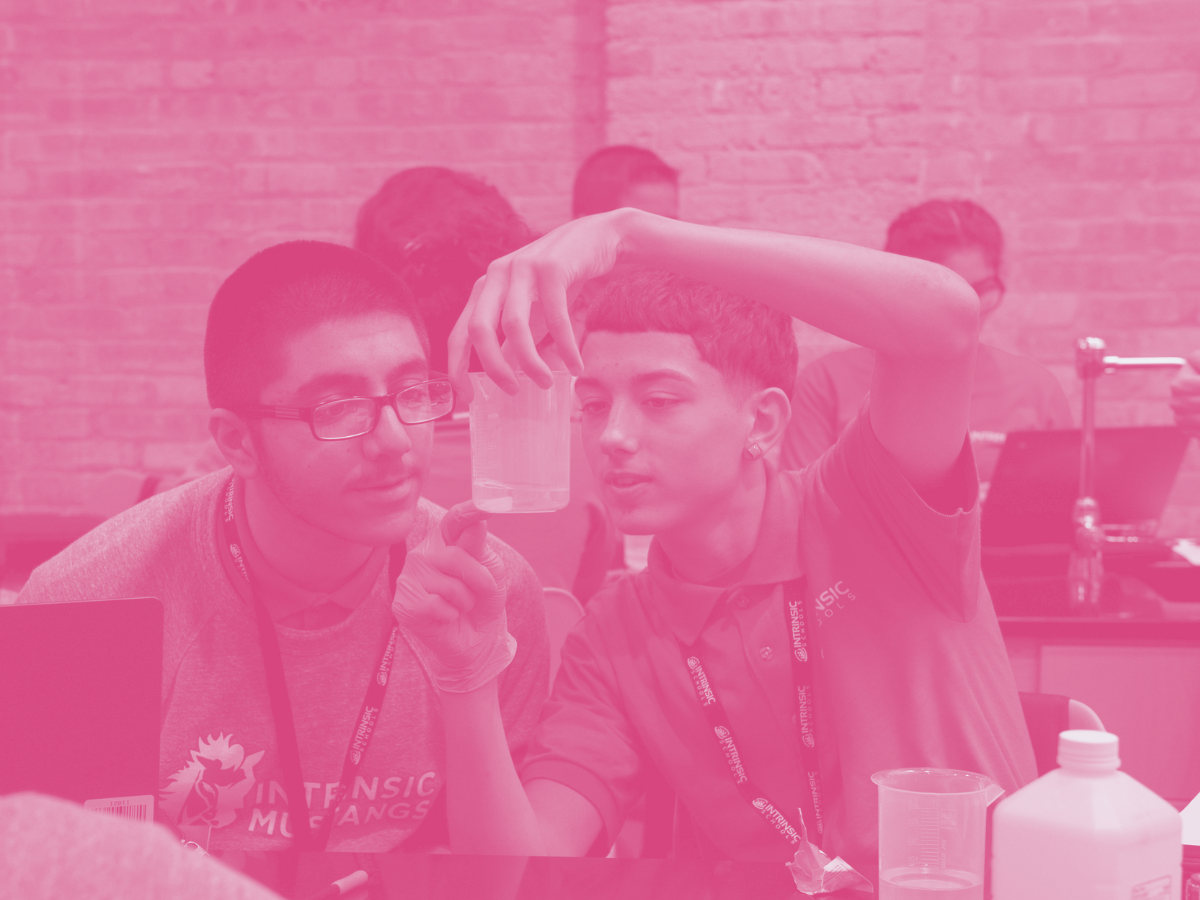
NGLC Invites Applications from New England High School Teams for Our Fall 2024 Learning Excursion
March 21, 2024
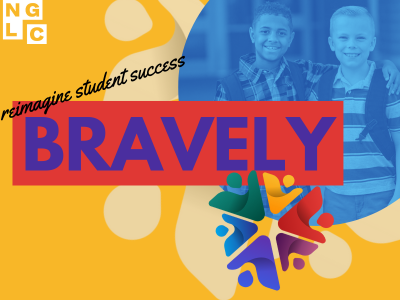
Bring Your Vision for Student Success to Life with NGLC and Bravely
March 13, 2024
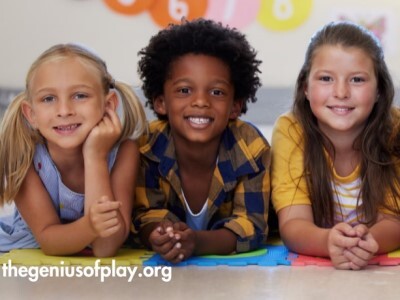
How to Nurture Diverse and Inclusive Classrooms through Play
Rebecca Horrace, Playful Insights Consulting, and Laura Dattile, PlanToys USA
March 5, 2024
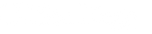
OUR PROBLEM-SOLVING CURRICULUM
Our curriculum features 13 modules that focus on building the relevant skills and knowledge for effective problem solving. We cover a wide variety of areas including problem identification, assumption reduction, and solution development.
Intro to
Problem Solving
Intro to problem-solving strategies, thought-provoking case studies, and problem-solving in history
Research and
How to perform relevant research, collect data, and survey. Various forms of bias in data.
Communication
The value of diversity in thought and perspective. Learn methods of communication and conflict de-escalation. Building effective-problem-solving environments.
Evaluation &
General guides for evaluating solutions. Learn to perform ethical evaluations (four virtues, ethics checklist, five P’s)
Overview of "What's the real problem?", Statement Restatement Techniques, Duncker Diagrams, and more
Determining all the assumptions both within the problem and within the solution. Performing assumption reduction based on limited resources
Scaling, Testing,
Risk Reduction
Perform risk reduction testing by identifying weak points in assumptions. How scaling affects assumptions and how to make meaningful reflection on data.
Mental Blocks,
Creative Thinking
Metacognitive approach to understanding and overcoming mental blocks. Strategies for promoting creativity.
Implementation
Persuasion, planning, resource management, coordination, deployment. Systems thinking strategies for deployment.
Problem reframing strategies to explore in and outside of problem-space. Solving a different problem to achieve the same desired outcome.
Development
Convergent-Divergent thinking, vertical and lateral thinking, futuring, cross-ferterilization, TRIZ
Troubleshooting
Troubleshooting process and heuristics using KT Analysis. SYL Problem ID Matrix.
Consequences
Solution in the intended operating environment and systems thinking strategies. Developing high leverage point solutions via causal loops.
An IERI – International Educational Research Institute Journal
- Open access
- Published: 10 December 2014
The acquisition of problem solving competence: evidence from 41 countries that math and science education matters
- Ronny Scherer 1 , 2 &
- Jens F Beckmann 3
Large-scale Assessments in Education volume 2 , Article number: 10 ( 2014 ) Cite this article
14k Accesses
16 Citations
1 Altmetric
Metrics details
On the basis of a ‘problem solving as an educational outcome’ point of view, we analyse the contribution of math and science competence to analytical problem-solving competence and link the acquisition of problem solving competence to the coherence between math and science education. We propose the concept of math-science coherence and explore whether society-, curriculum-, and school-related factors confound with its relation to problem solving.
By using the PISA 2003 data set of 41 countries, we apply multilevel regression and confounder analyses to investigate these effects for each country.
Our results show that (1) math and science competence significantly contribute to problem solving across countries; (2) math-science coherence is significantly related to problem solving competence; (3) country-specific characteristics confound this relation; (4) math-science coherence is linked to capability under-utilisation based on science performance but less on math performance.
Conclusions
In sum, low problem solving scores seem a result of an impeded transfer of subjectspecific knowledge and skills (i.e., under-utilisation of science capabilities in the acquisition of problem solving competence), which is characterised by low levels of math-science coherence.
The ability to solve real-world problems and to transfer problem-solving strategies from domain-specific to domain-general contexts and vice versa has been regarded an important competence students should develop during their education in school (Greiff et al. [ 2013 ]; van Merriënboer [ 2013 ]). In the context of large-scale assessments such as the PISA study problem solving competence is defined as the ability to solve cross-disciplinary and real-world problems by applying cognitive skills such as reasoning and logical thinking (Jonassen [ 2011 ]; OECD [ 2004 ]). Since this competence is regarded a desirable educational outcome, especially math and science educators have focused on developing students’ problem solving and reasoning competence in their respective domain-specific contexts (e.g., Kind [ 2013 ]; Kuo et al. [ 2013 ]; Wu and Adams [ 2006 ]). Accordingly, different conceptual frameworks were proposed that describe the cognitive processes of problem solving such as understanding the problem, building adequate representations of the problem, developing hypotheses, conducting experiments, and evaluating the solution (Jonassen [ 2011 ]; OECD [ 2005 ]). In comparing these approaches in math and science, it seems apparent that there is a conceptual overlap between the problem solving models in these two domains. This overlap triggers the question regarding its contribution to the development of students’ cross-curricular problem-solving competence (Abd-El-Khalick et al. [ 2004 ]; Bassok and Holyoak [ 1993 ]; Hiebert et al. [ 1996 ]).
The operationalization and scaling of performance in PISA assessments enables direct contrasting of scores in students’ competences in math and problem solving. Leutner et al. ([ 2012 ]) suggest that discrepancies between math and problem solving scores are indicative of the relative effectiveness of math education (OECD [ 2004 ]). In line with a “Capability-Utilisation Hypothesis”, it is assumed that math scores that negatively deviate from their problem solving counterpart signify an under-utilisation of students’ problem-solving capabilities as indicated by their scores in generic problem solving.
We intend to extend this view in two ways: First, by introducing the concept of math-science coherence we draw the focus on the potential synergistic link between math and science education and its contribution to the acquisition of problem solving competence. Second, the introduction of a Capability Under-Utilisation Index will enable us to extend the focus of the Capability-Utilisation Hypothesis to both, math and science education. The combination of the concept of math-science coherence with the notion of capability-utilisation will help to further explore the facilitating processes involved in the transition of subject-specific knowledge and skills to the acquisition of problem solving competence. These insights are expected to contribute to a better understanding of meaningful strategies to improve and optimize educational systems in different countries.
Theoretical framework
Problem solving as an educational goal.
In the PISA 2003 framework, problem solving is referred to as “an individual’s capacity to use cognitive processes to resolve real, cross-disciplinary situations where the solution path is not immediately obvious” (OECD [ 2004 ], p. 156). This definition is based on the assumption of domain-general skills and strategies that can be employed in various situations and contexts. These skills and strategies involve cognitive processes such as: Understanding and characterizing the problem, representing the problem, solving the problem, reflecting and communicating the problem solution (OECD [ 2003 ]). Problem solving is often regarded a process rather than an educational outcome, particularly in research on the assessment and instruction of problem solving (e.g., Greiff et al. [ 2013 ]; Jonassen [ 2011 ]). This understanding of the construct is based on the assumption that problem solvers need to perform an adaptive sequence of cognitive steps in order to solve a specific problem (Jonassen [ 2011 ]). Although problem solving has also been regarded as a process skill in large-scale assessments such as the PISA 2003 study, these assessments mainly focus on problem solving performance as an outcome that can be used for international comparisons (OECD [ 2004 ]). However, problem solving competence was operationalized as a construct comprised of cognitive processes. In the context of the PISA 2003 study, these processes were referred to as analytical problem solving, which was assessed by static tasks presented in paper-and-pencil format. Analytical problem-solving competence is related to school achievement and the development of higher-order thinking skills (e.g., Baumert et al. [ 2009 ]; OECD [ 2004 ]; Zohar [ 2013 ]). Accordingly, teachers and educators have focused on models of problem solving as guidelines for structuring inquiry-based processes in their subject lessons (Oser and Baeriswyl [ 2001 ]). Van Merriënboer ([ 2013 ]) pointed out that problem solving should not only be regarded a mere instructional method but also as a major educational goal. Recent curricular reforms have therefore shifted towards the development of problem solving abilities in school (Gallagher et al. [ 2012 ]; Koeppen et al. [ 2008 ]). These reforms were coupled with attempts to strengthen the development of transferable skills that can be applied in real-life contexts (Pellegrino and Hilton [ 2012 ]). For instance, in the context of 21 st century skills, researchers and policy makers have agreed on putting emphasis on fostering skills such as critical thinking, digital competence, and problem solving (e.g., Griffin et al. [ 2012 ]). In light of the growing importance of lifelong learning and the increased complexity of work- and real-life problem situations, these skills are now regarded as essential (Griffin et al. [ 2012 ]; OECD [ 2004 ]). Hence, large-scale educational studies such as PISA have shifted towards the assessment and evaluation of problem solving competence as a 21 st century skill.
The PISA frameworks of math and science competence
In large-scale assessments such as the PISA studies, students’ achievement in the domains of science and mathematics play an important role. Moreover, scientific and mathematical literacy are now regarded essential to being a reflective citizen (Bybee [ 2004 ]; OECD [ 2003 ]). Generally, Baumert et al. ([ 2009 ]) have shown that students’ math and science achievements are highly related to domain-general ability constructs such as reasoning or intelligence. In this context, student achievement refers to “the result of domain-specific processes of knowledge acquisition and information processing” (cf. Baumert et al. [ 2009 ], p. 169). This line of argument is reflected in definitions and frameworks of scientific and mathematical literacy, which are conceptualized as domain-specific competences that are hierarchically organized and build upon abilities closely related to problem solving (Brunner et al. [ 2013 ]).
Scientific literacy has been defined within a multidimensional framework, differentiating between three main cognitive processes, namely describing, explaining, and predicting scientific phenomena, understanding scientific investigations, and interpreting scientific evidence and conclusions (OECD [ 2003 ]). In addition, various types of knowledge such as ‘knowledge about the nature of science’ are considered as factors influencing students’ achievements in this domain (Kind [ 2013 ]). We conclude that the concept of scientific literacy encompasses domain-general problem-solving processes, elements of scientific inquiry (Abd-El-Khalick et al. [ 2004 ]; Nentwig et al. [ 2009 ]), and domain-specific knowledge.
The definition of mathematical literacy refers to students’ competence to utilise mathematical modelling and mathematics in problem-solving situations (OECD [ 2003 ]). Here, we can also identify overlaps between cognitive processes involved in mathematical problem solving and problem solving in general: Structuring, mathematizing, processing, interpreting, and validating (Baumert et al. [ 2009 ]; Hiebert et al. [ 1996 ]; Kuo et al. [ 2013 ]; Polya [ 1945 ]). In short, mathematical literacy goes beyond computational skills (Hickendorff [ 2013 ]; Wu and Adams [ 2006 ]) and is conceptually linked to problem solving.
In the PISA 2003 framework, the three constructs of math, science, and problem solving competence overlap conceptually. For instance, solving the math items requires reasoning, which comprises analytical skills and information processing. Given the different dimensions of the scientific literacy framework, the abilities involved in solving the science items are also related to problem solving, since they refer to the application of knowledge and the performance of inquiry processes (OECD [ 2003 ]). This conceptual overlap is empirically supported by high correlations between math and problem solving ( r = .89) and between science and problem solving ( r = .80) obtained for the sample of 41 countries involved in PISA 2003 (OECD [ 2004 ]). The relation between math and science competence was also high ( r = .83). On the one hand, the sizes of the inter-relationships, give rise to the question regarding the uniqueness of each of the competence measures. On the other hand, the high correlations indicate that problem-solving skills are relevant in math and science (Martin et al. [ 2012 ]). Although Baumert et al. ([ 2009 ]) suggest that the domain-specific competences in math and science require skills beyond problem solving (e.g., the application of domain-specific knowledge) we argue from an assessment perspective that the PISA 2003 tests in math, science, and problem solving measure predominantly basic academic skills relatively independent from academic knowledge (see also Bulle [ 2011 ]).
The concept of capability-utilisation
Discrepancies between students’ performance in math/science and problem solving were studied at country level (OECD [ 2004 ]) and were, for example for math and problem solving scores, interpreted in two ways: (1) If students’ perform better in math than in problem solving, they would “have a better grasp of mathematics content […] after accounting for the level of generic problem-solving skills…” (OECD [ 2004 ], p. 55); (2) If students’ estimated problem-solving competence is higher than their estimated math competence, “… this may suggest that students have the potential to achieve better results in mathematics than that reflected in their current performance…” (OECD [ 2004 ], p. 55). Whilst the latter discrepancy constitutes a capability under-utilisation in math, the former suggests challenges in utilising knowledge and skills acquired in domain-specific contexts in domain-unspecific contexts (i.e., transfer problem).
To quantify the degree to which students are able to transfer their problem solving capabilities from domain-specific problems in math or science to cross-curricular problems, we introduce the Capability Under-Utilisation Index (CUUI) as the relative difference between math or science and problem solving scores:
A positive CUUI indicates that the subject-specific education (i.e., math or science) in a country tends to under-utilise its students’ capabilities to problem solve. A negative CUUI indicates that a country’s educational system fails to fully utilise its students’ capabilities to acquire math and science literacy in the development of problem solving. The CUUI reflects the relative discrepancies between the achievement scores in different domains a .
The concept of math-science coherence
In light of the conceptual and empirical discussion on the relationship between math, science, and problem solving competence, we introduce the concept of math-science coherence as follows: First, math-science coherence refers to the set of cognitive processes involved in both subjects and thus represents processes which are related to reasoning and information processing, relatively independent from domain-specific knowledge. Second, math-science coherence reflects the degree to which math and science education is harmonized as a feature of the educational environment in a country. This interpretation is based on the premise that PISA measures students’ competence as educational outcomes (OECD [ 2004 ]). The operationalization of math-science coherence is realized by means of the correlation between math and science scores [ r (M,S)] at the country level. Low math-science coherence indicates that students who are successful in the acquisition of knowledge and skills in math are not necessarily successful in the acquisition of knowledge and skills in science and vice versa.
On the basis of this conceptualization of math-science coherence, we expect a significant and positive relation to problem solving scores, since the conceptual overlap between mathematical and scientific literacy refers to cognitive abilities such as reasoning and information processing that are also required in problem solving (Arts et al. [ 2006 ]; Beckmann [ 2001 ]; Wüstenberg et al. [ 2012 ]). Hence, we assert that math-science coherence facilitates the transfer of knowledge, skills, and insights across subjects resulting in better problem solving performance (OECD [ 2004 ]; Pellegrino and Hilton [ 2012 ]).
We also assume that math-science coherence as well as capability utilisation is linked to characteristics of the educational system of a country. For instance, as Janssen and Geiser ([ 2012 ]) and Blömeke et al. ([ 2011 ]) suggested, the developmental status of a country, measured by the Human Development Index (HDI; UNDP [ 2005 ]), is positively related to students’ academic achievements as well as to teachers’ quality of teaching. Furthermore, the socio-economic status of a country co-determines characteristics of its educational system, which ultimately affects a construct referred to as national intelligence (Lynn and Meisenberg [ 2010 ]). Research also indicated that curricular settings and educational objectives are related to school achievement in general (Bulle [ 2011 ]; Martin et al. [ 2004 ]). Besides these factors, school- and classroom-related characteristics might also confound the relation between math-science coherence and problem solving. For instance, the schools’ autonomy in developing curricula and managing educational resources might facilitate the incorporation of inquiry- and problem-based activities in science lessons (Chiu and Chow [ 2011 ]). These factors have been discussed as being influential to students’ competence development (OECD [ 2004 ], [ 2005 ]). Ewell ([ 2012 ]) implies that cross-national differences in problem solving competence might be related to differences in education and in using appropriate teaching material. These factors potentially confound the relation between math-science coherence and problem solving.
Discrepancies between math and problem solving scores are discussed in relation to quality of education. Although research has found that crossing the borders between STEM subjects positively affects students’ STEM competences (e.g., National Research Council NRC [ 2011 ]), we argue that the PISA analyses have fallen short in explaining cross-country differences in the development of problem solving competence, since they ignored the link between math and science competences and the synergistic effect of learning universally applicable problem-solving skills in diverse subject areas. Hence, we use the concept of math-science coherence to provide a more detailed description of the discrepancies between problem solving and domain-specific competences. In this regard, we argue that the coherence concept indicates the synergistic potential and students’ problem-solving competence the success of transfer.
The present study
The current study is based on the premise that in contrast to math and science competence problem solving competence is not explicitly taught as a subject at school. Problem solving competence, however, is an expected outcome of education (van Merriënboer [ 2013 ]). With the first step in our analyses, we seek to establish whether math and science education are in fact main contributors to the acquisition of problem solving competence. On the basis of this regression hypothesis, we subsequently focus on the question whether there are significant and systematic differences between countries ( Moderation-Hypothesis ). In light of the conceptual overlap due to cognitive processes involved in dealing with math, science and problem solving tasks and the shared item format employed in the assessments, we expect math and science competence scores to substantially predict scores in problem solving competence. Furthermore, since math and science education are differently organized across the 41 countries participating in the PISA 2003 study, differences in the contribution are also expected.
On the basis of these premises, we introduce the concept of math-science coherence, operationalised as the correlation between math and science scores [ r (M,S)], and analyse its relationship to problem solving and the effects of confounders (i.e., country characteristics) as a step of validation. Since math, science, and problem solving competence show a conceptual overlap, we expect problem solving and math-science coherence to be positively related. Countries’ educational systems differ in numerous aspects, their educational structure, and their educational objectives. Countries also differ with regard to the frequency of assessments, the autonomy of schools in setting up curricula and resources, and the educational resources available. Consequently, we expect the relation between math-science coherence and problem solving competence to be confounded by society-, curriculum-, and school-related factors ( Confounding-Hypothesis ).
In a final step, we aim to better understand the mechanisms with which math and science education contributes to the acquisition of problem-solving competence by exploring how math-science coherence, capability utilisation, and problem solving competence are related. We thus provide new insights into factors related to the transfer between students’ domain-specific and cross-curricular knowledge and skills ( Capability-Utilisation Hypothesis ).
In PISA 2003, a total sample of N = 276,165 students (49.4% female) from 41 countries participated. The entire sample was randomly selected by applying a two-step sampling procedure: First, schools were chosen within a country. Second, students were chosen within these schools. This procedure consequently led to a clustered structure of the data set, as students were nested in 10,175 schools. On average, 27 students per school were chosen across schools within countries. Students’ mean age was 15.80 years ( SD = 0.29 years) ranging from 15.17 to 16.25 years.
In the PISA 2003 study, different assessments were used in order to measure students’ competence in math, science, and problem solving. These assessments were administered as paper-and-pencil tests within a multi-matrix design (OECD [ 2005 ]). In this section, the assessments and further constructs are described that served as predictors of the contribution of math and science competence to problem solving at the country level.
Student achievement in math, science, and problem solving
In order to assess students’ competence to solve cross-curricular problems (i.e., analytical problem solving requiring information retrieval and reasoning), students had to work on an analytical problem-solving test. This test comprised a total of 19 items (7 items referred to trouble-shooting, 7 items referred to decision-making, and 5 items referred to system analysis and design; see OECD [ 2004 ]). Items were coded according to the PISA coding scheme, resulting in dichotomous and polytomous scores (OECD [ 2005 ]). Based on these scores, models of item response theory were specified in order to obtain person and item parameters (Leutner et al. [ 2012 ]). The resulting plausible values could be regarded as valid indicators of students’ abilities in problem solving (Wu [ 2005 ]). The problem solving test showed sufficient reliabilities between .71 and .89 for the 41 countries.
To assess mathematical literacy as an indicator of math competence , an 85-items test was administered (for details, refer to OECD [ 2003 ]). Responses were dichotomously or polytomously scored. Again, plausible values were obtained as person ability estimates and reliabilities were good (range: 0.83 – 0.93). In the context of mathematical literacy, students were asked to solve real-world problems by applying appropriate mathematical models. They were prompted to “identify and understand the role mathematics plays in the world, to make well-founded judgements and to use […] mathematics […]” (OECD [ 2003 ], p. 24).
Scientific literacy as a proxy for science competence was assessed by using problems referring to different content areas of science in life, health, and technology. The reliability estimates for the 35 items in this test ranged between .68 and .88. Again, plausible values served as indicators of this competence.
Country-specific characteristics
In our analyses, we incorporated a range of country-specific characteristics that can be subdivided into three main categories. These are: society-related factors, curriculum-related factors, and school-related factors. Country-specific estimates of National Intelligence as derived by Lynn and Meisenberg ([ 2010 ]) as well as the Human Development Index (HDI) were subsumed under society-related factors . The HDI incorporates indicators of a country’s health, education, and living standards (UNDP [ 2005 ]). Both variables are conceptualised as factors that contribute to country-specific differences in academic performance.
Holliday and Holliday ([ 2003 ]) emphasised the role of curricular differences in the understanding of between-country variance in test scores. We incorporated two curriculum-related factors in our analyses. First, we used Bulle’s ([ 2011 ]) classification of curricula into ‘progressive’ and ‘academic’. Bulle ([ 2011 ]) proposed this framework and classified the PISA 2003 countries according to their educational model. In her framework, she distinguishes between ‘academic models’ which are primarily geared towards teaching academic subjects (e.g., Latin, Germanic, and East-Asian countries) and ‘progressive models’ which focus on teaching students’ general competence in diverse contexts (e.g., Anglo-Saxon and Northern countries). In this regard, academic skills refer to the abilities of solving academic-type problems, whereas so called progressive skills are needed in solving real-life problems (Bulle [ 2011 ]). It can be assumed that educational systems that focus on fostering real-life and domain-general competence might be more conducive to successfully tackling the kind of problem solving tasks used in PISA (Kind [ 2013 ]). This classification of educational systems should be seen as the two extreme poles of a continuum rather than as a strict dichotomy. In line with the reflections above, we would argue that academic and progressive skills are not exclusively distinct, since both skills utilise sets of cognitive processes that largely overlap (Klahr and Dunbar [ 1988 ]). The fact that curricular objectives in some countries are shifting (e.g., in Eastern Asia) makes a clear distinction between both models even more difficult. Nonetheless, we will use this form of country-specific categorization based on Bulle’s model in our analyses.
Second, we considered whether countries’ science curricula were ‘integrated’ or ‘not integrated’ (Martin et al. [ 2004 ]). In this context, integration refers to linking multiple science subjects (biology, chemistry, earth science, and physics) to a unifying theme or issue (cf. Drake and Reid [ 2010 ], p. 1).
In terms of school-related factors, we used the PISA 2003 scales of ‘Frequency of assessments in schools’, ‘Schools’ educational resources’, and ‘School autonomy towards resources and curricula’ from the school questionnaire. Based on frequency and rating scales, weighted maximum likelihood estimates (WLE) indicated the degree to which schools performed in these scales (OECD [ 2005 ]).
The country-specific characteristics are summarized in the Table 1 .
The PISA 2003 assessments utilised a randomized incomplete block design to select different test booklets which covered the different content areas of math, science, and problem solving (Brunner et al. [ 2013 ]; OECD [ 2005 ]). The test administration took 120 minutes, and was managed for each participating country separately. It was established that quality standards of the assessment procedure were high.
Statistical analyses
In PISA 2003, different methods of obtaining person estimates with precise standard errors were applied. The most accurate procedure produced five plausible values, which were drawn from a person ability distribution (OECD [ 2005 ]). To avoid missing values in these parameters and to obtain accurate estimates, further background variables were used within the algorithms (Wu [ 2005 ]). The resulting plausible values were subsequently used as indicators of students’ competence in math, science, and problem solving. By applying Rubin’s combination rules (Bouhilila and Sellaouti [ 2013 ]; Enders [ 2010 ]), analyses were replicated with each of the five plausible values and then combined. In this multiple imputation procedure, standard errors were decomposed to the variability across and within the five imputations (Enders [ 2010 ]; OECD [ 2005 ]; Wu [ 2005 ]).
Within the multilevel regression analyses for each country, we specified the student level as level 1 and the school level as level 2. Since PISA 2003 applied a random sampling procedure at the student and the school level, we decided to control for the clustering of data at these two levels (OECD [ 2005 ]). In addition to this two-level procedure, we regarded the 41 countries as multiple groups (fixed effects). This decision was based on our assumption that the countries selected in PISA 2003 did not necessarily represent a sample of a larger population (Martin et al. [ 2012 ]). Moreover, we did not regard the effects of countries as interchangeable, because, given the specific characteristics of education and instruction within countries; we argue that the effects of competences in mathematics and science on problem solving have their own distinct interpretation in each country (Snijders and Bosker [ 2012 ]). The resulting models were compared by taking into account the Akaike’s information criteria ( AIC ), Bayesian information criteria ( BIC ), and the sample-size adjusted BIC . Also, a likelihood ratio test of the log-Likelihood values ( LogL ) was applied (Hox [ 2010 ]).
To test the Moderation-Hypothesis, we first specified a two-level regression model with problem solving scores as outcomes at the student level (level 1), which allowed variance in achievement scores across schools (level 2). In this model, math and science scores predicted problem solving scores at the student level. To account for differences in the probabilities of being selected as a student within the 41 countries and to adjust the standard errors of regression parameters, we used the robust maximum likelihood (MLR) estimator and students’ final weights (see also Brunner et al. [ 2013 ]; OECD [ 2005 ]). All analyses were conducted in Mplus 6.0 by using the TYPE = IMPUTATION option (Muthén and Muthén [ 2010 ]). As Hox ([ 2010 ]) suggested, using multilevel regression models without taking into account the clustering of data in schools often leads to biased estimates, since achievement variables often have substantial variance at the school level. Consequently, we allowed for level-2-variance within the scores.
After having established whether success in math and science education contributes to the development in problem solving competence across the 41 countries, we then tested whether cross-country differences in the unstandardized regression coefficients were statistically significant by using a multi-group regression model, in which the coefficients were constrained to be equal across countries. We compared this model with the freely estimated model.
Finally, we conceptualized the correlation between math and science scores as an indicator of the level of coherence in math and science education in a country. In relation to the Confounding-Hypothesis, we tested country-specific characteristics for their potentially confounding effects on the relation between math-science coherence and problem solving competence. Following the recommendations proposed by (MacKinnon et al. [ 2000 ]), the confounding analysis was conducted in two steps: (1) we estimated two regression equations. In the first equation, problem solving scores across the 41 countries were regressed on math-science coherence. In the second equation, the respective country characteristics were added as further predictors; (2) the difference between the regression coefficients for math-science coherence obtained in either equation represented the magnitude of a potential confounder effect.
Lastly, we tested the Capability-Utilisation Hypothesis by investigating the bivariate correlations among the CUU Indices and math-science coherence.
Regressing problem solving on math and science performance
To test the Moderation-Hypothesis, we specified regression models with students’ problem-solving score as the outcome and math and science scores as predictors for each of the 41 countries. Due to the clustering of data in schools, these models allowed for between-level variance. Intraclass correlations (ICC-1) for math, science, and problem solving performance ranged between .03 and .61 for the school level ( M = .33, SD = .16).
We specified multilevel regression models for each country separately. These results are reported in Table 2 . The regression coefficients for math on problem solving ranged from .53 to .82 with an average of M( β Math ) = .67 ( SD = .06). The average contribution of science towards problem solving was M( β Science ) = .16 ( SD = .09, Min = -.06, Max = .30). The combination of the distributions of both parameters resulted in substantial differences in the variance explanations of the problem solving scores across the 41 countries ( M[R 2 ] = .65, SD = .15, Min = .27, Max = .86). To test whether these differences were statistically significant, we constrained the regression coefficients of math and science competence within the multi-group regression model to be equal across the 41 countries. Compared to the freely estimated model ( LogL = -4,561,273.3, df = 492, AIC = 9,123,530.5, BIC = 9,128,410.7), the restricted model was empirically not preferred LogL = -4,564,877.9, df = 412, AIC = 9,130,579.8, BIC = 9,134,917.6; Δχ 2 [80] = 7,209.2, p < .001. These findings lend evidence for the Moderation-Hypothesis.
From a slightly different perspective, the country-specific amount of variance in problem solving scores that is explained by the variation in math and science performance scores ( R 2 ) is strongly associated with the country’s problem solving score ( r = .77, p < .001), which suggests that the contribution of science and math competence to the acquisition of problem solving competence was significantly lower in low-performing countries.
As shown in Table 2 , the regression weights of math and science were significant for all but two countries. Across countries the regression weight for math tended to be higher than the regression weight for science when predicting problem solving competence. This finding indicates a stronger overlap between students’ competences in mathematics and problem solving on the one hand and similarities between the assessments in both domains on the other hand.
Validating the concept of math-science coherence
In order to validate the concept of math-science coherence, which is operationalised as the correlation between math and science scores [ r (M,S)], we explored its relation to problem solving and country characteristics.
Regarding the regression outcomes shown in Table 2 , it is apparent that math-science coherence varied considerably across countries, ranging from .39 to .88 with an average of M(r) = .70 ( SD = .13). Interestingly, countries’ level of coherence in math-science education was substantially related to their problem solving scores ( r = .76, p < .001). An inspection of Figure 1 reveals that this effect was mainly due to countries that both achieve low problem solving scores and show relatively low levels of math-science coherence (see bottom left quadrant in Figure 1 ), whilst amongst the remaining countries the correlational link between math-science coherence and problem solving score was almost zero ( r = -.08, p = .71) b . This pattern extends the moderation perspective on the presumed dependency of problem solving competence from math and science competences.
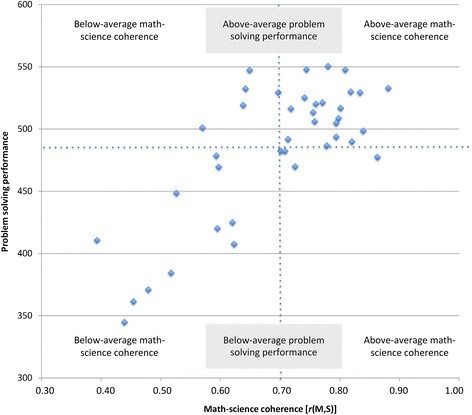
The relation between math-science coherence and problem solving performance across the 41 countries.
As a result of the moderator analysis, we know that countries not only differ in regard to their average problem-solving scores and level of coherence between math and science, countries also differ in the strengths with which math-science coherence predicts problem solving scores. To better understand the conceptual nature of the link between math-science coherence and problem solving, we now attempt to adjust this relationship for potential confounding effects that country-specific characteristics might have. To this end, we employed linear regression and path analysis with students’ problem-solving scores as outcomes, math-science coherence (i.e., r [M,S]) as predictor, and country characteristics as potential confounders.
To establish whether any of the country characteristics had a confounding effect on the link between math-science coherence and problem solving competence, two criteria had to be met: (1) a reduction of the direct effect of math-science coherence on problem solving scores, and (2) testing the difference between the direct effect within the baseline Model M0 and the effect with the confounding Model M1 (Table 3 ).
Regarding the society-related factors, both the countries’ HDI and their national intelligence were confounders with a positive effect. Furthermore, the countries’ integration of the science curriculum was also positively related to the problem solving performance. Finally, the degree of schools’ autonomy towards educational resources and the implementation of curricula and the frequency of assessments were school-related confounders, the former with a positive effect whilst the latter represents a negative confounder. The direct effect of math-science coherence to problem solving decreased and thus indicated that confounding was present (MacKinnon et al. [ 2000 ]).
These findings provide evidence on the Confounding-Hypothesis and support our expectations on the relation between math-science coherence, problem solving, and country characteristics. We regard these results as evidence for the validity of the math-science coherence measure.
Relating math-science coherence to the capability under-utilisation indices
To advance our understanding of the link between math-science coherence and problem solving scores, we tested the Capability-Utilisation Hypothesis. To this end, we explored the relationship between math-science coherence and the CUU Indices for math and science, respectively. For math competence the average Capability Under-Utilisation Index was rather neutral with M CUUI-Math = -0.001 ( SD = 0.02). This suggests that, on average, all countries sufficiently utilise their students’ math capabilities in facilitating the development of problem solving competence (i.e., transfer). It also suggests that math education across participating countries tends to sufficiently utilise generic problem-solving skills (Figure 2 ). The picture is different for science education. Here, the Capability Under-Utilisation Indices and their variation across the participating countries ( M CUUI-Science = -0.01, SD = 0.04) suggest that in a range of countries knowledge and skills taught in science education tend to be under-utilised in the facilitation of the acquisition of problem solving competence (Figure 3 ).
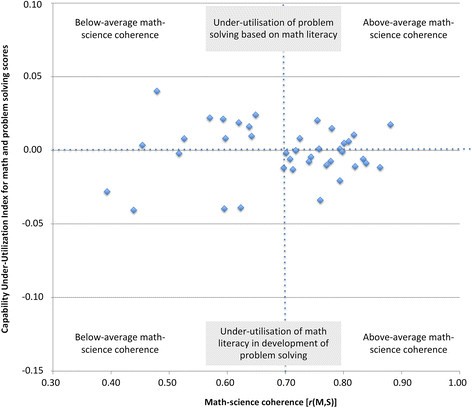
The relation between math-science coherence and the capability under-utilisation index for math and problem solving scores across the 41 countries.
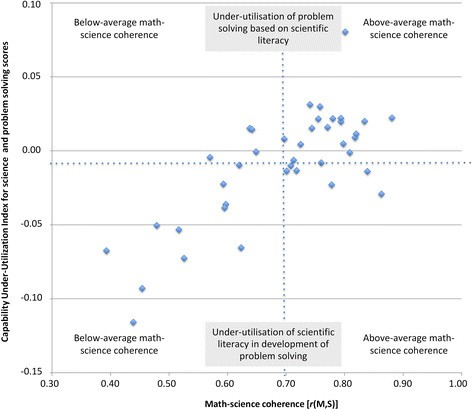
The relation between math-science coherence and the capability under-utilisation index for science and problem solving scores across the 41 countries.
For math competence, the relative difference to problem solving was not related to math-science coherence ( r = .02, p = .89; Figure 2 ). In contrast, the Capability Under-Utilisation Index for science showed a strong positive correlation with math-science coherence ( r = .76, p < .001; Figure 3 ), indicating that low levels of coherence between math and science education were associated with a less effective transfer of domain-specific knowledge and skills to problem solving.
The present study was aimed at investigating the differences in the contribution of math and science competence to problem solving competence across the 41 countries that participated in the PISA 2003 study (Moderation-Hypothesis). To this end, we proposed the concept of math-science coherence and explored its relationship to problem solving competence and how this relationship is confounded by country characteristics (Confounding-Hypothesis). To further extend our understanding of the link between math-science coherence and problem solving, we introduced the concept of capability-utilisation. Testing the Capability-Utilisation Hypothesis enabled us to identify what may contribute to varying levels of math-science coherence and ultimately the development of problem solving competence.
The contribution of math and science competence across countries
Regarding the prediction of problem solving competence, we found that in most countries, math and science competence significantly contributed to students’ performance in analytical problem solving. This finding was expected based on the conceptualizations of mathematical and scientific literacy within the PISA framework referring to shared cognitive processes such as information processing and reasoning (Kind [ 2013 ]; OECD [ 2005 ]), which are regarded as components of problem solving (Bybee [ 2004 ]; Klahr and Dunbar [ 1988 ]; Mayer [ 2010 ]).
It is noteworthy that, for some of the below-average performing countries, science competence did not significantly contribute to the prediction of problem solving competence. It can be speculated that education in these countries is more geared towards math education and modelling processes in mathematical scenarios, whilst the aspect of problem solving in science is less emphasised (Janssen and Geiser [ 2012 ]). The results of multilevel regression analyses supported this interpretation by showing that math competence was a stronger predictor of problem solving competence. On the one hand, this finding could be due to the design of the PISA tests (Adams [ 2005 ]), since math and problem solving items are designed in such a way that modelling real-life problems is required, whereas science items are mostly domain-specific and linked to science knowledge (Nentwig et al. [ 2009 ]; OECD [ 2004 ]). Moreover, one may argue that math and problem solving items allow students to employ different solution strategies, whereas science items offer fewer degrees of freedom for test takers (Nentwig et al. [ 2009 ]). In particular, the shared format of items in math, science, and problem solving may explain an overlap between their cognitive demands. For instance, most of the items were designed in such a way that students had to extract and identify relevant information from given tables or figures in order to solve specific problems. Hence, these items were static and did not require knowledge generation by interaction or exploration but rather the use of given information in problem situations (Wood et al. [ 2009 ]). In contrast to the domain-specific items in math and science, problem solving items did not require the use of prior knowledge in math and science (OECD [ 2004 ]). In addition, some of the math and science items involved cognitive operations that were specific to these domains. For instance, students had to solve a number of math items by applying arithmetic and combinatorial operations (OECD [ 2005 ]). Finally, since items referred to contextual stimuli, which were presented in textual formats, reading ability can be regarded as another, shared demand of solving the items. Furthermore, Rindermann ([ 2007 ]) clearly showed that the shared demands of the achievement tests in large-scale assessments such as PISA were strongly related to students’ general reasoning skills. This finding is in line with the strong relations between math, science, and problem solving competence, found in our study. The interpretation of the overlap between the three competences can also be interpreted from a conceptual point of view. In light of the competence frameworks in PISA, we argue that there are a number of skills that can be found in math, science, and problem solving: information retrieval and processing, knowledge application, and evaluation of results (Griffin et al. [ 2012 ]; OECD [ 2004 ], [ 2005 ]). These skills point out to the importance of reasoning in the three domains (Rindermann [ 2007 ]). Thus, the empirical overlap between math and problem solving can be explained by shared processes of, what Mayer ([ 2010 ]) refers to as, informal reasoning. On the other hand, the stronger effect of math competence could be an effect of the quality of math education. Hiebert et al. ([ 1996 ]) and Kuo et al. ([ 2013 ]) suggested that math education is more based on problem solving skills than other subjects in school (e.g., Polya [ 1945 ]). Science lessons, in contrast, are often not necessarily problem-based, despite the fact that they often start with a set problem. Risch ([ 2010 ]) showed in a cross-national review that science learning was more related to contents and contexts rather than to generic problem-solving skills. These tendencies might lead to a weaker contribution of science education to the development of problem solving competence (Abd-El-Khalick et al. [ 2004 ]).
In sum, we found support on the Moderation-Hypothesis, which assumed systematic differences in the contribution of math and science competence to problem solving competence across the 41 PISA 2003 countries.
The relation to problem solving
In our study, we introduced the concept of math-science coherence, which reflects the degree to which math and science education are harmonized. Since mathematical and scientific literacy show a conceptual overlap, which refers to a set of cognitive processes that are linked to reasoning and information processing (Fensham and Bellocchi [ 2013 ]; Mayer [ 2010 ]), a significant relation between math-science coherence and problem solving was expected. In our analyses, we found a significant and positive effect of math-science coherence on performance scores in problem solving. In this finding we see evidence for the validity of this newly introduced concept of math-science coherence and its focus on the synergistic effect of math and science education on problem solving. The results further suggest that higher levels of coordination between math and science education has beneficial effects on the development of cross-curricular problem-solving competence (as measured within the PISA framework).
Confounding effects of country characteristics
As another step of validating the concept of math-science coherence, we investigated whether country-specific characteristics that are linked to society-, curriculum-, and school-related factors confounded its relation to problem solving. Our results showed that national intelligence, the Human Development Index, the integration of the science curriculum, and schools’ autonomy were positively linked to math-science coherence and problem solving, whilst a schools’ frequency of assessment had a negative confounding effect.
The findings regarding the positive confounders are in line with and also extend a number of studies on cross-country differences in education (e.g., Blömeke et al. [ 2011 ]; Dronkers et al. [ 2014 ]; Janssen and Geiser [ 2012 ]; Risch [ 2010 ]). Ross and Hogaboam-Gray ([ 1998 ]), for instance, found that students benefit from an integrated curriculum, particularly in terms of motivation and the development of their abilities. In the context of our confounder analysis, the integration of the science curriculum as well as the autonomy to allocate resources is expected to positively affect math-science coherence. At the same time, an integrated science curriculum with a coordinated allocation of resources may promote inquiry-based experiments in science courses, which is assumed to be beneficial for the development of problem solving within and across domains. Teaching science as an integrated subject is often regarded a challenge for teachers, particularly when developing conceptual structures in science lessons (Lang and Olson, [ 2000 ]), leading to teaching practices in which cross-curricular competence is rarely taken into account (Mansour [ 2013 ]; van Merriënboer [ 2013 ]).
The negative confounding effect of assessment frequency suggests that high frequencies of assessment, as it presumably applies to both math and science subjects, contribute positively to math-science coherence. However, the intended or unintended engagement in educational activities associated with assessment preparation tends not to be conducive to effectively developing domain-general problem solving competence (see also Neumann et al. [ 2012 ]).
The positive confounder effect of HDI is not surprising as HDI reflects a country’s capability to distribute resources and to enable certain levels of autonomy (Reich et al. [ 2013 ]). To find national intelligence as a positive confounder is also to be expected as the basis for its estimation are often students’ educational outcome measures (e.g., Rindermann [ 2008 ]) and, as discussed earlier, academic achievement measures share the involvement of a set of cognitive processes (Baumert et al. [ 2009 ]; OECD [ 2004 ]).
In summary, the synergistic effect of a coherent math and science education on the development of problem solving competence is substantially linked to characteristics of a country’s educational system with respect to curricula and school organization in the context of its socio-economic capabilities. Math-science coherence, however, also is linked to the extent to which math or science education is able to utilise students’ educational capabilities.
Math-science coherence and capability-utilisation
So far, discrepancies between students’ performance in math and problem solving or science and problem solving have been discussed as indicators of students’ capability utilisation in math or science (Leutner et al. [ 2012 ]; OECD [ 2004 ]). We have extended this perspective by introducing Capability Under-Utilisation Indices for math and science to investigate the effectiveness with which knowledge and skills acquired in the context of math or science education are transferred into cross-curricular problem-solving competence. The Capability Under-Utilisation Indices for math and science reflect a potential quantitative imbalance between math, science, and problem solving performance within a country, whilst the also introduced concept of math-science coherence reflects a potential qualitative imbalance between math and science education.
The results of our analyses suggest that an under-utilisation of problem solving capabilities in the acquisition of science literacy is linked to lower levels of math-science coherence, which ultimately leads to lower scores in problem solving competence. This interpretation finds resonance in Ross and Hogaboam-Gray’s ([ 1998 ]) argumentation for integrating math and science education and supports the attempts of math and science educators to incorporate higher-order thinking skills in teaching STEM subjects (e.g., Gallagher et al. [ 2012 ]; Zohar [ 2013 ]).
In contrast, the CUU Index for math was not related to math-science coherence in our analyses. This might be due to the conceptualizations and assessments of mathematical literacy and problem solving competence. Both constructs share cognitive processes of reasoning and information processing, resulting in quite similar items. Consequently, the transfer from math-related knowledge and skills to cross-curricular problems does not necessarily depend on how math and science education are harmonised, since the conceptual and operational discrepancy between math and problem solving is rather small.
Math and science education do matter to the development of students’ problem-solving skills. This argumentation is based on the assumption that the PISA assessments in math, science, and problem solving are able to measure students’ competence as outcomes, which are directly linked to their education (Bulle [ 2011 ]; Kind [ 2013 ]). In contrast to math and science competence, problem solving competence is not explicitly taught as a subject. Problem solving competence requires the utilisation of knowledge and reasoning skills acquired in specific domains (Pellegrino and Hilton [ 2012 ]). In agreement with Kuhn ([ 2009 ]), we point out that this transfer does not happen automatically but needs to be actively facilitated. In fact, Mayer and Wittrock ([ 2006 ]) stressed that the development of transferable skills such as problem solving competence needs to be fostered within specific domains rather than taught in dedicated, distinct courses. Moreover, they suggested that students should develop a “repertoire of cognitive and metacognitive strategies that can be applied in specific problem-solving situations” (p. 299). Beyond this domain-specific teaching principle, research also proposes to train the transfer of problem solving competence in domains that are closely related (e.g., math and science; Pellegrino and Hilton [ 2012 ]). In light of the effects of aligned curricula (as represented by the concept of math-science coherence), we argue that educational efforts to increase students’ problem solving competence may focus on a coordinated improvement of math and science literacy and fostering problem solving competence within math and science. The emphasis is on coordinated, as the results of our analyses indicated that the coherence between math and science education, as a qualitative characteristic of a country’s educational system, is a strong predictor of problem solving competence. This harmonisation of math and science education may be achieved by better enabling the utilisation of capabilities, especially in science education. Sufficiently high levels of math-science coherence could facilitate the emergence of educational synergisms, which positively affect the development of problem solving competence. In other words, we argue for quantitative changes (i.e., improve science attainment) in order to achieve qualitative changes (i.e., higher levels of curriculum coherence), which are expected to create effective transitions of subject-specific knowledge and skills into subject-unspecific competences to solve real-life problems (Pellegrino and Hilton [ 2012 ]; van Merriënboer [ 2013 ]).
Finally, we encourage research that is concerned with the validation of the proposed indices for different forms of problem solving. In particular, we suggest studying the facilities of the capability-under-utilisation indices for analytical and dynamic problem solving, as assessed in the PISA 2012 study (OECD [ 2014 ]). Due to the different cognitive demands in analytical and dynamic problems (e.g., using existing knowledge vs. generating knowledge; OECD [ 2014 ]), we suspect differences in capability utilisation in math and science. This research could provide further insights into the role of 21 st century skills as educational goals.
a The differences between students’ achievement in mathematics and problem solving, and science and problem solving have to be interpreted relative to the OECD average, since the achievement scales were scaled with a mean of 500 and a standard deviation of 100 for the OECD countries (OECD [ 2004 ], p. 55). Although alternative indices such as country residuals may also be used in cross-country comparisons (e.g., Olsen [ 2005 ]), we decided to use CUU indices, as they reflect the actual differences in achievement scores.
b In addition, we checked whether this result was due to the restricted variances in low-performing countries and found that neither ceiling nor floor effects in the problem solving scores existed. The problem solving scale differentiated sufficiently reliably in the regions below and above the OECD mean of 500.
Abd-El-Khalick F, Boujaoude S, Duschl R, Lederman NG, Mamlok-Naaman R, Hofstein A, Niaz M, Treagust D, Tuan H-L: Inquiry in science education: International perspectives. Science Education 2004, 88: 397–419. doi:10.1002/sce.10118 10.1002/sce.10118
Article Google Scholar
Adams RJ: Reliability as a measurement design effect. Studies in Educational Evaluation 2005, 31: 162–172. doi:10.1016/j.stueduc.2005.05.008 10.1016/j.stueduc.2005.05.008
Arts J, Gijselaers W, Boshuizen H: Understanding managerial problem solving, knowledge use and information processing: Investigating stages from school to the workplace. Contemporary Educational Psychology 2006, 31: 387–410. doi:10.1016/j.cedpsych.2006.05.005 10.1016/j.cedpsych.2006.05.005
Bassok M, Holyoak K: Pragmatic knowledge and conceptual structure: determinants of transfer between quantitative domains. In Transfer on trial: intelligence, cognition, and instruction . Edited by: Detterman DK, Sternberg RJ. Ablex, Norwood, NJ; 1993:68–98.
Google Scholar
Baumert J, Lüdtke O, Trautwein U, Brunner M: Large-scale student assessment studies measure the results of processes of knowledge acquisition: evidence in support of the distinction between intelligence and student achievement. Educational Research Review 2009, 4: 165–176. doi:10.1016/j.edurev.2009.04.002 10.1016/j.edurev.2009.04.002
Beckmann JF: Zur Validierung des Konstrukts des intellektuellen Veränderungspotentials [On the validation of the construct of intellectual change potential] . logos, Berlin; 2001.
Blömeke S, Houang R, Suhl U: TEDS-M: diagnosing teacher knowledge by applying multidimensional item response theory and multiple-group models. IERI Monograph Series: Issues and Methodologies in Large-Scale Assessments 2011, 4: 109–129.
Bouhilila D, Sellaouti F: Multiple imputation using chained equations for missing data in TIMSS: a case study. Large-scale Assessments in Education 2013, 1: 4.
Brunner M, Gogol K, Sonnleitner P, Keller U, Krauss S, Preckel F: Gender differences in the mean level, variability, and profile shape of student achievement: Results from 41 countries. Intelligence 2013, 41: 378–395. doi:10.1016/j.intell.2013.05.009 10.1016/j.intell.2013.05.009
Bulle N: Comparing OECD educational models through the prism of PISA. Comparative Education 2011, 47: 503–521. doi: 10.1080/03050068.2011.555117 10.1080/03050068.2011.555117
Bybee R: Scientific Inquiry and Science Teaching. In Scientific Inquiry and the Nature of Science . Edited by: Flick L, Lederman N. Springer & Kluwers, New York, NY; 2004:1–14. doi:10.1007/978–1-4020–5814–1_1
Chiu M, Chow B: Classroom discipline across forty-One countries: school, economic, and cultural differences. Journal of Cross-Cultural Psychology 2011, 42: 516–533. doi:10.1177/0022022110381115 10.1177/0022022110381115
Drake S, Reid J: Integrated curriculum: Increasing relevance while maintaining accountability. Research into Practice 2010, 28: 1–4.
Dronkers J, Levels M, de Heus M: Migrant pupils’ scientific performance: the influence of educational system features of origin and destination countries. Large-scale Assessments in Education 2014, 2: 3.
Enders C: Applied Missing Data Analysis . The Guilford Press, New York, NY; 2010.
Ewell P: A world of assessment: OECD’s AHELO initiative. Change: The Magazine of Higher Learning 2012, 44: 35–42. doi:10.1080/00091383.2012.706515 10.1080/00091383.2012.706515
Fensham P, Bellocchi A: Higher order thinking in chemistry curriculum and its assessment. Thinking Skills and Creativity 2013, 10: 250–264. doi:10.1016/j.tsc.2013.06.003 10.1016/j.tsc.2013.06.003
Gallagher C, Hipkins R, Zohar A: Positioning thinking within national curriculum and assessment systems: perspectives from Israel, New Zealand and Northern Ireland. Thinking Skills and Creativity 2012, 7: 134–143. doi:10.1016/j.tsc.2012.04.005 10.1016/j.tsc.2012.04.005
Greiff S, Holt D, Funke J: Perspectives on problem solving in educational assessment: analytical, interactive, and collaborative problem solving. The Journal of Problem Solving 2013, 5: 71–91. doi:10.7771/1932–6246.1153 10.7771/1932-6246.1153
Griffin P, Care E, McGaw B: The changing role of education and schools. In Assessment and Teaching of 21st Century Skills . Edited by: Griffin P, McGaw B, Care E. Springer, Dordrecht; 2012:1–15. 10.1007/978-94-007-2324-5_1
Chapter Google Scholar
Hickendorff M: The language factor in elementary mathematics assessments: Computational skills and applied problem solving in a multidimensional IRT framework. Applied Measurement in Education 2013, 26: 253–278. doi:10.1080/08957347.2013.824451 10.1080/08957347.2013.824451
Hiebert J, Carpenter T, Fennema E, Fuson K, Human P, Murray H, Olivier A, Wearne D: Problem Solving as a Basis for Reform in Curriculum and Instruction: The Case of Mathematics. Educational Researcher 1996, 25: 12–21. doi:10.3102/0013189X025004012 10.3102/0013189X025004012
Holliday W, Holliday B: Why using international comparative math and science achievement data from TIMSS is not helpful. The Educational Forum 2003, 67: 250–257. 10.1080/00131720309335038
Hox J: Multilevel Analysis . 2nd edition. Routlegde, New York, NY; 2010.
Janssen A, Geiser C: Cross-cultural differences in spatial abilities and solution strategies: An investigation in Cambodia and Germany. Journal of Cross-Cultural Psychology 2012, 43: 533–557. doi:10.1177/0022022111399646 10.1177/0022022111399646
Jonassen D: Learning to solve problems . Routledge, New York, NY; 2011.
Kind P: Establishing Assessment Scales Using a Novel Disciplinary Rationale for Scientific Reasoning. Journal of Research in Science Teaching 2013, 50: 530–560. doi:10.1002/tea.21086 10.1002/tea.21086
Klahr D, Dunbar K: Dual Space Search during Scientific Reasoning. Cognitive Science 1988, 12: 1–48. doi:10.1207/s15516709cog1201_1 10.1207/s15516709cog1201_1
Koeppen K, Hartig J, Klieme E, Leutner D: Current issues in competence modeling and assessment. Journal of Psychology 2008, 216: 61–73. doi:10.1027/0044–3409.216.2.61
Kuhn D: Do students need to be taught how to reason? Educational Research Review 2009, 4: 1–6. doi:10.1016/j.edurev.2008.11.001 10.1016/j.edurev.2008.11.001
Kuo E, Hull M, Gupta A, Elby A: How Students Blend Conceptual and Formal Mathematical Reasoning in Solving Physics Problems. Science Education 2013, 97: 32–57. doi:10.1002/sce.21043 10.1002/sce.21043
Lang M, Olson J: Integrated science teaching as a challenge for teachers to develop new conceptual structures. Research in Science Education 2000, 30: 213–224. doi:10.1007/BF02461629 10.1007/BF02461629
Leutner D, Fleischer J, Wirth J, Greiff S, Funke J: Analytische und dynamische Problemlösekompetenz im Lichte internationaler Schulleistungsstudien [Analytical and dynamic problem-solvng competence in international large-scale studies]. Psychologische Rundschau 2012, 63: 34–42. doi:10.1026/0033–3042/a000108 10.1026/0033-3042/a000108
Lynn R, Meisenberg G: National IQs calculated and validated for 108 nations. Intelligence 2010, 38: 353–360. doi:10.1016/j.intell.2010.04.007 10.1016/j.intell.2010.04.007
MacKinnon D, Krull J, Lockwood C: Equivalence of the mediation, confounding, and suppression effect. Prevention Science 2000, 1: 173–181. doi:10.1023/A:1026595011371 10.1023/A:1026595011371
Mansour N: Consistencies and inconsistencies between science teachers’ beliefs and practices. International Journal of Science Education 2013, 35: 1230–1275. doi:10.1080/09500693.2012.743196 10.1080/09500693.2012.743196
Martin M, Mullis I, Gonzalez E, Chrostowski S: TIMSS 2003 International Science Report . IEA, Chestnut Hill, MA; 2004.
Martin AJ, Liem GAD, Mok MMC, Xu J: Problem solving and immigrant student mathematics and science achievement: Multination findings from the Programme for International Student Assessment (PISA). Journal of Educational Psychology 2012, 104: 1054–1073. doi:10.1037/a0029152 10.1037/a0029152
Mayer R: Problem solving and reasoning. In International Encyclopedia of Education . 3rd edition. Edited by: Peterson P, Baker E, McGraw B. Elsevier, Oxford; 2010:273–278. doi:10.1016/B978–0-08–044894–7.00487–5 10.1016/B978-0-08-044894-7.00487-5
Mayer R, Wittrock MC: Problem solving. In Handbook of Educational Psychology . 2nd edition. Edited by: Alexander PA, Winne PH. Lawrence Erlbaum, New Jersey; 2006:287–303.
Muthén B, Muthén L: Mplus 6 . Muthén & Muthén, Los Angeles, CA; 2010.
Successful K-12 STEM Education . National Academies Press, Washington, DC; 2011.
Nentwig P, Rönnebeck S, Schöps K, Rumann S, Carstensen C: Performance and levels of contextualization in a selection of OECD countries in PISA 2006. Journal of Research in Science Teaching 2009, 8: 897–908. doi:10.1002/tea.20338 10.1002/tea.20338
Neumann K, Kauertz A, Fischer H: Quality of Instruction in Science Education. In Second International Handbook of Science Education (Part One . Edited by: Fraser B, Tobin K, McRobbie C. Springer, Dordrecht; 2012:247–258.
The PISA 2003 Assessment Frameworks . OECD, Paris; 2003.
Problem solving for tomorrow’s world . OECD, Paris; 2004.
PISA 2003 Technical Report . OECD, Paris; 2005.
PISA 2012 Results : Creative Problem Solving – Students’ Skills in Tackling Real-Life Problems (Vol. V) . OECD, Paris; 2014.
Olsen RV: An exploration of cluster structure in scientific literacy in PISA: Evidence for a Nordic dimension? NorDiNa. ᅟ 2005, 1 (1):81–94.
Oser F, Baeriswyl F: Choreographies of Teaching: Bridging Instruction to Learning. In Handbook of Research on Teaching . 4th edition. Edited by: Richardson V. American Educational Research Association, Washington, DC; 2001:1031–1065.
Pellegrino JW, Hilton ML: Education for Life and Work – Developing Transferable Knowledge and Skills in the 21st Century . The National Academies Press, Washington, DC; 2012.
Polya G: How to solve it: a new aspect of mathematical method . Princeton University Press, Princeton, NJ; 1945.
Reich J, Hein S, Krivulskaya S, Hart L, Gumkowski N, Grigorenko E: Associations between household responsibilities and academic competencies in the context of education accessibility in Zambia. Learning and Individual Differences 2013, 27: 250–257. doi:10.1016/j.lindif.2013.02.005 10.1016/j.lindif.2013.02.005
Rindermann H: The g-factor of international cognitive ability comparisons: The homogeneity of results in PISA, TIMSS, PIRLS and IQ-tests across nations. European Journal of Personality 2007, 21: 661–706. doi:10.1002/per.634
Rindermann H: Relevance of education and intelligence at the national level for the economic welfare of people. Intelligence 2008, 36: 127–142. doi:10.1016/j.intell.2007.02.002 10.1016/j.intell.2007.02.002
Risch B: Teaching Chemistry around the World . Waxmann, Münster; 2010.
Ross J, Hogaboam-Gray A: Integrating mathematics, science, and technology: effects on students. International Journal of Science Education 1998, 20: 1119–1135. doi:10.1080/0950069980200908 10.1080/0950069980200908
Snijders TAB, Bosker RJ: Multilevel Analysis: An Introduction to Basic and Advanced Multilevel Modeling . 2nd edition. Sage Publications, London; 2012.
Human Development Report 2005 . UNDP, New York, NY; 2005.
Van Merriënboer J: Perspectives on problem solving and instruction. Computers & Education 2013, 64: 153–160. doi:10.1016/j.compedu.2012.11.025 10.1016/j.compedu.2012.11.025
Wood RE, Beckmann JF, Birney D: Simulations, learning and real world capabilities. Education Training 2009, 51 (5/6):491–510. doi:10.1108/00400910910987273 10.1108/00400910910987273
Wu M: The role of plausible values in large-scale surveys. Studies in Educational Evaluation 2005, 31: 114–128. doi:10.1016/j.stueduc.2005.05.005 10.1016/j.stueduc.2005.05.005
Wu M, Adams R: Modelling Mathematics Problem Solving Item Responses using a Multidimensional IRT Model. Mathematics Education Research Journal 2006, 18: 93–113. doi:10.1007/BF03217438 10.1007/BF03217438
Wüstenberg S, Greiff S, Funke J: Complex problem solving: More than reasoning? Intelligence 2012, 40: 1–14. doi:10.1016/j.intell.2011.11.003 10.1016/j.intell.2011.11.003
Zohar A: Scaling up higher order thinking in science classrooms: the challenge of bridging the gap between theory, policy and practice. Thinking Skills and Creativity 2013, 10: 168–172. doi:10.1016/j.tsc.2013.08.001 10.1016/j.tsc.2013.08.001
Download references
Author information
Authors and affiliations.
Centre for Educational Measurement, University of Oslo (CEMO), Oslo, Norway
Ronny Scherer
Faculty of Educational Sciences, University of Oslo, Oslo, Norway
School of Education, Durham University, Durham, UK
Jens F Beckmann
You can also search for this author in PubMed Google Scholar
Corresponding author
Correspondence to Ronny Scherer .
Additional information
Competing interests.
The authors declare that they have no competing interests.
Authors’ contributions
RS carried out the analyses, participated in the development of the rationale, and drafted the manuscript. JFB carried out some additional analyses, participated in the development of the rationale, and drafted the manuscript. Both authors read and approved the final manuscript.
Authors’ original submitted files for images
Below are the links to the authors’ original submitted files for images.
Authors’ original file for figure 1
Authors’ original file for figure 2, authors’ original file for figure 3, rights and permissions.
Open Access This article is distributed under the terms of the Creative Commons Attribution 4.0 International License ( https://creativecommons.org/licenses/by/4.0 ), which permits use, duplication, adaptation, distribution, and reproduction in any medium or format, as long as you give appropriate credit to the original author(s) and the source, provide a link to the Creative Commons license, and indicate if changes were made.
Reprints and permissions
About this article
Cite this article.
Scherer, R., Beckmann, J.F. The acquisition of problem solving competence: evidence from 41 countries that math and science education matters. Large-scale Assess Educ 2 , 10 (2014). https://doi.org/10.1186/s40536-014-0010-7
Download citation
Received : 08 June 2014
Accepted : 25 November 2014
Published : 10 December 2014
DOI : https://doi.org/10.1186/s40536-014-0010-7
Share this article
Anyone you share the following link with will be able to read this content:
Sorry, a shareable link is not currently available for this article.
Provided by the Springer Nature SharedIt content-sharing initiative
- Capability under-utilisation
- Math-science coherence
- Math education
- Problem solving competence
- Science education
5 Steps to Teaching Students a Problem-Solving Routine
- Share article
By Jeff Heyck-Williams, the director of curriculum and instruction for Two Rivers Public Charter School
When I visited a 5th grade class recently, the students were tackling the following problem:
If there are nine people in a room and every person shakes hands exactly once with each of the other people, how many handshakes will there be? How can you prove your answer is correct using a model or numerical explanation?
There were students on the rug modeling people with Unifix cubes. There were kids at one table vigorously shaking each other’s hand. There were kids at another table writing out a diagram with numbers. At yet another table, students were working on creating a numeric expression. What was common across this class was that all of the students were productively grappling around the problem.
On a different day, I was out at recess with a group of kindergartners who got into an argument over a vigorous game of tag. Several kids were arguing about who should be “it.” Many of them insisted that they hadn’t been tagged. They all agreed that they had a problem. With the assistance of the teacher, they walked through a process of identifying what they knew about the problem and how best to solve it. They grappled with this very real problem to come to a solution that all could agree upon.
Then just last week, I had the pleasure of watching a culminating showcase of learning for our 8th graders. They presented to their families about their project exploring the role that genetics plays in our society. Tackling the problem of how we should or should not regulate gene research and editing in the human population, students explored both the history and scientific concerns about genetics and the ethics of gene editing. Each student developed arguments about how we as a country should proceed in the burgeoning field of human genetics, which they took to Capitol Hill to share with legislators. Through the process, students read complex text to build their knowledge, identified the underlying issues and questions, and developed unique solutions to this very real problem.
Problem-solving is at the heart of each of these scenarios and is an essential set of skills our students need to develop. They need the abilities to think critically and solve challenging problems without a roadmap to solutions. At Two Rivers Public Charter School in the District of Columbia, we have found that one of the most powerful ways to build these skills in students is through the use of a common set of steps for problem-solving. These steps, when used regularly, become a flexible cognitive routine for students to apply to problems across the curriculum and their lives.
The Problem-Solving Routine
At Two Rivers, we use a fairly simple routine for problem-solving that has five basic steps. The power of this structure is that it becomes a routine that students are able to use regularly across multiple contexts. The first three steps are implemented before problem-solving. Students use one step during problem-solving. Finally, they finish with a reflective step after problem-solving.
Problem Solving from Two Rivers Public Charter School on Vimeo .

Before Problem-Solving: The KWI
The three steps before problem-solving: We call them the K-W-I.
The “K” stands for “know” and requires students to identify what they already know about a problem. The goal in this step of the routine is two-fold. First, the student needs to analyze the problem and identify what is happening within the context of the problem. For example, in the math problem above, students identify that they know there are nine people and each person must shake hands with each other person. Second, the student needs to activate their background knowledge about that context or other similar problems. In the case of the handshake problem, students may recognize that this seems like a situation in which they will need to add or multiply.
The “W” stands for “what” a student needs to find out to solve the problem. At this point in the routine, the student always must identify the core question that is being asked in a problem or task. However, it may also include other questions that help a student access and understand a problem more deeply. For example, in addition to identifying that they need to determine how many handshakes in the math problem, students may also identify that they need to determine how many handshakes each individual person has or how to organize their work to make sure that they count the handshakes correctly.
The “I” stands for “ideas” and refers to ideas that a student brings to the table to solve a problem effectively. In this portion of the routine, students list the strategies that they will use to solve a problem. In the example from the math class, this step involved all of the different ways that students tackled the problem from Unifix cubes to creating mathematical expressions.
This KWI routine before problem-solving sets students up to actively engage in solving problems by ensuring they understand the problem and have some ideas about where to start in solving the problem. Two remaining steps are equally important during and after problem-solving.
During Problem-Solving: The Metacognitive Moment
The step that occurs during problem-solving is a metacognitive moment. We ask students to deliberately pause in their problem-solving and answer the following questions: “Is the path I’m on to solve the problem working?” and “What might I do to either stay on a productive path or readjust my approach to get on a productive path?” At this point in the process, students may hear from other students that have had a breakthrough or they may go back to their KWI to determine if they need to reconsider what they know about the problem. By naming explicitly to students that part of problem-solving is monitoring our thinking and process, we help them become more thoughtful problem-solvers.
After Problem-Solving: Evaluating Solutions
As a final step, after students solve the problem, they evaluate both their solutions and the process that they used to arrive at those solutions. They look back to determine if their solution accurately solved the problem, and when time permits, they also consider if their path to a solution was efficient and how it compares with other students’ solutions.
The power of teaching students to use this routine is that they develop a habit of mind to analyze and tackle problems wherever they find them. This empowers students to be the problem-solvers that we know they can become.
The opinions expressed in Next Gen Learning in Action are strictly those of the author(s) and do not reflect the opinions or endorsement of Editorial Projects in Education, or any of its publications.
Sign Up for The Savvy Principal
- Our Mission
Problem-Solving in Elementary School
Elementary students practice problem-solving and self-questioning techniques to improve reading and social and emotional learning skills.
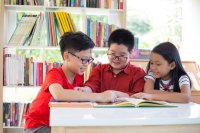
In a school district in New Jersey, beginning in kindergarten each child is seen as a future problem solver with creative ideas that can help the world. Vince Caputo, superintendent of the Metuchen School District, explained that what drew him to the position was “a shared value for whole child education.”
Caputo’s first hire as superintendent was Rick Cohen, who works as both the district’s K–12 director of curriculum and principal of Moss Elementary School . Cohen is committed to integrating social and emotional learning (SEL) into academic curriculum and instruction by linking cognitive processes and guided self-talk.
Cohen’s first focus was kindergarten students. “I recommended Moss teachers teach just one problem-solving process to our 6-year-olds across all academic content areas and challenge students to use the same process for social problem-solving,” he explained.
Reading and Social Problem-Solving
Moss Elementary classrooms use a specific process to develop problem-solving skills focused on tending to social and interpersonal relationships. The process also concentrates on building reading skills—specifically, decoding and comprehension.
Stop, Look, and Think. Students define the problem. As they read, they look at the pictures and text for clues, searching for information and asking, “What is important and what is not?” Social problem-solving aspect: Students look for signs of feelings in others’ faces, postures, and tone of voice.
Gather Information . Next, students explore what feelings they’re having and what feelings others may be having. As they read, they look at the beginning sound of a word and ask, “What else sounds like this?” Social problem-solving aspect: Students reflect on questions such as, “What word or words describe the feeling you see or hear in others? What word describes your feeling? How do you know, and how sure are you?”
Brainstorming . Then students seek different solutions. As they read, they wonder, “Does it sound right? Does it make sense? How else could it sound to make more sense? What other sounds do those letters make?” Social problem-solving aspect: Students reflect on questions such as, “How can you solve the problem or make the situation better? What else can you think of? What else can you try? What other ideas do you have?”
Pick the Best One. Next, students evaluate the solution. While reading, they scan for smaller words they know within larger, more difficult words. They read the difficult words the way they think they sound while asking, “Will it make sense to other people?” Social problem-solving aspect: Students reflect on prompts such as, “Pick the solution that you think will be best to solve the problem. Ask yourself, ‘What will happen if I do this—for me, and for others involved?’”
Go . In the next step, students make a plan and act. They do this by rereading the text. Social problem-solving aspect: Students are asked to try out what they will say and how they will say it. They’re asked to pick a good time to do this, when they’re willing to try it.
Check . Finally, students reflect and revise. After they have read, they ponder what exactly was challenging about what they read and, based on this, decide what to do next. Social problem-solving aspect: Students reflect on questions such as, “How did it work out? Did you solve the problem? How did others feel about what happened? What did you learn? What would you do if the same thing happened again?”
You can watch the Moss Elementary Problem Solvers video and see aspects of this process in action.
The Process of Self-Questioning
Moss Elementary students and other students in the district are also taught structured self-questioning. Cohen notes, “We realized that many of our elementary students would struggle to generalize the same steps and thinking skills they previously used to figure out an unknown word in a text or resolve social conflicts to think through complex inquiries and research projects.” The solution? Teach students how to self-question, knowing they can also apply this effective strategy across contexts. The self-questioning process students use looks like this:
Stop and Think. “What’s the question?”
Gather Information. “How do I gather information? What are different sides of the issue?”
Brainstorm and Choose. “How do I select, organize, and choose the information? What are some ways to solve the problem? What’s the best choice?”
Plan and Try. “What does the plan look like? When and how can it happen? Who needs to be involved?”
Check & Revise. “How can I present the information? What did I do well? How can I improve?”
The Benefits
Since using the problem-solving and self-questioning processes, the students at Moss Elementary have had growth in their scores for the last two years on the fifth-grade English language arts PARCC tests . However, as Cohen shares, “More important than preparing our students for the tests on state standards, there is evidence that we are also preparing them for the tests of life.”
K-12 Resources By Teachers, For Teachers Provided by the K-12 Teachers Alliance
- Teaching Strategies
- Classroom Activities
- Classroom Management
- Technology in the Classroom
- Professional Development
- Lesson Plans
- Writing Prompts
- Graduate Programs
Approaches to Successful Cross-Curricular Integration
Colleen sullivan.
- November 5, 2019

What is Cross-Curricular Instruction?
Cross-curricular instruction is a great way for teachers to incorporate different disciplines in education into one. It can also be referred to as Interdisciplinary Learning. The different school subjects are just a small puzzle piece of the larger puzzle. They can all go together as one to make up the big puzzle in education.
For instance, what students learn in math can be applied to English, social studies, and science in some capacity, and if not all the subjects, at least some. In order to implement this type of instruction effectively, teachers need to plan and collaborate steadily . Therefore, the overall quality of lessons can be chosen carefully and modified as needed. Cross-curricular planning fosters a great deal of equal responsibility.
Benefits of Cross-Curricular Instruction
As mentioned, teachers are brought together to plan and brainstorm with cross-curricular instruction. Through planning together, teachers and staff members can develop a deeper appreciation of what they each bring to the table as far as expertise and experiences. Additionally, through conversing and establishing one another’s expertise and skills, teachers are more likely to create richer lessons for high-quality instruction across the board.
Not only does this model provide collaboration among teachers, but it does among students as well. Positive social interactions can lead to deeper conversations and the acquisition of new knowledge. Additionally, the idea of teamwork creates opportunities for new discoveries and ideas. Through maintaining positive mindsets and open-mindedness, students can learn to be flexible. As students discover that learning is an ongoing process, they may begin to use what they have already learned to navigate the new difficulties of what they are currently learning. They will come to realize that everything can relate.
Thus, cross-curricular instruction offers many great advantages to students. It fosters teamwork, new discoveries, more motivation, and more knowledge of the learning process. A greater appreciation of the learning process leads students to become life-long learners. For any educator, this is most certainly the goal in mind.
I, myself, am a life-long learner. I have always tried to instill this in my students from the very beginning of the school year. Back when I was a student, it took me a while to see the connections between subject areas to truly say I have learned something new. I then began to feel more comfortable applying my learning to new situations and experiences. Different branches of learning came together, and I was able to see the true importance of the learning process and what it meant for me as a learner.
Strategies for Planning Integrated Curriculum
There are many strategies that can be employed with integrated curriculum. One strategy is for teachers to select a theme for students to learn around. This allows for students to have the opportunity to seek out information they already know and work to learn more, thus leading them to make connections among their learning and new acquisition of knowledge.
Another strategy is for teachers to choose a key concept to guide instruction. Once they establish a theme that is fitting, they can choose tasks within a key concept that encourage students to seek out information, think critically, solve problems, research, and make connections among their learning. This key concept should be directly about the theme but also allow students to transfer what they explore and learn to their own lives. Applying knowledge is an integral part of the learning process, and in this stage, it needs to be a main goal set to be accomplished.
Once themes are determined, the goal is to select tasks that encourage students to investigate, wonder, problem-solve, and ask questions. Key concepts for a theme should provide a clear focus for all instruction and learning. The key concept guides all activities and lessons. It is expected that, by the end of the theme, all students will begin to internalize, build upon, and transfer this key concept to their own lives.
Next, teachers need to identify skills and strategies that need to be taught. Rather than considering a product or result of a lesson, this strategy of identifying skills and strategies that students need allows teachers to plan accordingly to reach mastery of the key concepts. It is certainly not to discount the importance of products and assessments , as they are equally as important. However, teachers can more clearly and concisely plan activities and lessons that will introduce, teach, and enhance key concepts to students. Through this, it creates further opportunities for students to think, navigate, and explore. Then, as the result, they can make connections as a part of cross-curricular instruction.
To go along with the planning aspect of cross-curricular instruction, another strategy that can be utilized by teachers is to ensure that they are choosing appropriate resources. They should take into consideration the scope of planning, templates, material selection, and more.
Teachers need to be reflective of their practice . Teaching is a profession that continuously opens opportunities to identify ways to improve and grow. Teachers need to monitor what students are achieving and gaining and evaluate progress. Through doing so, they can establish what the next steps are in their planning and processes. Of course, if they notice the methods are not working among students, they need to make the necessary modifications, back track, and make another attempt towards student progress and mastery.
References “Integrated Instruction”. March 2015. OEL Resource. http://www.p12.nysed.gov/earlylearning/documents/OELResource_IntegratedInstruction.pdf
*updated february, 2021.
- #CrossCurricularInstruction
More in Teaching Strategies

Write On! Fun Ways to Help Kids Master Pencil Grip
Teaching children proper pencil grip will lay the foundation for successful writing. Holding…

Practical Strategies for Supporting Executive Function in the Classroom
Executive functions are self-regulating skills that we use every single day. Imagine a…

Helping Students Improve Their Handwriting
Despite the widespread use of technology in the classroom, handwriting remains an essential…

Don’t Just Tell Students to Solve Problems. Teach Them How.
The positive impact of an innovative uc san diego problem-solving educational curriculum continues to grow.
- Daniel Kane - [email protected]
Published Date
Share this:, article content.
Problem solving is a critical skill for technical education and technical careers of all types. But what are best practices for teaching problem solving to high school and college students?
The University of California San Diego Jacobs School of Engineering is on the forefront of efforts to improve how problem solving is taught. This UC San Diego approach puts hands-on problem-identification and problem-solving techniques front and center. Over 1,500 students across the San Diego region have already benefited over the last three years from this program. In the 2023-2024 academic year, approximately 1,000 upper-level high school students will be taking the problem solving course in four different school districts in the San Diego region. Based on the positive results with college students, as well as high school juniors and seniors in the San Diego region, the project is getting attention from educators across the state of California, and around the nation and the world.
{/exp:typographee}
In Summer 2023, th e 27 community college students who took the unique problem-solving course developed at the UC San Diego Jacobs School of Engineering thrived, according to Alex Phan PhD, the Executive Director of Student Success at the UC San Diego Jacobs School of Engineering. Phan oversees the project.
Over the course of three weeks, these students from Southwestern College and San Diego City College poured their enthusiasm into problem solving through hands-on team engineering challenges. The students brimmed with positive energy as they worked together.
What was noticeably absent from this laboratory classroom: frustration.
“In school, we often tell students to brainstorm, but they don’t often know where to start. This curriculum gives students direct strategies for brainstorming, for identifying problems, for solving problems,” sai d Jennifer Ogo, a teacher from Kearny High School who taught the problem-solving course in summer 2023 at UC San Diego. Ogo was part of group of educators who took the course themselves last summer.
The curriculum has been created, refined and administered over the last three years through a collaboration between the UC San Diego Jacobs School of Engineering and the UC San Diego Division of Extended Studies. The project kicked off in 2020 with a generous gift from a local philanthropist.
Not getting stuck
One of the overarching goals of this project is to teach both problem-identification and problem-solving skills that help students avoid getting stuck during the learning process. Stuck feelings lead to frustration – and when it’s a Science, Technology, Engineering and Math (STEM) project, that frustration can lead students to feel they don’t belong in a STEM major or a STEM career. Instead, the UC San Diego curriculum is designed to give students the tools that lead to reactions like “this class is hard, but I know I can do this!” – as Ogo, a celebrated high school biomedical sciences and technology teacher, put it.
Three years into the curriculum development effort, the light-hearted energy of the students combined with their intense focus points to success. On the last day of the class, Mourad Mjahed PhD, Director of the MESA Program at Southwestern College’s School of Mathematics, Science and Engineering came to UC San Diego to see the final project presentations made by his 22 MESA students.
“Industry is looking for students who have learned from their failures and who have worked outside of their comfort zones,” said Mjahed. The UC San Diego problem-solving curriculum, Mjahed noted, is an opportunity for students to build the skills and the confidence to learn from their failures and to work outside their comfort zone. “And from there, they see pathways to real careers,” he said.
What does it mean to explicitly teach problem solving?
This approach to teaching problem solving includes a significant focus on learning to identify the problem that actually needs to be solved, in order to avoid solving the wrong problem. The curriculum is organized so that each day is a complete experience. It begins with the teacher introducing the problem-identification or problem-solving strategy of the day. The teacher then presents case studies of that particular strategy in action. Next, the students get introduced to the day’s challenge project. Working in teams, the students compete to win the challenge while integrating the day’s technique. Finally, the class reconvenes to reflect. They discuss what worked and didn't work with their designs as well as how they could have used the day’s problem-identification or problem-solving technique more effectively.
The challenges are designed to be engaging – and over three years, they have been refined to be even more engaging. But the student engagement is about much more than being entertained. Many of the students recognize early on that the problem-identification and problem-solving skills they are learning can be applied not just in the classroom, but in other classes and in life in general.
Gabriel from Southwestern College is one of the students who saw benefits outside the classroom almost immediately. In addition to taking the UC San Diego problem-solving course, Gabriel was concurrently enrolled in an online computer science programming class. He said he immediately started applying the UC San Diego problem-identification and troubleshooting strategies to his coding assignments.
Gabriel noted that he was given a coding-specific troubleshooting strategy in the computer science course, but the more general problem-identification strategies from the UC San Diego class had been extremely helpful. It’s critical to “find the right problem so you can get the right solution. The strategies here,” he said, “they work everywhere.”
Phan echoed this sentiment. “We believe this curriculum can prepare students for the technical workforce. It can prepare students to be impactful for any career path.”
The goal is to be able to offer the course in community colleges for course credit that transfers to the UC, and to possibly offer a version of the course to incoming students at UC San Diego.
As the team continues to work towards integrating the curriculum in both standardized high school courses such as physics, and incorporating the content as a part of the general education curriculum at UC San Diego, the project is expected to impact thousands more students across San Diego annually.
Portrait of the Problem-Solving Curriculum
On a sunny Wednesday in July 2023, an experiential-learning classroom was full of San Diego community college students. They were about half-way through the three-week problem-solving course at UC San Diego, held in the campus’ EnVision Arts and Engineering Maker Studio. On this day, the students were challenged to build a contraption that would propel at least six ping pong balls along a kite string spanning the laboratory. The only propulsive force they could rely on was the air shooting out of a party balloon.
A team of three students from Southwestern College – Valeria, Melissa and Alondra – took an early lead in the classroom competition. They were the first to use a plastic bag instead of disposable cups to hold the ping pong balls. Using a bag, their design got more than half-way to the finish line – better than any other team at the time – but there was more work to do.
As the trio considered what design changes to make next, they returned to the problem-solving theme of the day: unintended consequences. Earlier in the day, all the students had been challenged to consider unintended consequences and ask questions like: When you design to reduce friction, what happens? Do new problems emerge? Did other things improve that you hadn’t anticipated?
Other groups soon followed Valeria, Melissa and Alondra’s lead and began iterating on their own plastic-bag solutions to the day’s challenge. New unintended consequences popped up everywhere. Switching from cups to a bag, for example, reduced friction but sometimes increased wind drag.
Over the course of several iterations, Valeria, Melissa and Alondra made their bag smaller, blew their balloon up bigger, and switched to a different kind of tape to get a better connection with the plastic straw that slid along the kite string, carrying the ping pong balls.
One of the groups on the other side of the room watched the emergence of the plastic-bag solution with great interest.
“We tried everything, then we saw a team using a bag,” said Alexander, a student from City College. His team adopted the plastic-bag strategy as well, and iterated on it like everyone else. They also chose to blow up their balloon with a hand pump after the balloon was already attached to the bag filled with ping pong balls – which was unique.
“I don’t want to be trying to put the balloon in place when it's about to explode,” Alexander explained.
Asked about whether the structured problem solving approaches were useful, Alexander’s teammate Brianna, who is a Southwestern College student, talked about how the problem-solving tools have helped her get over mental blocks. “Sometimes we make the most ridiculous things work,” she said. “It’s a pretty fun class for sure.”
Yoshadara, a City College student who is the third member of this team, described some of the problem solving techniques this way: “It’s about letting yourself be a little absurd.”
Alexander jumped back into the conversation. “The value is in the abstraction. As students, we learn to look at the problem solving that worked and then abstract out the problem solving strategy that can then be applied to other challenges. That’s what mathematicians do all the time,” he said, adding that he is already thinking about how he can apply the process of looking at unintended consequences to improve both how he plays chess and how he goes about solving math problems.
Looking ahead, the goal is to empower as many students as possible in the San Diego area and beyond to learn to problem solve more enjoyably. It’s a concrete way to give students tools that could encourage them to thrive in the growing number of technical careers that require sharp problem-solving skills, whether or not they require a four-year degree.
You May Also Like
Liquid droplets shape how cells respond to change, serving up sustainability and eliminating food waste in hospital cafeterias, breakthrough study on post-traumatic stress disorder, creating a “greener,” more connected society, stay in the know.
Keep up with all the latest from UC San Diego. Subscribe to the newsletter today.
You have been successfully subscribed to the UC San Diego Today Newsletter.
Campus & Community
Arts & culture, visual storytelling.
- Media Resources & Contacts
Signup to get the latest UC San Diego newsletters delivered to your inbox.
Award-winning publication highlighting the distinction, prestige and global impact of UC San Diego.
Popular Searches: Covid-19 Ukraine Campus & Community Arts & Culture Voices
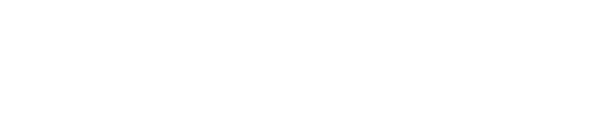
Or search by topic
Number and algebra
- The Number System and Place Value
- Calculations and Numerical Methods
- Fractions, Decimals, Percentages, Ratio and Proportion
- Properties of Numbers
- Patterns, Sequences and Structure
- Algebraic expressions, equations and formulae
- Coordinates, Functions and Graphs
Geometry and measure
- Angles, Polygons, and Geometrical Proof
- 3D Geometry, Shape and Space
- Measuring and calculating with units
- Transformations and constructions
- Pythagoras and Trigonometry
- Vectors and Matrices
Probability and statistics
- Handling, Processing and Representing Data
- Probability
Working mathematically
- Thinking mathematically
- Mathematical mindsets
- Cross-curricular contexts
- Physical and digital manipulatives
For younger learners
- Early Years Foundation Stage
Advanced mathematics
- Decision Mathematics and Combinatorics
- Advanced Probability and Statistics
Published 2013 Revised 2019
Problem Solving and the New Curriculum
- seeking solutions not just memorising procedures
- exploring patterns not just memorising formulas
- formulating conjectures, not just doing exercises.
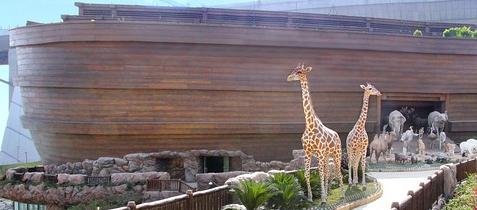
Noah watched the animals going into the ark. He was counting and by noon he got to $12$, but he was only counting the legs of the animals. How many creatures did he see? See if you can find other answers? Try to tell someone how you found these answers out?
Planning a School Trip
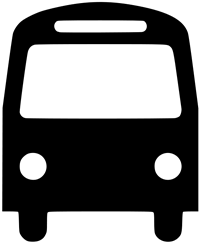
This activity is taken from the ATM publication "We Can Work It Out!", a book of collaborative problem solving activity cards by Anitra Vickery and Mike Spooner. It is available from The Association of Teachers of Mathematics https://www.atm.org.uk/Shop/Primary-Education/Primary-Education-Books/Books--Hardcopy/We-Can-Work-It-Out-1/act054
References Polya, G. 1945) How to Solve It. Princeton University Press Schoenfeld, A.H. (1992) Learning to think mathematically: problem solving, metacognition and sense-making in mathematics. In D.Grouws (ed) Handbook for Research on Mathematics Teaching and Learning (pp334-370) New York: MacMillan
Lampert m (1992) quoted in schoenfeld, above..
- Free Resources
- 0 items £0.00
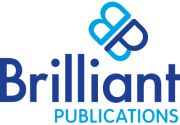
Problem Solving Across the Curriculum
The Problem Solving Across the Curriculum series will give pupils the skills, strategies, and disposition needed to become lifelong problem solvers.
The series provides teachers with a wide range of problematic situations for which there is more than one solution or more than one approach to the solution. The activities can easily be integrated into the following curriculum areas:
- Visual literacy
- PSHE/Values
- Music and dance
£ 40.00 price excluding tax
Share this item:
Description, additional information.
- Reviews (0)
- Try before you buy
- Video Attachments
A PACK SAVING OF £10.97
Problem Solving Across the Curriculum can be used to complement any classroom work across the curriculum. The activities can be used in isolation, in sequence, or dipped into as teachers require.
The activities in the Problem Solving Across the Curriculum series present a wide range of problematic situations for which there is more than one solution or more than one approach to the solution. In real life problems are not usually neatly presented nor are the solutions immediately visible. When a potential solution is found, it needs to be evaluated to see if it is the best solution possible.
These problem-solving activities will lay the foundational skills, strategies and disposition needed for pupils to become lifelong problem solvers.
There are five different types of problems presented:
- Mathematical problems – focus on using estimation efficient number strategies, shape and position with flexibility and strategic thinking.
- Visual literacy problems – focus on using elements of visual language (colour, position, shape and planning) to convey ideas, create mood and develop visual flexibility and creativity.
- Music and dance problems – focus on developing perceptions about the ways in which rhythm, pattern, sound and movement can be combined to create solutions to musical challenges.
- Values problems – focus on reflecting and evaluating responses to everyday problem situations where interpersonal or ethical decisions need to be made. They provide an opportunity for philosophical thinking.
- English problems – focus on communicating clearly through spoken and written language specific to the task, purpose and audience.
There are no reviews yet.
You must be logged in to post a review.
Every picture tells a story. What is this picture telling you? (From 5-7 year olds book)
A creative task to make 'talking heads'. (from 5-7 year olds book), why do companies advertise how does it work (from 7-9 year olds book), can you make the device described on this task card and write instructions for someone else to make one (from 7-9 year olds book), a film sound track really sets the scene. have a go at creating your own. (from 9-11 year olds book), work in a group to write some interesting opening lines for stories (from 9-11 year olds book), you may also like….
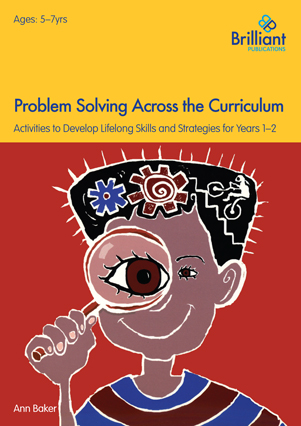
Problem Solving Across the Curriculum for 5 – 7 Year Olds
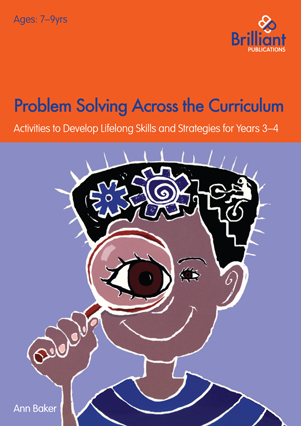
Problem Solving Across the Curriculum for 7 – 9 Year Olds
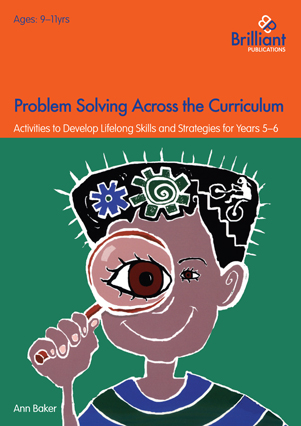
Problem Solving Across the Curriculum for 9 – 11 Year Olds
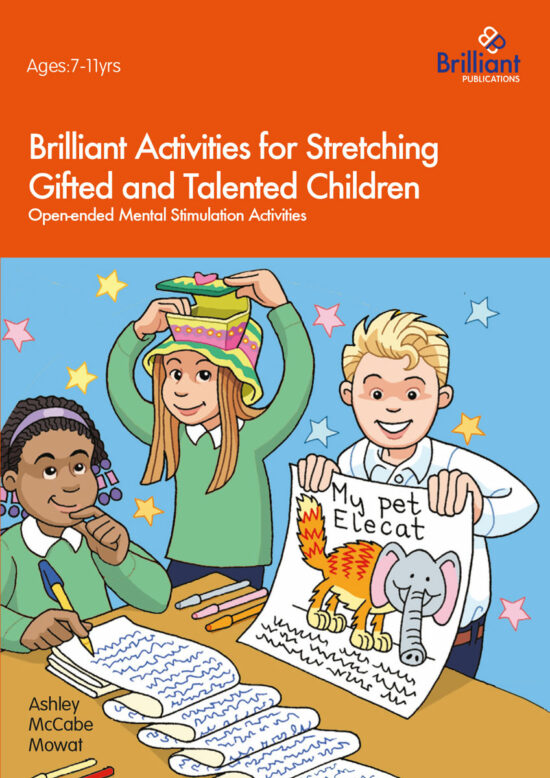
Brilliant Activities for Stretching Gifted and Talented Children

- Education & Teaching
- Schools & Teaching

Enjoy fast, free delivery, exclusive deals, and award-winning movies & TV shows with Prime Try Prime and start saving today with fast, free delivery
Amazon Prime includes:
Fast, FREE Delivery is available to Prime members. To join, select "Try Amazon Prime and start saving today with Fast, FREE Delivery" below the Add to Cart button.
- Cardmembers earn 5% Back at Amazon.com with a Prime Credit Card.
- Unlimited Free Two-Day Delivery
- Streaming of thousands of movies and TV shows with limited ads on Prime Video.
- A Kindle book to borrow for free each month - with no due dates
- Listen to over 2 million songs and hundreds of playlists
- Unlimited photo storage with anywhere access
Important: Your credit card will NOT be charged when you start your free trial or if you cancel during the trial period. If you're happy with Amazon Prime, do nothing. At the end of the free trial, your membership will automatically upgrade to a monthly membership.
Buy new: $58.95
Return this item for free.
Free returns are available for the shipping address you chose. You can return the item for any reason in new and unused condition: no shipping charges
- Go to your orders and start the return
- Select the return method

Download the free Kindle app and start reading Kindle books instantly on your smartphone, tablet, or computer - no Kindle device required .
Read instantly on your browser with Kindle for Web.
Using your mobile phone camera - scan the code below and download the Kindle app.

Image Unavailable

- To view this video download Flash Player
Follow the author

Mathematics Across the Curriculum: Problem-Solving, Reasoning and Numeracy in Primary Schools Illustrated Edition
Purchase options and add-ons.
Mathematics is a core subject and using and applying mathematics in problem solving activities is crucial in enabling children to use their knowledge and skills in a range of situations. Mathematics Across the Curriculum shows how to teach mathematical concepts through different subjects and discussing the reasoning and research behind using problem solving and investigation teaching techniques. Best practice for planning and assessment, classroom organisation and practice, and use of resources are all discussed, with clear links to recent research and government standards and initiatives. Case studies from practicing teachers enable readers to easily relate the theoretical information to their classroom and teaching. Mathematics Across the Curriculum is essential reading for all trainee and newly qualified teachers seeking to teach engaging, inspiring and challenging mathematics lessons. It will also be a useful resource for established teachers embarking on their Masters in Teaching and Learning (MTL).
- ISBN-10 1441123563
- ISBN-13 978-1441123565
- Edition Illustrated
- Publisher Continuum
- Publication date July 8, 2010
- Language English
- Dimensions 7.44 x 0.42 x 9.69 inches
- Print length 200 pages
- See all details

Editorial Reviews
About the author, product details.
- Publisher : Continuum; Illustrated edition (July 8, 2010)
- Language : English
- Paperback : 200 pages
- ISBN-10 : 1441123563
- ISBN-13 : 978-1441123565
- Item Weight : 14.1 ounces
- Dimensions : 7.44 x 0.42 x 9.69 inches
- #6,049 in Elementary Education
- #9,241 in Math Teaching Materials
- #565,638 in Unknown
About the author
Discover more of the author’s books, see similar authors, read author blogs and more
Customer reviews
Customer Reviews, including Product Star Ratings help customers to learn more about the product and decide whether it is the right product for them.
To calculate the overall star rating and percentage breakdown by star, we don’t use a simple average. Instead, our system considers things like how recent a review is and if the reviewer bought the item on Amazon. It also analyzed reviews to verify trustworthiness.
No customer reviews
- Amazon Newsletter
- About Amazon
- Accessibility
- Sustainability
- Press Center
- Investor Relations
- Amazon Devices
- Amazon Science
- Sell on Amazon
- Sell apps on Amazon
- Supply to Amazon
- Protect & Build Your Brand
- Become an Affiliate
- Become a Delivery Driver
- Start a Package Delivery Business
- Advertise Your Products
- Self-Publish with Us
- Become an Amazon Hub Partner
- › See More Ways to Make Money
- Amazon Visa
- Amazon Store Card
- Amazon Secured Card
- Amazon Business Card
- Shop with Points
- Credit Card Marketplace
- Reload Your Balance
- Amazon Currency Converter
- Your Account
- Your Orders
- Shipping Rates & Policies
- Amazon Prime
- Returns & Replacements
- Manage Your Content and Devices
- Recalls and Product Safety Alerts
- Conditions of Use
- Privacy Notice
- Consumer Health Data Privacy Disclosure
- Your Ads Privacy Choices
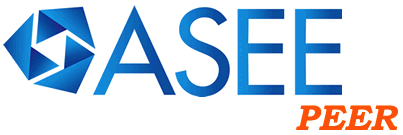
Numerical Problem Solving across the Curriculum with Python and MATLAB Using Interactive Coding Templates: A Workshop for Chemical Engineering Faculty
Download Paper | Permalink
2023 ASEE Annual Conference & Exposition
Baltimore , Maryland
June 25, 2023
June 28, 2023
Chemical Engineering Division (ChED) Technical Session 7: Innovative Pedagogy
Chemical Engineering Division (ChED)
10.18260/1-2--43749
https://peer.asee.org/43749
Paper Authors
Austin n. johns the state university of new york, buffalo.
visit author page
Austin N. Johns is an active-duty captain and developmental engineer in the United States Air Force. In 2017, he earned a B.S. in Chemical Engineering from Oklahoma State University. In 2023, he earned a M.S. in Chemical Engineering from the University at Buffalo, The State University of New York. His graduate research focused on developing computational educational resources for use in the chemical engineering curriculum. The views expressed in this paper are those of the author and do not reflect the official policy or position of the United States Air Force, Department of Defense, or the U.S. Government.
Robert P. Hesketh Rowan University
Robert Hesketh is a Professor of Chemical Engineering at Rowan University. He received his B.S. in 1982 from the University of Illinois and his Ph.D. from the University of Delaware in 1987. After his Ph.D. he conducted research at the University of Cam
Matthew D. Stuber University of Connecticut
Dr. Matt Stuber is an Assistant Professor with the Dept. of Chemical & Biomolecular Engineering and the Institute for Advanced Systems Engineering at the University of Connecticut. He received his PhD from the Massachusetts Institute of Technology (MIT) and his BS from the University of Minnesota – Twin cities, both in chemical engineering. In his post-doctoral work, he cofounded a water-tech start-up company focusing on developing flexible high-efficiency solar-driven desalination technologies for diverse applications where membrane technologies prove inadequate. At UConn, his core research focus is on optimization theory, methods, and software for modeling and simulation, robust simulation and design, and controls and operations. His application interests lie in addressing challenging and timely applications from a spectrum of industries including food, energy, water and natural resources, chemicals, finance, and healthcare. The systems-level thinking combined with quantitative rigor enables the development of novel solutions to emerging and intractable problems across these diverse areas.
Ashlee N. Ford Versypt The State University of New York, Buffalo orcid.org/0000-0001-9059-5703
Dr. Ashlee N. Ford Versypt is an Associate Professor in the Department of Chemical and Biological Engineering at the University at Buffalo (UB), The State University of New York. She is also an Affiliated Faculty in the Department of Engineering Education and the Institute for Artificial Intelligence and Data Science. She received her B.S. from the University of Oklahoma and her M.S. and Ph.D. from the University of Illinois. She did a postdoc at the Massachusetts Institute of Technology before starting her academic career at Oklahoma State University (OSU), where she was an assistant professor 2014-2020 and then a tenured associate professor until January 2021 before moving to UB. Dr. Ford Versypt leads the Systems Biomedicine and Pharmaceutics Laboratory. She was the 2020-2021 Chair for the ASEE Chemical Engineering Division (CHED). Dr. Ford Versypt has been recognized with the NSF CAREER Award, ASEE CHED Ray W. Fahien Award and Joseph J. Martin Award, and AIChE CAST Division David Himmelblau Award for Innovations in Computer-Based Chemical Engineering Education. She is an Academic Trustee of Computer Aids for Chemical Engineering Corporation.
With the fourth industrial revolution well underway, the proportion of occupations requiring “high” or “medium” digital skills has never been greater. Among those most in demand are engineers skilled in computing and advanced problem solving to support the ongoing digitalization, networking, and automation. A numerical analysis course in the core undergraduate engineering curriculum is a natural place for students to learn numerical methods for advanced problem solving across engineering applications. The use of computing across the entire chemical engineering curriculum also offers opportunities to hone students’ abilities as computational thinkers and effective problem solvers to meet the current and future needs of an increasingly complex and digital industry and society. While the current chemical engineering curriculum includes computational training, there is a need to efficiently increase the exposure of students to computing within mathematical problem-solving contexts and develop their proficiency in computer programming, all while balancing demands to reduce credit hours. Some chemical engineering faculty interested in enhancing the computational nature of their courses face a barrier to doing so due to unfamiliarity with some modern computational educational resources that may not have been covered in their training or may not be used in their research areas. The authors developed a workshop to teach chemical engineering faculty to use and develop interactive coding templates (MATLAB Live Scripts and Jupyter Notebooks) and to equip faculty to incorporate these techniques across the undergraduate curriculum. The workshop was presented at the 2022 ASEE/AIChE Summer School for Engineering Faculty. The purpose of this paper is to disseminate the workshop resources, providing educators with a suite of interactive templates focused on chemical engineering-related case studies and with training to create and adapt their own related materials. The paper details the interactive coding templates provided during the workshop along with the relevant pedagogical background and some lessons learned for future related workshops. Educators who did not attend the workshop are also a target audience of this paper as it provides tips and access to the relevant materials for implementing computational thinking through interactive coding templates into their classroom practices.
APA - LaTeX bibitem
Mla - latex bibitem, endnote - ris.
Johns, A. N., & Hesketh, R. P., & Stuber, M. D., & Ford Versypt, A. N. (2023, June), Numerical Problem Solving across the Curriculum with Python and MATLAB Using Interactive Coding Templates: A Workshop for Chemical Engineering Faculty Paper presented at 2023 ASEE Annual Conference & Exposition, Baltimore , Maryland. 10.18260/1-2--43749
ASEE holds the copyright on this document. It may be read by the public free of charge. Authors may archive their work on personal websites or in institutional repositories with the following citation: © 2023 American Society for Engineering Education. Other scholars may excerpt or quote from these materials with the same citation. When excerpting or quoting from Conference Proceedings, authors should, in addition to noting the ASEE copyright, list all the original authors and their institutions and name the host city of the conference. - Last updated April 1, 2015
Climate advocates want to solve their 'biggest problem' in the US: Turning out voters
ABC News is taking a look at solutions for issues related to climate change and the environment with the series "The Power of Us: People, The Climate, and Our Future."
In battleground states across the country, environmental activists like Dr. Emily Church are canvassing on behalf of an organization called the Environmental Voter Project in an effort to turn out people who care the most about climate change -- but who haven't shown up for past elections.
During a recent effort in Pittsburgh, Church, a biology professor who leads local canvasses for the project, recalled to ABC News how she used to lobby lawmakers directly to take action on climate change, but they told her voters don't care about the issue.
She said she's now trying to prove them wrong.
"The people who prioritize climate and the environment need to show up," Church said. "That's how we're going to get anything done."
The Environmental Voter Project, or EVP, is targeting very specific individuals: registered voters who list climate change as their No. 1 issue but who are unlikely to cast ballots in November's election based on their voting history.
"Our biggest problem in the climate movement right now [is] we don't have enough voting power," EVP founder and executive Nathaniel Stinnett said.
EVP takes a targeted approach to door knocking, Stinnett explained. Using polling, the group first determines which registered voters in a particular area, like Pittsburgh, would rank climate as their top voting issue. They then cross-reference profiles with voting records to find people who have not come out to the polls recently or regularly.
By Stinnett's accounting, the group has been successful across general elections, primaries and even in local races.
"We've sometimes increased turnout by as much as 1.8 percentage points in general elections, 3.6 points in primaries and 5.7 points in local elections," he said, noting that while 1.8 percentage points might sound small, it could determine an election. Pennsylvania, for example, was only won by President Joe Biden in 2020 by 1.17%.
For the canvassing effort in Pittsburgh, Stinnett said EVP targeted people who didn't vote in the 2020 election or elections in the years since. He added that they identified 22,135 voters in the city who are highly likely to rank climate as their top priority but unlikely to vote in November.
The group claims nonpartisanship but acknowledges that right now it's Democrats working on climate change almost exclusively. One of their hopes is to bring more Republicans to the table, too.
"We want to scare the bejesus out of as many politicians as possible, no matter what side of the aisle they're on, until they think, 'You know what, the only way I can win elections is if I start recognizing the biggest crisis,'" Stinnett said.
Over time, climate change has become a more salient voting issue. In 2010, only a slim majority of Americans agreed that global warming was occurring, according to polling by the Yale Program on Climate Change Communication . Now, 72% of Americans agree.
But climate is currently not one of the biggest motivators for people this election cycle, surveys have indicated -- though climate advocates hope to change the electorate by encouraging turnout of climate-concerned voters.
According to a February poll by the Wall Street Journal , registered voters listed immigration (20%), the economy (14%), abortion (8%) and democracy (8%) as their top issues. Climate change ranked 11th, with 2% of voters choosing it as their top issue.
More broadly, Gallup's tracking of what Americans say is the country's most important problem over time shows climate, pollution and the environment at 2% in March, far below economic issues and immigration.
Polling has also shown that in addition to a partisan divide on the issue, a generational shift may be at play.
"Young voters in general tend to be more Democratic, and that is kind of tied up inextricably with their belief that climate is really important," said Nathaniel Rakich, a senior editor and senior elections analyst at 538. "So if Republicans don't want to basically be losing this upcoming electorate by large margins for decades to come, they're going to have to eat into that Democratic support by at least proposing some solutions and addressing climate change."
Even the Biden administration, which has prioritized fighting climate change, is being pushed by progressives to do more on the issue.
Twenty-one activists with the environmental advocacy group Sunrise Movement were arrested outside of Biden campaign headquarters in Wilmington, Delaware, in February. That group and other advocates have additional demonstrations planned in the run-up to the November election.
"I think there were some missteps by the administration -- permitting the Willow project in Alaska was a step backwards. That was unfortunate," Evergreen Action Executive Director Lena Moffitt said, referring to a large-scale oil drilling initiative backed by Alaska lawmakers and others in the state for its economic value, but which environmentalists criticized as undercutting the White House's climate goals.
"We know that we need to move away from fossil fuels and, at the same time, the administration is doing a lot to hasten that move away from fossil fuels," Moffitt said.
The choice for voters in November, on the issue of climate, is stark. President Biden has spoken urgently of the dangers of not slowing climate change and has pushed renewable energy solutions, backed electric vehicle infrastructure and created a new Climate Corps to train and expand the environmental workforce.
Biden last week finalized new protections against oil and gas production for some13 million acres of land in Alaska and, through the Environmental Protection Agency, has imposed aggressive emissions standards for vehicles to cut future greenhouse gases.
Meanwhile, former President Donald Trump, who has long questioned climate science, without evidence, has opposed Biden's clean energy policies and promised to roll them back -- arguing they are a drag on the economy and make the U.S. less competitive and independent.
"The fact is President Biden has done more to address climate change than any president in U.S. history. And there's a lot more to be done," Moffitt said. "Scientists have said that we still can avoid the worst of the worst of the climate crisis. But what we do in these next few years is essential to which path we choose."
Stinnett agreed, telling ABC News that too often Americans have been told to focus on their own individual habits rather than government policy.
"[Politicians say,] 'Hey, don't pay attention to that coal-fired power plant back there. Instead, it's all your fault for having a plastic water bottle in your hand.' And we bought it. We bought it hook, line and sinker," he said. "In truth, it is far more of a political and a systemic problem that needs political and systemic solutions."
In Pittsburgh, Church said that despite the difficulty in getting new, environmentally minded voters to the polls, she thinks the challenge is worth it.
"The science is very clear. So we know what we need to do," she said, "it's just a matter of getting it done."
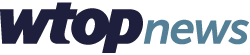
Climate advocates want to solve their ‘biggest problem’ in the US: Turning out voters
April 21, 2024, 4:45 PM
- Share This:
- share on facebook
- share on threads
- share on linkedin
- share on email

In battleground states across the country, environmental activists like Dr. Emily Church are canvassing on behalf of an organization called the Environmental Voter Project in an effort to turn out people who care the most about climate change — but who haven’t shown up for past elections.
During a recent effort in Pittsburgh, Church, a biology professor who leads local canvasses for the project, recalled to ABC News how she used to lobby lawmakers directly to take action on climate change, but they told her voters don’t care about the issue.
She said she’s now trying to prove them wrong.
“The people who prioritize climate and the environment need to show up,” Church said. “That’s how we’re going to get anything done.”
The Environmental Voter Project, or EVP, is targeting very specific individuals: registered voters who list climate change as their No. 1 issue but who are unlikely to cast ballots in November’s election based on their voting history.
“Our biggest problem in the climate movement right now [is] we don’t have enough voting power,” EVP founder and executive Nathaniel Stinnett said.
EVP takes a targeted approach to door knocking, Stinnett explained. Using polling, the group first determines which registered voters in a particular area, like Pittsburgh, would rank climate as their top voting issue. They then cross-reference profiles with voting records to find people who have not come out to the polls recently or regularly.
By Stinnett’s accounting, the group has been successful across general elections, primaries and even in local races.
“We’ve sometimes increased turnout by as much as 1.8 percentage points in general elections, 3.6 points in primaries and 5.7 points in local elections,” he said, noting that while 1.8 percentage points might sound small, it could determine an election. Pennsylvania, for example, was only won by President Joe Biden in 2020 by 1.17%.
For the canvassing effort in Pittsburgh, Stinnett said EVP targeted people who didn’t vote in the 2020 election or elections in the years since. He added that they identified 22,135 voters in the city who are highly likely to rank climate as their top priority but unlikely to vote in November.
The group claims nonpartisanship but acknowledges that right now it’s Democrats working on climate change almost exclusively. One of their hopes is to bring more Republicans to the table, too.
“We want to scare the bejesus out of as many politicians as possible, no matter what side of the aisle they’re on, until they think, ‘You know what, the only way I can win elections is if I start recognizing the biggest crisis,'” Stinnett said.
Over time, climate change has become a more salient voting issue. In 2010, only a slim majority of Americans agreed that global warming was occurring, according to polling by the Yale Program on Climate Change Communication. Now, 72% of Americans agree.
But climate is currently not one of the biggest motivators for people this election cycle, surveys have indicated — though climate advocates hope to change the electorate by encouraging turnout of climate-concerned voters.
According to a February poll by the Wall Street Journal, registered voters listed immigration (20%), the economy (14%), abortion (8%) and democracy (8%) as their top issues. Climate change ranked 11th, with 2% of voters choosing it as their top issue.
More broadly, Gallup’s tracking of what Americans say is the country’s most important problem over time shows climate, pollution and the environment at 2% in March, far below economic issues and immigration.
Polling has also shown that in addition to a partisan divide on the issue, a generational shift may be at play.
“Young voters in general tend to be more Democratic, and that is kind of tied up inextricably with their belief that climate is really important,” said Nathaniel Rakich, a senior editor and senior elections analyst at 538. “So if Republicans don’t want to basically be losing this upcoming electorate by large margins for decades to come, they’re going to have to eat into that Democratic support by at least proposing some solutions and addressing climate change.”
Even the Biden administration, which has prioritized fighting climate change, is being pushed by progressives to do more on the issue.
Twenty-one activists with the environmental advocacy group Sunrise Movement were arrested outside of Biden campaign headquarters in Wilmington, Delaware, in February. That group and other advocates have additional demonstrations planned in the run-up to the November election.
“I think there were some missteps by the administration — permitting the Willow project in Alaska was a step backwards. That was unfortunate,” Evergreen Action Executive Director Lena Moffitt said, referring to a large-scale oil drilling initiative backed by Alaska lawmakers and others in the state for its economic value, but which environmentalists criticized as undercutting the White House’s climate goals.
“We know that we need to move away from fossil fuels and, at the same time, the administration is doing a lot to hasten that move away from fossil fuels,” Moffitt said.
The choice for voters in November, on the issue of climate, is stark. President Biden has spoken urgently of the dangers of not slowing climate change and has pushed renewable energy solutions, backed electric vehicle infrastructure and created a new Climate Corps to train and expand the environmental workforce.
Biden last week finalized new protections against oil and gas production for some13 million acres of land in Alaska and, through the Environmental Protection Agency, has imposed aggressive emissions standards for vehicles to cut future greenhouse gases.
Meanwhile, former President Donald Trump, who has long questioned climate science, without evidence, has opposed Biden’s clean energy policies and promised to roll them back — arguing they are a drag on the economy and make the U.S. less competitive and independent.
“The fact is President Biden has done more to address climate change than any president in U.S. history. And there’s a lot more to be done,” Moffitt said. “Scientists have said that we still can avoid the worst of the worst of the climate crisis. But what we do in these next few years is essential to which path we choose.”
Stinnett agreed, telling ABC News that too often Americans have been told to focus on their own individual habits rather than government policy.
“[Politicians say,] ‘Hey, don’t pay attention to that coal-fired power plant back there. Instead, it’s all your fault for having a plastic water bottle in your hand.’ And we bought it. We bought it hook, line and sinker,” he said. “In truth, it is far more of a political and a systemic problem that needs political and systemic solutions.”
In Pittsburgh, Church said that despite the difficulty in getting new, environmentally-minded voters to the polls, she thinks the challenge is worth it.
“The science is very clear. So we know what we need to do,” she said, “it’s just a matter of getting it done.”
Copyright © 2024, ABC Audio. All rights reserved.
Related News
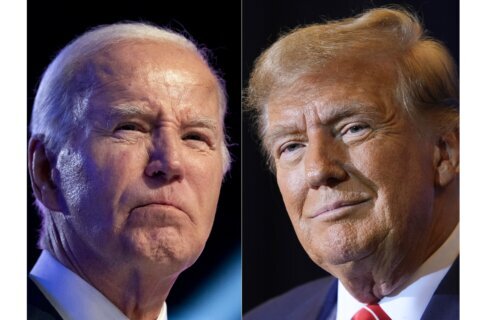
In race for his Senate seat, Joe Manchin endorses West Virginia Democratic Mayor Glenn Elliott
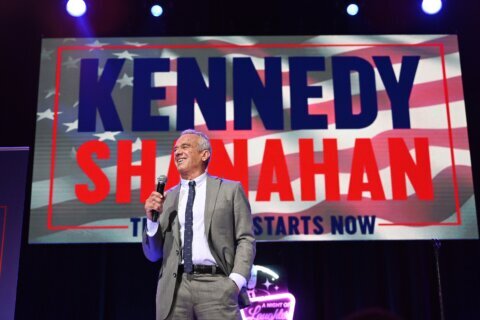
Kennedy says he loves his family ‘either way’ after relatives endorse Biden’s campaign over his
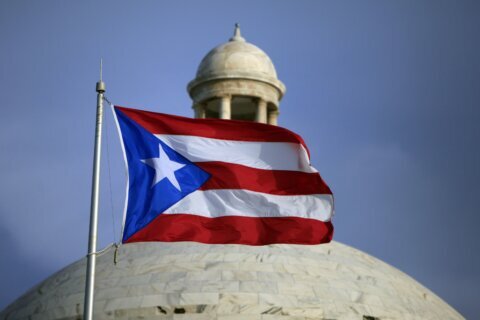
Puerto Rico Republicans award Trump all 23 of their delegates
Recommended.
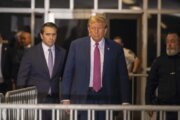
Trump tried to 'corrupt' the 2016 election, prosecutor alleges as hush money trial gets underway
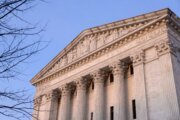
Supreme Court will take up the legal fight over ghost guns, firearms without serial numbers
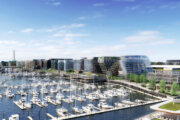
DC's most expensive street? Maine Avenue
Related categories:.

IMAGES
VIDEO
COMMENTS
Algorithmic design - making steps and rules to solve problems. Most problems will require students to employ multiple strategies. Julie Evans, CEO of the education nonprofit Project Tomorrow, illustrated that point by asking attendees at one session to draw a cat in less than 30 seconds.No drawing looked exactly the same, but the participating educators had to quickly break their mental image ...
Computational Thinking Across the Curriculum. Four of the skills used to solve computer science problems can be applied in other classes as well. As defined by Jeannette Wing, computational thinking is "a way of solving problems, designing systems, and understanding human behavior by drawing on the concepts of computer science.".
Problems and problem solving may be context and discipline specific, but the concept and process have overarching components and similarities across contexts. Jonassen (2000, p. 65) defines a problem as an "unknown entity in some situation (the difference between a goal state and a current state)" such that "finding or solving for the ...
The Problem Solving Experience: Classroom Curriculum Designed to Promote Problem Solving in the 21st Century is a complete curriculum targeted at grades 5-8, and portions can be adjusted for use with younger or older students.The curriculum can be implemented as a full semester course, or spread across 1-4 school years. Complete lesson plans ...
A problem-centred curriculum is a cross-curricular, inquiry-based, student-centred approach that is built around real-world problems. ... problem-solving skills, higher-order thinking skills, and self-directed learning skills which are transferable across disciplines (Savery, 2006). Students should be given opportunities to reflect on what they ...
— The Problem Solving Experience Curriculum - targeted at grades 5-8 to be implemented either as a full course, or spread out over 1-4 years, with complete lesson plans included. — Problem Solving Across the Curriculum (PSAC) - provides hundred of Future Scenes that can be modified for any classroom or grade level to give students the ...
the teachers to infuse the problem solving approach across the broader curriculum. In the first phase, one of the authors - hereafter known as the trainer - used problems as examples to help develop teachers' problem solving habits within Pólya's (1954) stages
INTRODUCTION. There is a widespread consensus across many countries that problem solving is a fundamental aspect within the school mathematics curriculum, and it appears prominently in mathematics curricula around the world (Törner et al., 2007).Problem solving is critical for life in the modern world and a central aspect of mathematics (ACME, 2011, 2016; English & Gainsburg, 2016; English ...
10. Connect students with change makers. Entrepreneurs all over the world are using the processes students use in GPS: The Series. Put your students in touch with them to bring concepts to life. GPS: The Series offers six videos called "The Putri Files", where GPS team leader Putri interviews these entrepreneurs.
The Problem-Solving Routine. At Two Rivers, we use a fairly simple routine for problem solving that has five basic steps. The power of this structure is that it becomes a routine that students are able to use regularly across multiple contexts. The first three steps are implemented before problem-solving. Students use one step during problem ...
OUR PROBLEM-SOLVING CURRICULUM. Our curriculum features 13 modules that focus on building the relevant skills and knowledge for effective problem solving. We cover a wide variety of areas including problem identification, assumption reduction, and solution development.
On the basis of a 'problem solving as an educational outcome' point of view, we analyse the contribution of math and science competence to analytical problem-solving competence and link the acquisition of problem solving competence to the coherence between math and science education. We propose the concept of math-science coherence and explore whether society-, curriculum-, and school ...
The three steps before problem-solving: We call them the K-W-I. The "K" stands for "know" and requires students to identify what they already know about a problem. The goal in this step of ...
Elementary students practice problem-solving and self-questioning techniques to improve reading and social and emotional learning skills. ... who works as both the district's K-12 director of curriculum and principal of ... "I recommended Moss teachers teach just one problem-solving process to our 6-year-olds across all academic content ...
for advanced problem solving across engineering applications. The use of computing across the entire chemical engineering curriculum also offers opportunities to hone students' abilities as computational thinkers and effective problem solvers to meet the current and future needs of an increasingly complex and digital industry and society.
Cross-curricular instruction is a great way for teachers to incorporate different disciplines in education into one. It can also be referred to as Interdisciplinary Learning. The different school subjects are just a small puzzle piece of the larger puzzle. They can all go together as one to make up the big puzzle in education.
Mathematics is a core subject and using and applying mathematics in problem solving activities is crucial in enabling children to use their knowledge and skills in a range of situations. Mathematics Across the Curriculum shows how to teach mathematical concepts through different subjects and discussing the reasoning and research behind using problem solving and investigation teaching techniques.
Mathematics Across the Curriculum shows how to teach mathematical concepts through different subjects and discussing the reasoning and research behind using problem solving and investigation teaching techniques. Best practice for planning and assessment, classroom organisation and practice, and use of resources are all discussed, with clear ...
The University of California San Diego Jacobs School of Engineering is on the forefront of efforts to improve how problem solving is taught. This UC San Diego approach puts hands-on problem-identification and problem-solving techniques front and center. Over 1,500 students across the San Diego region have already benefited over the last three ...
Problem solving in Polya's view is about engaging with real problems; guessing, discovering, and making sense of mathematics. (Real problems don't have to be 'real world' applications, they can be within mathematics itself. The main criterion is that they should be non-routine and new to the student.) Compared to the interpretation as a set of ...
The Problem Solving Across the Curriculum series will give pupils the skills, strategies, and disposition needed to become lifelong problem solvers. The series provides teachers with a wide range of problematic situations for which there is more than one solution or more than one approach to the solution. The activities can easily be integrated into the following curriculum areas: Maths ...
Mathematics Across the Curriculum shows how to teach mathematical concepts through different subjects and discussing the reasoning and research behind using problem solving and investigation teaching techniques. Best practice for planning and assessment, classroom organisation and practice, and use of resources are all discussed, with clear ...
Austin N. Johns, Robert P. Hesketh, Matthew D. Stuber, and Ashlee N. Ford Versypt. "Numerical Problem Solving across the Curriculum with Python and MATLAB Using Interactive Coding Templates: A Workshop for Chemical Engineering Faculty". 2023 ASEE Annual Conference & Exposition, Baltimore , Maryland, 2023, June. ASEE Conferences, 2023.
By Stinnett's accounting, the group has been successful across general elections, primaries and even in local races. "We've sometimes increased turnout by as much as 1.8 percentage points in ...
ABC News. In battleground states across the country, environmental activists like Dr. Emily Church are canvassing on behalf of an organization called the Environmental Voter Project in an effort ...