Browse Course Material
Course info.
- Karen Boiko

Departments
- Comparative Media Studies/Writing
As Taught In
- Academic Writing
- Creative Writing
- Nonfiction Prose
Learning Resource Types
The science essay, course description.
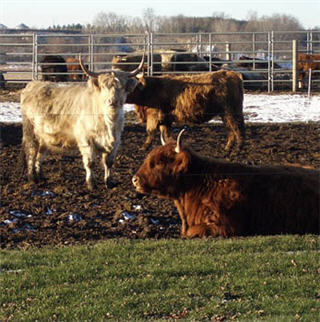
You are leaving MIT OpenCourseWare
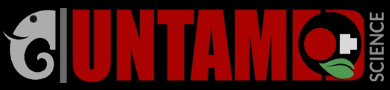
- Blog Post Writing in Science and Technology Classrooms: Writing Exercises
Writing Exercises for Science and Technology Classrooms
Writing strategies in science and technology classes improve students’ ability to summarize information and make connections between new information and experiences.
When students write, they are active learners. The process itself has been shown to increase students’ understanding of concepts and provides an outlet through which to make connections.
Writing in science and technology classrooms shows students that these subjects are not as much about the memorization of facts as the discovery of something new and how to communicate what has been found. This can be done through a variety of summative and exploratory exercises, including the process of learning how to write compare and contrast essay . Through these writing exercises, students learn to identify similarities and differences, develop a clear thesis statement, and organize their thoughts effectively.
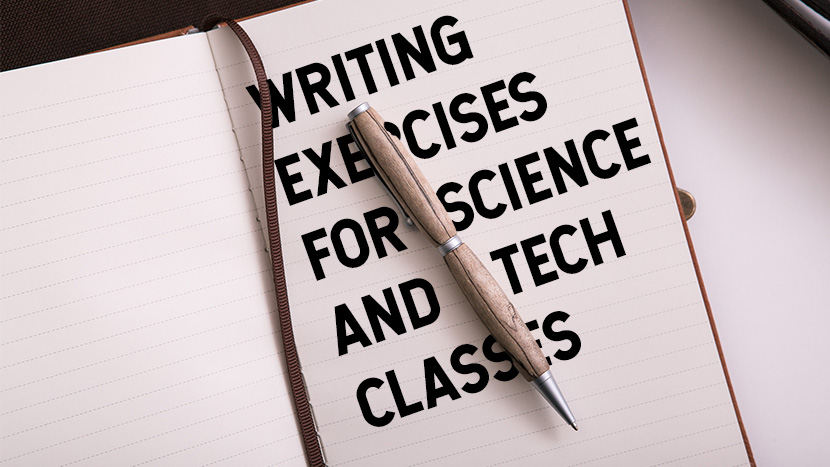
The Importance of Writing in Science and Technology
In science and technology classrooms, writing serves many purposes. It allows students to document their observations, experiment results, and research findings. This documentation forms a crucial part of the scientific process, helping students to reflect on their work and communicate their ideas effectively.
Moreover, writing helps students to synthesize information, organize their thoughts, and make connections between different concepts. For instance, when students write about a technology or scientific concept, they must first understand it thoroughly. They then need to break it down into its constituent parts, explain these in easy-to-understand language, and show how they connect. This process enhances their comprehension and retention of the material.
Summary Exercises
Writing assignments that summarize information take a variety of forms in science and technology classrooms. Lab reports are commonly utilized to not only collect student data and provide context for the scientific method but also provide a medium through which students analyze what they observe and provide concluding thoughts. Paragraphs or essays can be assigned to evaluate a scientific article. More advanced students could send a letter to the editor of a scientific or technical journal.
Other student-centered summative exercises include shorter writing pieces. Students can write a sentence or two to answer a question about a homework assignment. They can write an explanation to go with a graph, diagram, or model. True or false questions on an assessment can also become a writing practice when students are asked to provide a rewording of a false statement so that it becomes true.
Exploratory Exercises
Though not as precise as summative exercises, exploratory writing has an important place in science and technology classrooms. These exercises are less formal and encourage students to make connections between known concepts or explore new ideas by using what they already know.
One way to incorporate writing in this informal manner is to utilize brainstorming. Brainstorming allows students to think about what they already know and how it might be related to the problem or question posed. You could get students to brainstorm ideas and put it into an AI for essay writing then analyze what they think turned out well, and what they would do better if they had written it themselves.
Journal Assignments
Another informal writing exercise that can promote critical thinking and help develop new connections with new material is through the use of a journal. Journal assignments can take many forms, from requiring a specified number of entries, to having the student’s choose how often they write each week, or even providing specific questions or statements for students to contemplate through their writing.
Research Papers
Writing a research paper requires students to delve deep into a specific topic within science or technology. This process necessitates a comprehensive understanding of the subject. Students must read widely, analyze existing literature, and synthesize information from various sources. In doing so, they gain a more profound, layered understanding of the topic at hand.
Research papers promote independent learning. Rather than relying solely on classroom instruction, students must take the initiative to seek out information, understand complex concepts, and draw their own conclusions. This fosters a sense of responsibility for their learning and prepares them for future academic and professional pursuits where self-directed learning is key.
Reflection Essays
Reflection essays require students to think deeply about what they have learned, understand the concepts at a deeper level, and articulate their thoughts. By reflecting on a particular lesson, experiment , or project, students have the chance to consolidate their understanding, identify areas of confusion, and determine strategies to improve their learning.
Reflection essays can help bridge the gap between theory and practice. After experimenting, for instance, students can reflect on the results and how they relate to the theoretical concepts they’ve studied. This process helps them see the relevance of what they’re learning and apply theoretical knowledge to practical situations.
Technical Documentation
Writing technical documents requires students to have a comprehensive understanding of the subject. They need to break down complex ideas into simple, digestible pieces of information. This process reinforces their understanding and helps them view the topic from different perspectives.
Technical documentation also enhances students’ communication skills. It encourages them to write precisely and logically, making complex information understandable to non-experts. This skill is crucial in the science and technology fields, where professionals often need to communicate their work to diverse audiences.
Incorporating technical documentation in the classroom also prepares students for real-world scenarios. Whether they’re writing user manuals, product specifications, or technical reports, students get a taste of what’s expected in a professional setting. This experience can be invaluable when they step into the workforce.
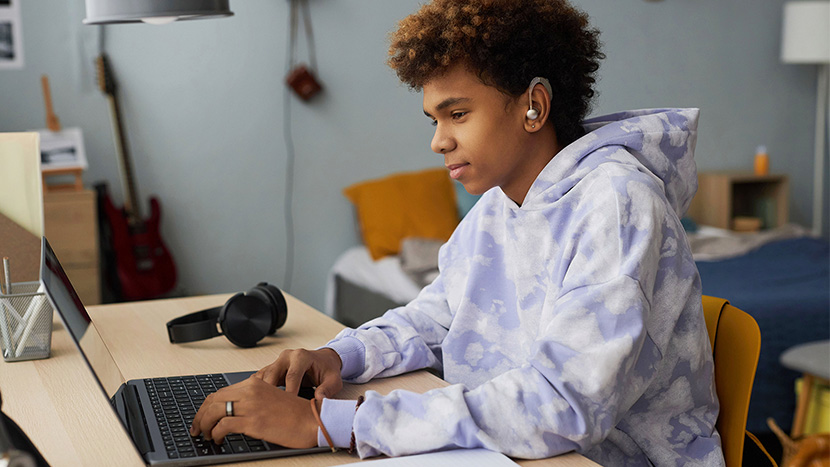
When writing for science or technology classes, students should aim to be logical and objective. Unless the writing is exploratory, it should also be quantitative and verifiable. Students should be encouraged to avoid the first person, be organized, and provide data or references whenever appropriate. Writing is introduced in this context to develop critical thinking skills while increasing their understanding of the concepts.
Choose one of the following categories to see related pages:
Share this page.
Austin is the principle web director for Untamed Science and Stone Age Man . He is also the web-director of the series for the High School biology, Middle Grades Science and Elementary Science content. When Austin isn't making amazing content for the web, he's out on his mountain bike or in a canoe.
Science Newsletter:
Full list of our videos.
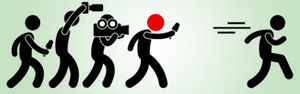
Teaching Biology?
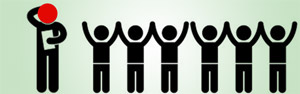
How to Make Science Films
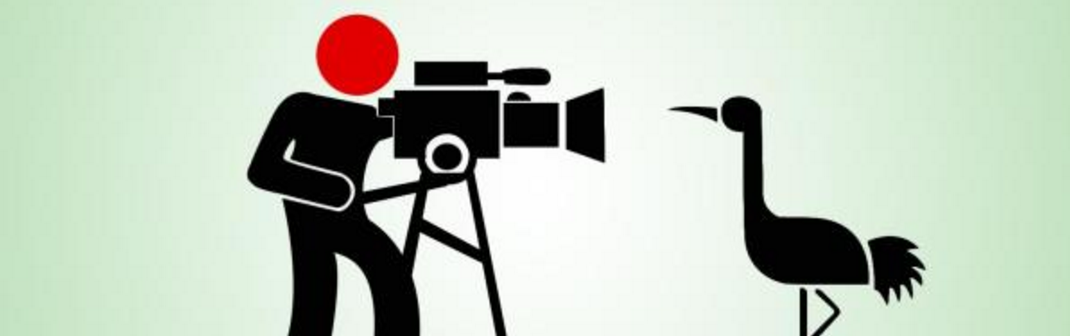
Read our Wildlife Guide
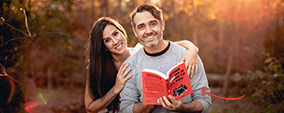
New From Untamed Science
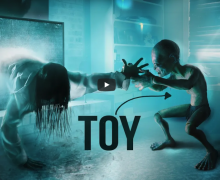

45,000+ students realised their study abroad dream with us. Take the first step today
Meet top uk universities from the comfort of your home, here’s your new year gift, one app for all your, study abroad needs, start your journey, track your progress, grow with the community and so much more.

Verification Code
An OTP has been sent to your registered mobile no. Please verify
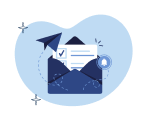
Thanks for your comment !
Our team will review it before it's shown to our readers.
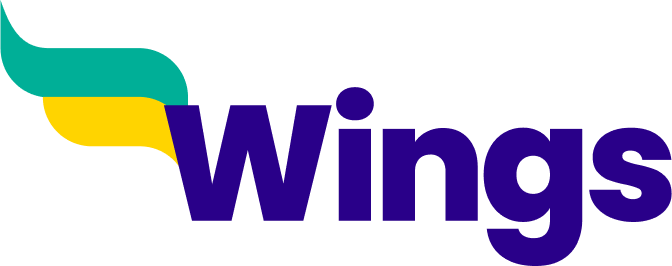
- School Education /
Essay on Science and Technology for Students: 100, 200, 350 Words
- Updated on
- Sep 20, 2023
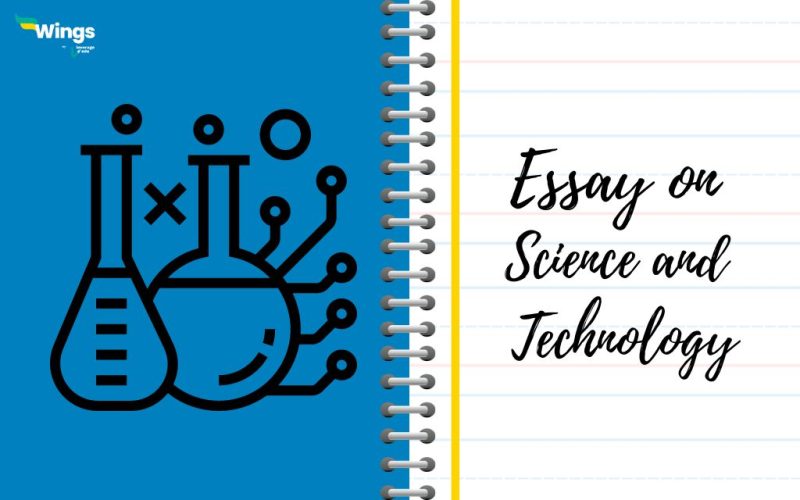
Writing an essay on science and technology requires you to keep yourself updated with the recent developments in this field. Science is a field which has no limits. It is the most potent of all the fields and when combined with technology, then even the sky doesn’t remain a limit. Science is everywhere from the minute microscopic organisms to the gigantic celestial bodies. It’s the very essence of our existence. Let’s learn about Science and Technology in an essay format.

Also Read – Essay on Corruption
Essay on Science and Technology in 100 Words
Everything we do, every breath we take, every move we make, every interaction with any object, and even the thoughts we have, and the dreams we see, all involve science. Similarly, as the world is progressing, technology is getting intertwined with even the basic aspects of our lives. Be it education, sports, entertainment, talking to our loved ones, etc. Everything is inclusive of Technology nowadays. It is safe to say that Science and Technology go hand-in-hand. They are mutually inclusive of each other. Although from a broader perspective, Technology is a branch of Science, but still, each of these fields cannot be sustained without the other.
Essay on Science and Technology in 200 Words
Science and Technology are important aspects of life from the very beginning of the day to the end of it. We wake up in the morning because of the sound of our alarm clocks and go to bed at night after switching off our lights. Most importantly, it helps us save time is one of the results of advancements in science and technology. Each day new Technologies are being developed that are making human life easier and much more convenient.Advantages of Science and Technology
If we were to name the advantages of science and technology, then we would fall short of words because they are numerous. These range from the very little things to the very big ones.
Science and Technology are the fields that have enabled man to look beyond our own planet and hence, discover new planets and much more. And the most recent of the Project of India, The successful landing of Chandrayaan-3 on the south pole of the moon proves that the potential of Science and Technology cannot be fathomed via any means. The potential it holds is immense.
In conclusion, we can confidently say that Science and Technology have led us to achieve an absolutely amazing life. However, it is extremely important to make use of the same in a judicious way so as to ensure its sustenance.
Also Read – Essay on Noise Pollution
Essay on Science and Technology in 350 Words
Science and Technology include everything, from the smallest of the microbes to the most complex of the mechanisms. Our world cannot exist without Science and Technology. It is hard to imagine our lives without science and technology now.
Impact of Science & Technology
The impact of science and technology is so massive that it incorporates almost each and every field of science and even others. The cures to various diseases are being made due to the advancement in Science and Technology only. Also, technology has enhanced the production of crops and other agricultural practices also rely on Science and Technology for their own advancement. All of the luxuries that we have on a day-to-day basis in our lives are because of Science and Technology. Subsequently, the fields of Science and Technology have also assisted in the development of other fields as well such as, Mathematics , Astrophysics , Nuclear Energy , etc. Hence, we can say that we live in the era of Science and Technology.
Safety Measures
Although the field of Science and Technology has provided the world with innumerable advancements and benefits that are carrying the world forward, there are a lot of aspects of the same that have a negative impact too. The negative impact of these is primarily on nature and wildlife and hence, indirectly and directly on humans as well.
The large factories that are associated with manufacturing or other developmental processes release large amounts of waste which may or may not be toxic in nature. This waste gets deposited in nature and water bodies and causes pollution. The animals marine or terrestrial living in their respective ecosystems may even ingest plastic or other toxic waste and that leads to their death. There are a lot of other negative aspects of the same.
Hence, it becomes our responsibility to use Science and Technology judiciously and prevent the degradation of nature and wildlife so as to sustain our planet, along with all its ecosystems, which will eventually ensure our existence in a healthy ecosystem leading to healthy and long life.
Science is something that is limitless. It is the most potent of all the fields and when combined with technology, then even the sky doesn’t remain a limit. Science is everywhere from the minute microscopic organisms to the most gigantic ones. It’s the very essence of our existence.
Science and Technology are important aspects of life. All of the luxuries that we have on a day-to-day basis in our lives are because of Science and Technology. Most importantly, it helps us save time is one of the results of advancements in science and technology. It is hard to imagine our lives without science and technology now.
In any nation, science and technology holds a crucial part in its development in all aspect. The progress of the nation is dependent upon science and technology. It holds the to economic growth, changing the quality of life, and transformation of the society.
We hope this blog of ours on Essay on Science and Technology has helped you gain a deeper knowledge of the same. For more such informative and educational essays please visit our site:- Leverage Edu Essay Writing .
Deepansh Gautam
Leave a Reply Cancel reply
Save my name, email, and website in this browser for the next time I comment.
Contact no. *

Connect With Us
45,000+ students realised their study abroad dream with us. take the first step today..

Resend OTP in

Need help with?
Study abroad.
UK, Canada, US & More
IELTS, GRE, GMAT & More
Scholarship, Loans & Forex
Country Preference
New Zealand
Which English test are you planning to take?
Which academic test are you planning to take.
Not Sure yet

When are you planning to take the exam?
Already booked my exam slot
Within 2 Months
Want to learn about the test
Which Degree do you wish to pursue?
When do you want to start studying abroad.
January 2024
September 2024
What is your budget to study abroad?
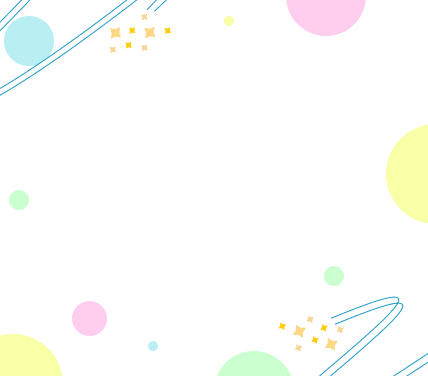
How would you describe this article ?
Please rate this article
We would like to hear more.
Have something on your mind?

Make your study abroad dream a reality in January 2022 with

India's Biggest Virtual University Fair

Essex Direct Admission Day
Why attend .

Don't Miss Out
Organizing Your Social Sciences Research Assignments
- Annotated Bibliography
- Analyzing a Scholarly Journal Article
- Group Presentations
- Dealing with Nervousness
- Using Visual Aids
- Grading Someone Else's Paper
- Types of Structured Group Activities
- Group Project Survival Skills
- Leading a Class Discussion
- Multiple Book Review Essay
- Reviewing Collected Works
- Writing a Case Analysis Paper
- Writing a Case Study
- About Informed Consent
- Writing Field Notes
- Writing a Policy Memo
- Writing a Reflective Paper
- Writing a Research Proposal
- Generative AI and Writing
- Acknowledgments
Reflective writing is a process of identifying, questioning, and critically evaluating course-based learning opportunities, integrated with your own observations, experiences, impressions, beliefs, assumptions, or biases, and which describes how this process stimulated new or creative understanding about the content of the course.
A reflective paper describes and explains in an introspective, first person narrative, your reactions and feelings about either a specific element of the class [e.g., a required reading; a film shown in class] or more generally how you experienced learning throughout the course. Reflective writing assignments can be in the form of a single paper, essays, portfolios, journals, diaries, or blogs. In some cases, your professor may include a reflective writing assignment as a way to obtain student feedback that helps improve the course, either in the moment or for when the class is taught again.
How to Write a Reflection Paper . Academic Skills, Trent University; Writing a Reflection Paper . Writing Center, Lewis University; Critical Reflection . Writing and Communication Centre, University of Waterloo; Tsingos-Lucas et al. "Using Reflective Writing as a Predictor of Academic Success in Different Assessment Formats." American Journal of Pharmaceutical Education 81 (2017): Article 8.
Benefits of Reflective Writing Assignments
As the term implies, a reflective paper involves looking inward at oneself in contemplating and bringing meaning to the relationship between course content and the acquisition of new knowledge . Educational research [Bolton, 2010; Ryan, 2011; Tsingos-Lucas et al., 2017] demonstrates that assigning reflective writing tasks enhances learning because it challenges students to confront their own assumptions, biases, and belief systems around what is being taught in class and, in so doing, stimulate student’s decisions, actions, attitudes, and understanding about themselves as learners and in relation to having mastery over their learning. Reflection assignments are also an opportunity to write in a first person narrative about elements of the course, such as the required readings, separate from the exegetic and analytical prose of academic research papers.
Reflection writing often serves multiple purposes simultaneously. In no particular order, here are some of reasons why professors assign reflection papers:
- Enhances learning from previous knowledge and experience in order to improve future decision-making and reasoning in practice . Reflective writing in the applied social sciences enhances decision-making skills and academic performance in ways that can inform professional practice. The act of reflective writing creates self-awareness and understanding of others. This is particularly important in clinical and service-oriented professional settings.
- Allows students to make sense of classroom content and overall learning experiences in relation to oneself, others, and the conditions that shaped the content and classroom experiences . Reflective writing places you within the course content in ways that can deepen your understanding of the material. Because reflective thinking can help reveal hidden biases, it can help you critically interrogate moments when you do not like or agree with discussions, readings, or other aspects of the course.
- Increases awareness of one’s cognitive abilities and the evidence for these attributes . Reflective writing can break down personal doubts about yourself as a learner and highlight specific abilities that may have been hidden or suppressed due to prior assumptions about the strength of your academic abilities [e.g., reading comprehension; problem-solving skills]. Reflective writing, therefore, can have a positive affective [i.e., emotional] impact on your sense of self-worth.
- Applying theoretical knowledge and frameworks to real experiences . Reflective writing can help build a bridge of relevancy between theoretical knowledge and the real world. In so doing, this form of writing can lead to a better understanding of underlying theories and their analytical properties applied to professional practice.
- Reveals shortcomings that the reader will identify . Evidence suggests that reflective writing can uncover your own shortcomings as a learner, thereby, creating opportunities to anticipate the responses of your professor may have about the quality of your coursework. This can be particularly productive if the reflective paper is written before final submission of an assignment.
- Helps students identify their tacit [a.k.a., implicit] knowledge and possible gaps in that knowledge . Tacit knowledge refers to ways of knowing rooted in lived experience, insight, and intuition rather than formal, codified, categorical, or explicit knowledge. In so doing, reflective writing can stimulate students to question their beliefs about a research problem or an element of the course content beyond positivist modes of understanding and representation.
- Encourages students to actively monitor their learning processes over a period of time . On-going reflective writing in journals or blogs, for example, can help you maintain or adapt learning strategies in other contexts. The regular, purposeful act of reflection can facilitate continuous deep thinking about the course content as it evolves and changes throughout the term. This, in turn, can increase your overall confidence as a learner.
- Relates a student’s personal experience to a wider perspective . Reflection papers can help you see the big picture associated with the content of a course by forcing you to think about the connections between scholarly content and your lived experiences outside of school. It can provide a macro-level understanding of one’s own experiences in relation to the specifics of what is being taught.
- If reflective writing is shared, students can exchange stories about their learning experiences, thereby, creating an opportunity to reevaluate their original assumptions or perspectives . In most cases, reflective writing is only viewed by your professor in order to ensure candid feedback from students. However, occasionally, reflective writing is shared and openly discussed in class. During these discussions, new or different perspectives and alternative approaches to solving problems can be generated that would otherwise be hidden. Sharing student's reflections can also reveal collective patterns of thought and emotions about a particular element of the course.
Bolton, Gillie. Reflective Practice: Writing and Professional Development . London: Sage, 2010; Chang, Bo. "Reflection in Learning." Online Learning 23 (2019), 95-110; Cavilla, Derek. "The Effects of Student Reflection on Academic Performance and Motivation." Sage Open 7 (July-September 2017): 1–13; Culbert, Patrick. “Better Teaching? You Can Write On It “ Liberal Education (February 2022); McCabe, Gavin and Tobias Thejll-Madsen. The Reflection Toolkit . University of Edinburgh; The Purpose of Reflection . Introductory Composition at Purdue University; Practice-based and Reflective Learning . Study Advice Study Guides, University of Reading; Ryan, Mary. "Improving Reflective Writing in Higher Education: A Social Semiotic Perspective." Teaching in Higher Education 16 (2011): 99-111; Tsingos-Lucas et al. "Using Reflective Writing as a Predictor of Academic Success in Different Assessment Formats." American Journal of Pharmaceutical Education 81 (2017): Article 8; What Benefits Might Reflective Writing Have for My Students? Writing Across the Curriculum Clearinghouse; Rykkje, Linda. "The Tacit Care Knowledge in Reflective Writing: A Practical Wisdom." International Practice Development Journal 7 (September 2017): Article 5; Using Reflective Writing to Deepen Student Learning . Center for Writing, University of Minnesota.
How to Approach Writing a Reflection Paper
Thinking About Reflective Thinking
Educational theorists have developed numerous models of reflective thinking that your professor may use to frame a reflective writing assignment. These models can help you systematically interpret your learning experiences, thereby ensuring that you ask the right questions and have a clear understanding of what should be covered. A model can also represent the overall structure of a reflective paper. Each model establishes a different approach to reflection and will require you to think about your writing differently. If you are unclear how to fit your writing within a particular reflective model, seek clarification from your professor. There are generally two types of reflective writing assignments, each approached in slightly different ways.
1. Reflective Thinking about Course Readings
This type of reflective writing focuses on thoughtfully thinking about the course readings that underpin how most students acquire new knowledge and understanding about the subject of a course. Reflecting on course readings is often assigned in freshmen-level, interdisciplinary courses where the required readings examine topics viewed from multiple perspectives and, as such, provide different ways of analyzing a topic, issue, event, or phenomenon. The purpose of reflective thinking about course readings in the social and behavioral sciences is to elicit your opinions, beliefs, and feelings about the research and its significance. This type of writing can provide an opportunity to break down key assumptions you may have and, in so doing, reveal potential biases in how you interpret the scholarship.
If you are assigned to reflect on course readings, consider the following methods of analysis as prompts that can help you get started :
- Examine carefully the main introductory elements of the reading, including the purpose of the study, the theoretical framework being used to test assumptions, and the research questions being addressed. Think about what ideas stood out to you. Why did they? Were these ideas new to you or familiar in some way based on your own lived experiences or prior knowledge?
- Develop your ideas around the readings by asking yourself, what do I know about this topic? Where does my existing knowledge about this topic come from? What are the observations or experiences in my life that influence my understanding of the topic? Do I agree or disagree with the main arguments, recommended course of actions, or conclusions made by the author(s)? Why do I feel this way and what is the basis of these feelings?
- Make connections between the text and your own beliefs, opinions, or feelings by considering questions like, how do the readings reinforce my existing ideas or assumptions? How the readings challenge these ideas or assumptions? How does this text help me to better understand this topic or research in ways that motivate me to learn more about this area of study?
2. Reflective Thinking about Course Experiences
This type of reflective writing asks you to critically reflect on locating yourself at the conceptual intersection of theory and practice. The purpose of experiential reflection is to evaluate theories or disciplinary-based analytical models based on your introspective assessment of the relationship between hypothetical thinking and practical reality; it offers a way to consider how your own knowledge and skills fit within professional practice. This type of writing also provides an opportunity to evaluate your decisions and actions, as well as how you managed your subsequent successes and failures, within a specific theoretical framework. As a result, abstract concepts can crystallize and become more relevant to you when considered within your own experiences. This can help you formulate plans for self-improvement as you learn.
If you are assigned to reflect on your experiences, consider the following questions as prompts to help you get started :
- Contextualize your reflection in relation to the overarching purpose of the course by asking yourself, what did you hope to learn from this course? What were the learning objectives for the course and how did I fit within each of them? How did these goals relate to the main themes or concepts of the course?
- Analyze how you experienced the course by asking yourself, what did I learn from this experience? What did I learn about myself? About working in this area of research and study? About how the course relates to my place in society? What assumptions about the course were supported or refuted?
- Think introspectively about the ways you experienced learning during the course by asking yourself, did your learning experiences align with the goals or concepts of the course? Why or why do you not feel this way? What was successful and why do you believe this? What would you do differently and why is this important? How will you prepare for a future experience in this area of study?
NOTE: If you are assigned to write a journal or other type of on-going reflection exercise, a helpful approach is to reflect on your reflections by re-reading what you have already written. In other words, review your previous entries as a way to contextualize your feelings, opinions, or beliefs regarding your overall learning experiences. Over time, this can also help reveal hidden patterns or themes related to how you processed your learning experiences. Consider concluding your reflective journal with a summary of how you felt about your learning experiences at critical junctures throughout the course, then use these to write about how you grew as a student learner and how the act of reflecting helped you gain new understanding about the subject of the course and its content.
ANOTHER NOTE: Regardless of whether you write a reflection paper or a journal, do not focus your writing on the past. The act of reflection is intended to think introspectively about previous learning experiences. However, reflective thinking should document the ways in which you progressed in obtaining new insights and understandings about your growth as a learner that can be carried forward in subsequent coursework or in future professional practice. Your writing should reflect a furtherance of increasing personal autonomy and confidence gained from understanding more about yourself as a learner.
Structure and Writing Style
There are no strict academic rules for writing a reflective paper. Reflective writing may be assigned in any class taught in the social and behavioral sciences and, therefore, requirements for the assignment can vary depending on disciplinary-based models of inquiry and learning. The organization of content can also depend on what your professor wants you to write about or based on the type of reflective model used to frame the writing assignment. Despite these possible variations, below is a basic approach to organizing and writing a good reflective paper, followed by a list of problems to avoid.
Pre-flection
In most cases, it's helpful to begin by thinking about your learning experiences and outline what you want to focus on before you begin to write the paper. This can help you organize your thoughts around what was most important to you and what experiences [good or bad] had the most impact on your learning. As described by the University of Waterloo Writing and Communication Centre, preparing to write a reflective paper involves a process of self-analysis that can help organize your thoughts around significant moments of in-class knowledge discovery.
- Using a thesis statement as a guide, note what experiences or course content stood out to you , then place these within the context of your observations, reactions, feelings, and opinions. This will help you develop a rough outline of key moments during the course that reflect your growth as a learner. To identify these moments, pose these questions to yourself: What happened? What was my reaction? What were my expectations and how were they different from what transpired? What did I learn?
- Critically think about your learning experiences and the course content . This will help you develop a deeper, more nuanced understanding about why these moments were significant or relevant to you. Use the ideas you formulated during the first stage of reflecting to help you think through these moments from both an academic and personal perspective. From an academic perspective, contemplate how the experience enhanced your understanding of a concept, theory, or skill. Ask yourself, did the experience confirm my previous understanding or challenge it in some way. As a result, did this highlight strengths or gaps in your current knowledge? From a personal perspective, think introspectively about why these experiences mattered, if previous expectations or assumptions were confirmed or refuted, and if this surprised, confused, or unnerved you in some way.
- Analyze how these experiences and your reactions to them will shape your future thinking and behavior . Reflection implies looking back, but the most important act of reflective writing is considering how beliefs, assumptions, opinions, and feelings were transformed in ways that better prepare you as a learner in the future. Note how this reflective analysis can lead to actions you will take as a result of your experiences, what you will do differently, and how you will apply what you learned in other courses or in professional practice.
Basic Structure and Writing Style
Reflective Background and Context
The first part of your reflection paper should briefly provide background and context in relation to the content or experiences that stood out to you. Highlight the settings, summarize the key readings, or narrate the experiences in relation to the course objectives. Provide background that sets the stage for your reflection. You do not need to go into great detail, but you should provide enough information for the reader to understand what sources of learning you are writing about [e.g., course readings, field experience, guest lecture, class discussions] and why they were important. This section should end with an explanatory thesis statement that expresses the central ideas of your paper and what you want the readers to know, believe, or understand after they finish reading your paper.
Reflective Interpretation
Drawing from your reflective analysis, this is where you can be personal, critical, and creative in expressing how you felt about the course content and learning experiences and how they influenced or altered your feelings, beliefs, assumptions, or biases about the subject of the course. This section is also where you explore the meaning of these experiences in the context of the course and how you gained an awareness of the connections between these moments and your own prior knowledge.
Guided by your thesis statement, a helpful approach is to interpret your learning throughout the course with a series of specific examples drawn from the course content and your learning experiences. These examples should be arranged in sequential order that illustrate your growth as a learner. Reflecting on each example can be done by: 1) introducing a theme or moment that was meaningful to you, 2) describing your previous position about the learning moment and what you thought about it, 3) explaining how your perspective was challenged and/or changed and why, and 4) introspectively stating your current or new feelings, opinions, or beliefs about that experience in class.
It is important to include specific examples drawn from the course and placed within the context of your assumptions, thoughts, opinions, and feelings. A reflective narrative without specific examples does not provide an effective way for the reader to understand the relationship between the course content and how you grew as a learner.
Reflective Conclusions
The conclusion of your reflective paper should provide a summary of your thoughts, feelings, or opinions regarding what you learned about yourself as a result of taking the course. Here are several ways you can frame your conclusions based on the examples you interpreted and reflected on what they meant to you. Each example would need to be tied to the basic theme [thesis statement] of your reflective background section.
- Your reflective conclusions can be described in relation to any expectations you had before taking the class [e.g., “I expected the readings to not be relevant to my own experiences growing up in a rural community, but the research actually helped me see that the challenges of developing my identity as a child of immigrants was not that unusual...”].
- Your reflective conclusions can explain how what you learned about yourself will change your actions in the future [e.g., “During a discussion in class about the challenges of helping homeless people, I realized that many of these people hate living on the street but lack the ability to see a way out. This made me realize that I wanted to take more classes in psychology...”].
- Your reflective conclusions can describe major insights you experienced a critical junctures during the course and how these moments enhanced how you see yourself as a student learner [e.g., "The guest speaker from the Head Start program made me realize why I wanted to pursue a career in elementary education..."].
- Your reflective conclusions can reconfigure or reframe how you will approach professional practice and your understanding of your future career aspirations [e.g.,, "The course changed my perceptions about seeking a career in business finance because it made me realize I want to be more engaged in customer service..."]
- Your reflective conclusions can explore any learning you derived from the act of reflecting itself [e.g., “Reflecting on the course readings that described how minority students perceive campus activities helped me identify my own biases about the benefits of those activities in acclimating to campus life...”].
NOTE: The length of a reflective paper in the social sciences is usually less than a traditional research paper. However, don’t assume that writing a reflective paper is easier than writing a research paper. A well-conceived critical reflection paper often requires as much time and effort as a research paper because you must purposeful engage in thinking about your learning in ways that you may not be comfortable with or used to. This is particular true while preparing to write because reflective papers are not as structured as a traditional research paper and, therefore, you have to think deliberately about how you want to organize the paper and what elements of the course you want to reflect upon.
ANOTHER NOTE: Do not limit yourself to using only text in reflecting on your learning. If you believe it would be helpful, consider using creative modes of thought or expression such as, illustrations, photographs, or material objects that reflects an experience related to the subject of the course that was important to you [e.g., like a ticket stub to a renowned speaker on campus]. Whatever non-textual element you include, be sure to describe the object's relevance to your personal relationship to the course content.
Problems to Avoid
A reflective paper is not a “mind dump” . Reflective papers document your personal and emotional experiences and, therefore, they do not conform to rigid structures, or schema, to organize information. However, the paper should not be a disjointed, stream-of-consciousness narrative. Reflective papers are still academic pieces of writing that require organized thought, that use academic language and tone , and that apply intellectually-driven critical thinking to the course content and your learning experiences and their significance.
A reflective paper is not a research paper . If you are asked to reflect on a course reading, the reflection will obviously include some description of the research. However, the goal of reflective writing is not to present extraneous ideas to the reader or to "educate" them about the course. The goal is to share a story about your relationship with the learning objectives of the course. Therefore, unlike research papers, you are expected to write from a first person point of view which includes an introspective examination of your own opinions, feelings, and personal assumptions.
A reflection paper is not a book review . Descriptions of the course readings using your own words is not a reflective paper. Reflective writing should focus on how you understood the implications of and were challenged by the course in relation to your own lived experiences or personal assumptions, combined with explanations of how you grew as a student learner based on this internal dialogue. Remember that you are the central object of the paper, not the research materials.
A reflective paper is not an all-inclusive meditation. Do not try to cover everything. The scope of your paper should be well-defined and limited to your specific opinions, feelings, and beliefs about what you determine to be the most significant content of the course and in relation to the learning that took place. Reflections should be detailed enough to covey what you think is important, but your thoughts should be expressed concisely and coherently [as is true for any academic writing assignment].
Critical Reflection . Writing and Communication Centre, University of Waterloo; Critical Reflection: Journals, Opinions, & Reactions . University Writing Center, Texas A&M University; Connor-Greene, Patricia A. “Making Connections: Evaluating the Effectiveness of Journal Writing in Enhancing Student Learning.” Teaching of Psychology 27 (2000): 44-46; Good vs. Bad Reflection Papers , Franklin University; Dyment, Janet E. and Timothy S. O’Connell. "The Quality of Reflection in Student Journals: A Review of Limiting and Enabling Factors." Innovative Higher Education 35 (2010): 233-244: How to Write a Reflection Paper . Academic Skills, Trent University; Amelia TaraJane House. Reflection Paper . Cordia Harrington Center for Excellence, University of Arkansas; Ramlal, Alana, and Désirée S. Augustin. “Engaging Students in Reflective Writing: An Action Research Project.” Educational Action Research 28 (2020): 518-533; Writing a Reflection Paper . Writing Center, Lewis University; McGuire, Lisa, Kathy Lay, and Jon Peters. “Pedagogy of Reflective Writing in Professional Education.” Journal of the Scholarship of Teaching and Learning (2009): 93-107; Critical Reflection . Writing and Communication Centre, University of Waterloo; How Do I Write Reflectively? Academic Skills Toolkit, University of New South Wales Sydney; Reflective Writing . Skills@Library. University of Leeds; Walling, Anne, Johanna Shapiro, and Terry Ast. “What Makes a Good Reflective Paper?” Family Medicine 45 (2013): 7-12; Williams, Kate, Mary Woolliams, and Jane Spiro. Reflective Writing . 2nd edition. London: Red Globe Press, 2020; Yeh, Hui-Chin, Shih-hsien Yang, Jo Shan Fu, and Yen-Chen Shih. “Developing College Students’ Critical Thinking through Reflective Writing.” Higher Education Research and Development (2022): 1-16.
Writing Tip
Focus on Reflecting, Not on Describing
Minimal time and effort should be spent describing the course content you are asked to reflect upon. The purpose of a reflection assignment is to introspectively contemplate your reactions to and feeling about an element of the course. D eflecting the focus away from your own feelings by concentrating on describing the course content can happen particularly if "talking about yourself" [i.e., reflecting] makes you uncomfortable or it is intimidating. However, the intent of reflective writing is to overcome these inhibitions so as to maximize the benefits of introspectively assessing your learning experiences. Keep in mind that, if it is relevant, your feelings of discomfort could be a part of how you critically reflect on any challenges you had during the course [e.g., you realize this discomfort inhibited your willingness to ask questions during class, it fed into your propensity to procrastinate, or it made it difficult participating in groups].
Writing a Reflection Paper . Writing Center, Lewis University; Reflection Paper . Cordia Harrington Center for Excellence, University of Arkansas.
Another Writing Tip
Helpful Videos about Reflective Writing
These two short videos succinctly describe how to approach a reflective writing assignment. They are produced by the Academic Skills department at the University of Melbourne and the Skills Team of the University of Hull, respectively.
- << Previous: Writing a Policy Memo
- Next: Writing a Research Proposal >>
- Last Updated: Jun 3, 2024 9:44 AM
- URL: https://libguides.usc.edu/writingguide/assignments
The relationship between technology and science: Some historical and philosophical reflections. Part I
- Published: June 1994
- Volume 4 , pages 123–153, ( 1994 )
Cite this article
- Paul L. Gardner 1
1493 Accesses
26 Citations
Explore all metrics
This essay offers a detailed review of the literature on the relationship between technology and science. It is in two parts. Part I begins by describing ‘science’ and ‘technology’, and the differences between them. It then discusses the commonly-held technology-as-applied-science (TAS) view; the origins of this view, the support for it, and the strong historical and philosophical challenges to it, beginning more than half a century ago, are explored. The development of the steam engine is then offered as a brief case study to illustrate that science-technology relations are more complex than implied by the TAS view. Part I concludes with a consideration of ontological arguments supporting the reverse view, namely that technology is often a necessary precursor to science.
Part II, to be published in a following issue, explores some of the consequences of the TAS view. One consequence is that it has generated a story-line in which scientific ideas are emphasised and other factors necessary for technological innovation have been down-played. Another consequence is that, even in cases where technology does apply scientific knowledge, the process of application is often considered obvious; the difficulties of translating ideas into artefacts may not be appreciated. The essay argues for the telling of a more complex story of science-technology relations, one which recognises their historical independence in the past, and their mutual, two-way interaction in many modern fields of endeavour. It concludes with a consideration of some economic and educational implications.
This is a preview of subscription content, log in via an institution to check access.
Access this article
Price includes VAT (Russian Federation)
Instant access to the full article PDF.
Rent this article via DeepDyve
Institutional subscriptions
Similar content being viewed by others
Philosophy of Science and Philosophy of Technology: One or Two Philosophies of One or Two Objects?
Science and technology: what they are and why their relation matters.
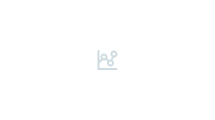
Heidegger and Husserl on the Technological-Scientific Worldview
Author information, authors and affiliations.
Centre for Science, Mathematics and Technology Education, Monash University, 3168, Clayton, Victoria, Australia
Paul L. Gardner
You can also search for this author in PubMed Google Scholar
Rights and permissions
Reprints and permissions
About this article
Gardner, P.L. The relationship between technology and science: Some historical and philosophical reflections. Part I. Int J Technol Des Educ 4 , 123–153 (1994). https://doi.org/10.1007/BF01204544
Download citation
Issue Date : June 1994
DOI : https://doi.org/10.1007/BF01204544
Share this article
Anyone you share the following link with will be able to read this content:
Sorry, a shareable link is not currently available for this article.
Provided by the Springer Nature SharedIt content-sharing initiative
- History and philosophy of science and technology
- science and technology education
- Find a journal
- Publish with us
- Track your research
Home / Essay Samples / Information Science and Technology / Impact of Technology / Understanding the Importance of Science, Technology and Society
Understanding the Importance of Science, Technology and Society
- Category: Information Science and Technology
- Topic: Advantages of Technology , Effects of Technology
Pages: 2 (934 words)
Views: 1800
- Downloads: -->
--> ⚠️ Remember: This essay was written and uploaded by an--> click here.
Found a great essay sample but want a unique one?
are ready to help you with your essay
You won’t be charged yet!
Digital Era Essays
Effects of Watching too much TV Essays
Bitcoin Essays
Artificial Intelligence Essays
Computer Graphics Essays
Related Essays
We are glad that you like it, but you cannot copy from our website. Just insert your email and this sample will be sent to you.
By clicking “Send”, you agree to our Terms of service and Privacy statement . We will occasionally send you account related emails.
Your essay sample has been sent.
In fact, there is a way to get an original essay! Turn to our writers and order a plagiarism-free paper.
samplius.com uses cookies to offer you the best service possible.By continuing we’ll assume you board with our cookie policy .--> -->